Jump to a key chapter
Introduction to Geotechnical Engineering
Geotechnical Engineering is a critical discipline within the field of civil engineering. It deals with the behavior of earth materials and helps in the design and construction of foundations, retaining walls, tunnels, and other structures.
What is Geotechnical Engineering?
The field of Geotechnical Engineering encompasses a wide range of activities. It includes the study of soil properties, slope stability, and the behavior of earth materials under different conditions. By understanding these factors, you can design structures that are safe, economical, and environmentally sound.
In essence, Geotechnical Engineering serves as the backbone for all infrastructure projects. Here are some key activities involved:
- Site investigation and soil sampling
- Geotechnical analysis and testing
- Foundation design
- Slope stability assessments
- Construction monitoring and supervision
Example: When designing a bridge, a geotechnical engineer will study the soil and rock beneath it to determine what type of foundation will be needed to support the structure, taking into account factors such as load, vibration, and potential erosion.
Geotechnical Engineering Definition
Geotechnical Engineering is the branch of civil engineering concerned with the engineering behavior of earth materials. It involves assessing and analyzing soil and rock properties to design and construct safe and effective foundations, retaining structures, and earthworks.
Deep Dive: Soil mechanics, a sub-discipline within geotechnical engineering, deals specifically with the behavior of soil layers. This includes understanding soil compaction, permeability, and the various methods used for soil stabilization. Geotechnical engineers often employ complex mathematical models to predict soil behavior under different stress conditions.
History and Evolution of Geotechnical Engineering
Geotechnical Engineering has a rich history that dates back to ancient civilizations. Early societies like the Egyptians and Romans used basic principles of soil mechanics to construct monumental structures, including pyramids and aqueducts. Over time, the field has evolved significantly thanks to scientific advancements and technological innovations.
In the 18th and 19th centuries, the development of classical soil mechanics theories by scientists such as Karl Terzaghi and Arthur Casagrande laid the foundation for modern Geotechnical Engineering. The introduction of laboratory testing techniques and analytical methods transformed the field and allowed for more precise and reliable designs.
Hint: Key historical figures in Geotechnical Engineering include Karl Terzaghi, often considered the father of soil mechanics, and Arthur Casagrande, known for his contributions to soil classification and compaction techniques.
Modern Geotechnical Engineering employs sophisticated tools, including computer simulations and advanced laboratory testing, to study complex soil-structure interactions. Today, geotechnical engineers play a vital role in the construction of skyscrapers, transportation systems, and environmental remediation projects.
With the growing emphasis on sustainability, current trends in Geotechnical Engineering focus on eco-friendly construction practices and the reuse of materials. Innovations such as geosynthetics and ground improvement techniques are regularly being incorporated to enhance both the performance and environmental impact of engineering projects.
Fundamentals of Geotechnical Engineering
Understanding the fundamentals of Geotechnical Engineering is crucial for developing safe and efficient infrastructure. This field examines the properties and behavior of earth materials and applies this knowledge to the engineering design of various structures.
Basic Geotechnical Engineering Principles
The basic principles of Geotechnical Engineering revolve around comprehending soil mechanics, rock mechanics, and the mechanics of other geological materials. Engineers analyze these properties to inform the design and construction of foundations, retaining walls, tunnels, and other structures.
Geotechnical principles include:
- Understanding soil properties such as density, permeability, and shear strength
- Assessing slope stability
- Analyzing soil-structure interaction
- Evaluating bearing capacity of soils
Bearing Capacity: The capacity of soil to support the loads applied to the ground. It is typically measured in terms of maximum allowable stress.
Example: When constructing a building, engineers perform a bearing capacity test to ensure the soil can support the weight of the structure. This involves calculating the stress distribution within the soil and comparing it to the soil's bearing capacity, typically using the formula \( q_{ult} = c N_c + \gamma D_f N_q + 0.5 \gamma B N_\gamma \) where \( q_{ult} \) is the ultimate bearing capacity, \( c \) is the cohesion, \( \gamma \) is the unit weight of soil, \( D_f \) is the depth of foundation, and \( B \) is the width of foundation.
Hint: Always ensure that the water table is considered in bearing capacity calculations, as it can significantly influence the stress distribution within the soil.
Common Geotechnical Engineering Materials
Different materials are used in geotechnical engineering, each having unique properties that affect their performance. The primary materials include:
- Soils
- Rocks
- Geosynthetics
Soil Types: There are various soil types, such as clay, silt, sand, and gravel. Each soil type has unique characteristics that impact its behavior and suitability for construction projects. For instance, clay particles are very small and cohesive, leading to high plasticity and lower permeability. Conversely, sand particles are larger and non-cohesive, offering greater drainage but less strength. The effective stress principle, formulated as \( \sigma' = \sigma - u \), where \( \sigma' \) is the effective stress, \( \sigma \) is the total stress, and \( u \) is the pore water pressure, helps in understanding how these soils behave under different conditions.
Rocks: Rocks are another significant material in geotechnical engineering. They are used in constructing foundations, tunnels, and other underground structures. The properties of rocks, such as compressive strength, permeability, and porosity, are crucial for determining their suitability in different applications.
Geosynthetics: These are synthetic products used to solve various geotechnical problems. Geosynthetics include geotextiles, geomembranes, and geogrids, and are used for soil reinforcement, drainage, filtration, and erosion control.
Role of Soil Mechanics in Geotechnical Engineering
Soil Mechanics plays a pivotal role in Geotechnical Engineering. It is the study of the physical properties and behaviors of soil as a construction material and under stress conditions. Engineers use soil mechanics to predict how soil will behave under different loads, which is crucial for the design and construction of foundations and earthworks. Key concepts in soil mechanics include:
- Effective Stress Principle
- Consolidation
- Shear Strength
Shear Strength: The maximum resistance of soil against shearing forces, which is calculated as \( \tau = c + \sigma tan \phi \). Here, \( \tau \) is the shear stress, \( c \) is the cohesion of soil, \( \sigma \) is the normal stress, and \( \phi \) is the angle of internal friction.
Example: During slope stability analysis, engineers use shear strength parameters to determine the safety factor. They typically calculate the factor of safety (FoS) using the formula \( FoS = \frac{Resisting Forces}{Driving Forces} \). If the FoS is greater than 1, the slope is considered stable.
Geotechnical Engineering Techniques
Geotechnical Engineering Techniques encompass a variety of methods used to analyze, enhance, and construct earth-related structures. These techniques inform critical aspects of civil engineering projects, ensuring that structures are both safe and stable.
Soil Testing Methods
Soil testing methods are fundamental in Geotechnical Engineering, as they help determine the physical properties of soil. These properties influence the design and safety of engineering projects.
Soil Testing: A series of laboratory and field tests conducted to assess the physical and mechanical properties of soil. These tests guide engineers in designing foundations and other structures.
Common soil testing methods include:
- Standard Penetration Test (SPT)
- Cone Penetration Test (CPT)
- Atterberg Limits Test
- Triaxial Shear Test
- Proctor Compaction Test
Example: In construction projects, the Standard Penetration Test (SPT) is commonly used to determine soil density and strength. During this test, a sample tube is driven into the ground using a weighted hammer, and the number of hammer blows required to drive the tube a specified distance is counted. This data helps engineers estimate the soil's bearing capacity.
Deep Dive: The Atterberg Limits Test classifies fine-grained soils based on their plasticity. It determines the plastic limit, liquid limit, and shrinkage limit of the soil. These values help in understanding soil behavior under different moisture conditions. Soil behavior changes significantly from a solid state to a plastic state, and eventually to a liquid state as moisture content increases. The liquid limit (LL) is the water content at which soil changes from a plastic to a liquid state, while the plastic limit (PL) is where soil transitions from a semi-solid to a plastic state. These limits are critical in predicting soil behavior under varying water conditions.
Ground Improvement Techniques
Ground improvement techniques are essential for enhancing the properties of soil to ensure the stability and integrity of structures. These techniques are applied to increase soil strength, reduce settlement, and improve drainage.
Common ground improvement techniques include:
- Compaction
- Grouting
- Soil Stabilization
- Geosynthetics
- Drainage Control
Soil Stabilization: A process used to improve the physical properties of soil by adding materials such as lime, cement, or fly ash. This enhances the soil's load-bearing capacity and stability.
Example: Compaction is a ground improvement technique where soil is densified using mechanical means. This reduces voids between soil particles, increases density, and improves soil strength. Compaction is commonly achieved using rollers, compactors, or tamping equipment. The level of compaction is often measured using the Proctor Compaction Test.
Hint: Geosynthetics, such as geotextiles and geogrids, play a significant role in ground improvement by reinforcing soil, aiding in drainage, and preventing erosion.
Foundation Engineering Basics
Foundation engineering is a crucial aspect of Geotechnical Engineering that ensures structures are anchored securely to the ground. It involves designing foundation systems that can safely transmit loads from the structure to the soil or rock below.
Foundation: A structural element that transfers the load from a building or structure to the ground, ensuring stability and strength.
Common foundation types include:
- Shallow Foundations
- Deep Foundations
- Pile Foundations
- Pier Foundations
- Mat Foundations
Example: Pile Foundations are used when the soil near the surface is not strong enough to support the load of the structure. Piles are long, slender columns made of concrete, steel, or wood that are driven deep into the ground to reach stronger soil or rock layers. This type of foundation is commonly used for bridges and high-rise buildings.
Deep Dive: Shallow Foundations are typically used for smaller structures where the load is relatively low, and the soil has sufficient strength near the surface. These include spread footings and slab-on-grade foundations. For spread footings, the load is distributed over a broad area of soil, while slab-on-grade foundations involve a concrete slab directly resting on the ground. These types of foundations are simple to construct and cost-effective but are not suitable for all soil conditions.
Applications of Geotechnical Engineering
Geotechnical Engineering has extensive applications in various sectors. Understanding the subsurface conditions is crucial for designing and constructing structures safely and effectively.
Geotechnical Considerations in Construction
When planning construction projects, geotechnical considerations are paramount to ensure stability and safety. This involves assessing soil properties, groundwater conditions, and potential geohazards.
Hint: Proper site investigation can help identify potential issues like soil liquefaction, which is critical for earthquake-prone areas.
Important geotechnical considerations include:
- Site Investigations: Conducting thorough geotechnical site investigations to understand soil and rock properties.
- Foundation Design: Designing foundations that can safely transfer building loads to the ground.
- Groundwater Control: Managing groundwater levels to prevent erosion and instability.
- Earthworks: Planning for soil excavation, compaction, and stabilization.
Example: In designing a high-rise building, a geotechnical engineer performs a detailed soil analysis to determine the type of foundation required. This could be a shallow foundation for firm soils or deep piles for weaker soils.
Deep Dive: Ground improvement techniques such as dynamic compaction, soil nailing, and grouting are often used to enhance soil properties at construction sites. For example, dynamic compaction involves dropping a heavy weight repeatedly on the ground to increase soil density. Soil nailing involves inserting steel rods into slopes to stabilize them, while grouting involves injecting a mixture into the soil to fill voids and strengthen the ground.
Case Studies in Geotechnical Engineering
Learning from real-world case studies helps you understand the practical applications and challenges of Geotechnical Engineering. These case studies showcase how geotechnical principles are applied to solve complex engineering problems.
Hint: Studying failed projects can provide valuable insights into what went wrong and how to avoid similar mistakes in future projects.
Notable geotechnical engineering case studies include:
- Millennium Tower, San Francisco: Examining settlement issues and the subsequent stabilization techniques used.
- Panama Canal Expansion: Detailing the extensive geotechnical investigations and challenges faced during the expansion project.
- Boston's Big Dig: Understanding the underground construction techniques and soil stabilization methods employed.
- Leaning Tower of Pisa: Analyzing the historical geotechnical challenges and the modern methods used for stabilization.
Example: The Panama Canal Expansion project faced geological challenges due to varying soil conditions. Through comprehensive geotechnical investigations, engineers implemented soil stabilization techniques like vibro-compaction and jet grouting to ensure the stability of new lock chambers.
Deep Dive: The Millennium Tower in San Francisco is a stark example of geotechnical engineering challenges. The tower experienced significant settlement post-construction due to inadequate foundation design, highlighting the importance of thorough geotechnical analysis. Engineers later used techniques like underpinning to stabilize the structure, showcasing advanced solutions to geotechnical issues.
Future Trends in Geotechnical Engineering
The field of Geotechnical Engineering is constantly evolving, with emerging trends and technologies shaping its future. Innovations in material science, data collection, and analytical methods are enhancing the way geotechnical challenges are addressed.
Hint: Emerging trends such as AI and machine learning are being leveraged to predict soil behavior and optimize engineering solutions.
Key future trends in Geotechnical Engineering include:
- Sustainable Practices: Focusing on environmentally friendly construction methods and materials to minimize environmental impact.
- Advanced Monitoring Systems: Using sensors and IoT devices to monitor soil conditions and structure health in real-time.
- Geotechnical Software: Employing sophisticated software for better analysis, simulation, and design of geotechnical projects.
- New Materials: Exploring innovative materials like geopolymers for soil stabilization and reinforcement.
Example: Advanced monitoring systems using sensors embedded in soil and structures are becoming more prevalent. These systems provide real-time data on soil settlement, moisture content, and structural load, enabling proactive maintenance and prevention of potential failures.
Deep Dive: Sustainable Geotechnical Engineering is an evolving trend that focuses on using eco-friendly construction practices and materials. Techniques such as using recycled materials for backfill, green retaining walls, and bio-engineered slopes are being adopted widely. Research is also ongoing in the development of geopolymers, which are eco-friendly alternatives to traditional concrete for soil stabilization. These materials have a lower carbon footprint and can be produced using industrial by-products, aligning with the principles of sustainable development.
Geotechnical Engineering - Key takeaways
- Geotechnical Engineering Definition: A branch of civil engineering focused on the behavior of earth materials, including soil and rock, to design safe and effective foundations, retaining structures, and other infrastructures.
- Fundamentals of Geotechnical Engineering: Understanding properties and behaviors of earth materials for the engineering design of structures, with principles including soil mechanics, rock mechanics, and soil-structure interaction.
- Soil Mechanics: A pivotal sub-discipline that studies the physical properties and behaviors of soil under stress conditions, including concepts like effective stress, consolidation, and shear strength.
- Geotechnical Engineering Techniques: Methods used for soil testing, ground improvement, and foundation engineering, ensuring safety and stability of structures.
- Historical Evolution: Modern Geotechnical Engineering has evolved from basic principles used by ancient civilizations, significantly advanced by figures such as Karl Terzaghi and Arthur Casagrande in the 18th and 19th centuries.
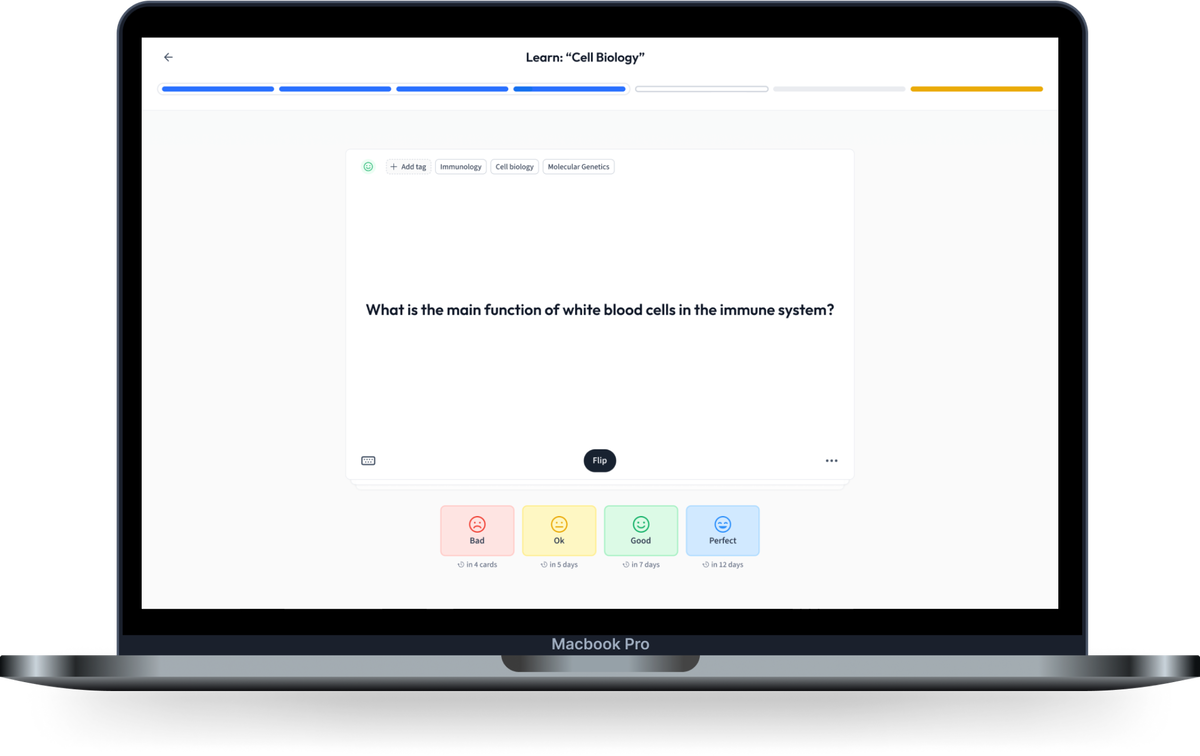
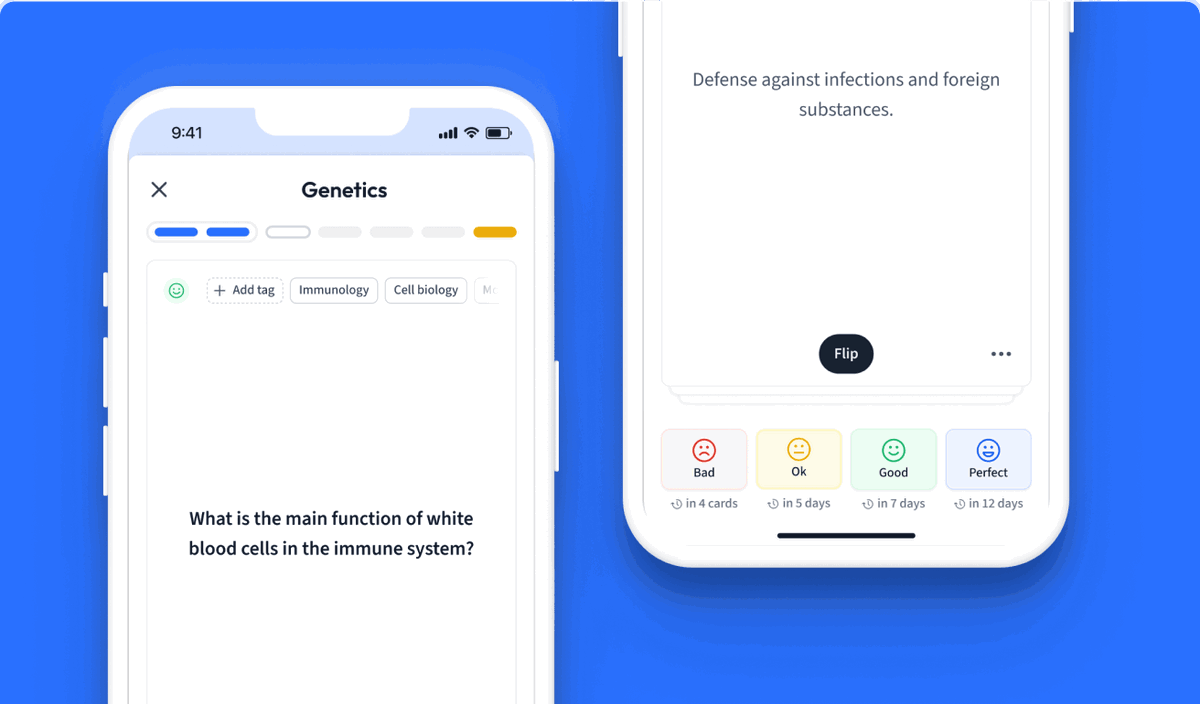
Learn with 12 Geotechnical Engineering flashcards in the free StudySmarter app
Already have an account? Log in
Frequently Asked Questions about Geotechnical Engineering
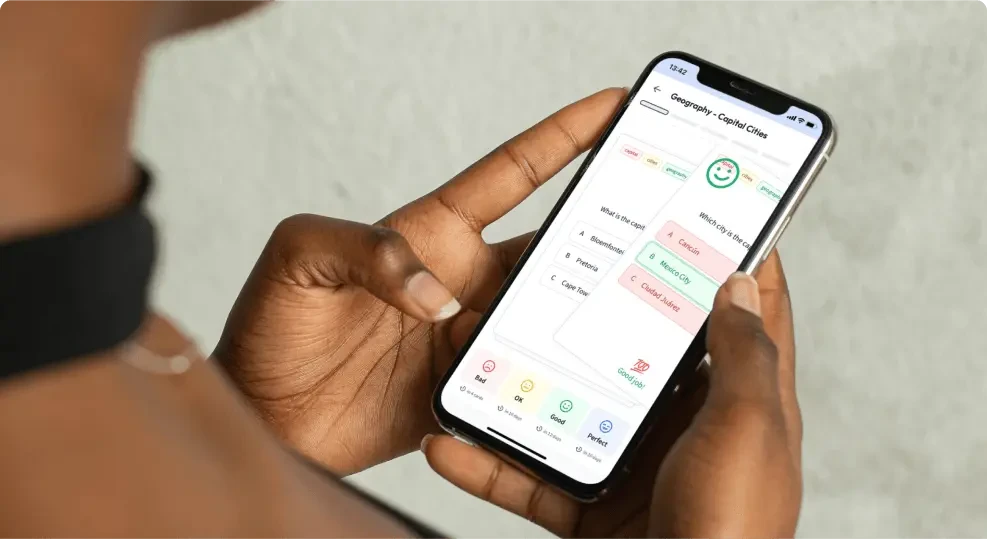
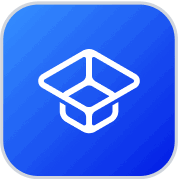
About StudySmarter
StudySmarter is a globally recognized educational technology company, offering a holistic learning platform designed for students of all ages and educational levels. Our platform provides learning support for a wide range of subjects, including STEM, Social Sciences, and Languages and also helps students to successfully master various tests and exams worldwide, such as GCSE, A Level, SAT, ACT, Abitur, and more. We offer an extensive library of learning materials, including interactive flashcards, comprehensive textbook solutions, and detailed explanations. The cutting-edge technology and tools we provide help students create their own learning materials. StudySmarter’s content is not only expert-verified but also regularly updated to ensure accuracy and relevance.
Learn more