Jump to a key chapter
The concept of an ecological footprint is central to understanding humanity's impact on the planet, particularly in architecture and urban planning.
An ecological footprint in architecture refers to the environmental impacts generated by constructing and operating buildings.
Ecological footprints measure how much land and water area a human population requires to produce the resources it consumes and to absorb its waste, including carbon emissions. In architecture, this concept is crucial as it reflects the relationship between buildings and the natural environment. An ecological footprint considers:
- The energy usage for heating, cooling, and lighting buildings
- The materials used in construction processes
- The waste produced by building activities
- The overall sustainability of building design and operations
Factors influencing the size of an ecological footprint in architecture include several key components.
Various factors contribute to the ecological footprint of a building. These include:
- Materials Used: Natural materials like wood, clay, and straw typically have lower footprints compared to synthetic materials like steel and concrete.
- Energy Efficiency: The design of the building plays a crucial role. More energy-efficient buildings have lower ecological footprints because they require less energy for heating, cooling, and lighting.
- Waste Management: Proper handling and reduction of waste during construction and demolition can significantly reduce a building's ecological footprint.
- Location: Buildings situated in areas with access to public transport can reduce the transport-related footprint by encouraging less vehicle use.
- Water Usage: Efficient water management systems reduce water consumption, thereby lowering the ecological footprint.
Ecological footprint in architecture is the measure of resource consumption and waste production by a building over its entire lifecycle, emphasizing sustainability.
Choosing materials with recycled content can further reduce a building's ecological footprint.
Urban planning significantly influences the ecological footprints by designing sustainable cities and communities.
The role of urban planning in managing ecological footprints cannot be overstated. Through urban planning, cities can be designed to minimize environmental impacts by:
- Promoting Public Transportation: Integrating efficient public transport systems encourages less reliance on personal vehicles, reducing carbon emissions and land usage.
- Encouraging High-Density Developments: High-density living reduces the per capita footprint by using resources more efficiently across shared spaces.
- Creating Green Spaces: Parks and green areas improve urban air quality and offer ecosystems for local wildlife while reducing the urban heat island effect.
- Implementing Regulations: Building codes and ordinances that mandate energy efficiency and sustainable practices are critical in reducing ecological footprints.
Incorporating sustainable practices goes beyond simply reducing the ecological footprint of individual structures or areas—it's about cultivating a holistic ecosystem approach. Cities should aim for a balance where homes, businesses, and public spaces coexist harmoniously with nature. This idea, often referred to as biophilic design, emphasizes the importance of connecting human environments with the natural world. Green roofs, vertical gardens, and community farms are some examples of biophilic elements that can be integrated into urban planning, significantly enhancing the wellbeing and ecological footprint of urban spaces. These initiatives not only mitigate environmental impacts but also foster a more cohesive and supportive community.
Sustainability in Architecture refers to designing buildings that minimize environmental impact while maximizing resource efficiency.
Understanding sustainability in architecture involves exploring principles and practices that ensure buildings are energy-efficient, resource-conscious, and environmentally friendly. The objective is to create structures that effectively occupy their space without causing harm to the planet. This approach benefits not only the environment but also the people who live and work in these buildings.
Key Concepts of Sustainability in Architecture include several important principles.
The key concepts of sustainability in architecture revolve around creating a balance between human activity and environmental preservation. Here are the primary concepts:
- Energy Efficiency: Incorporating technologies and designs that minimize energy use.
- Use of Sustainable Materials: Choosing materials that can be recycled or have a lower environmental impact.
- Water Conservation: Implementing water-saving techniques such as low-flow fixtures and rainwater harvesting.
- Indoor Environment Quality: Enhancing air and light quality within buildings to promote occupant health and productivity.
- Site Optimization: Careful selection of building sites to reduce environmental damage during construction and throughout the lifecycle of the building.
Solar panels and wind turbines can vastly reduce a building's reliance on non-renewable energy sources.
For instance, a building incorporating rooftop gardens can improve insulation and reduce heating and cooling demands, thus enhancing energy efficiency.
Benefits of Sustainable Design go beyond environmental conservation and include economic and social advantages.
The benefits of sustainable design are manifold. Implementing sustainable practices in architecture leads to:
- Cost Savings: Long-term reduction in energy and utility bills due to efficiency measures.
- Improved Health and Well-being: Natural lighting and ventilation systems improve air quality and comfort.
- Enhanced Market Value: Sustainability is becoming increasingly attractive to buyers and investors, boosting property values.
- Reduced Waste: By focusing on renewable resources and recyclable materials, less waste is generated during construction and use.
- Social Impact: Sustainable buildings contribute to community well-being with improved aesthetics and functionality.
Going beyond standard practices, sustainable design often includes advanced methodologies such as the integration of 'living walls' – vertical gardens that improve air quality and provide natural insulation. These walls not only showcase the innovative potential of green technology but also demonstrate how aesthetics and functionality can converge in architectural design.
Case Studies on Sustainability in Architecture demonstrate practical applications of sustainable practices.
Examining case studies provides valuable insights into how sustainable principles are applied in real-world situations. Notable examples include:
- The Bullitt Center in Seattle: Often referred to as the greenest commercial building in the world, it includes rainwater harvesting, composting toilets, and energy-efficient systems.
- BedZED (Beddington Zero Energy Development) in London: A community entirely powered by renewable energy, showcasing a wide array of sustainability principles.
- The Eden Project in Cornwall: With its modern biomes, it utilizes sustainable construction techniques and energy sources to create a unique educational environment.
Green Architecture Principles refer to designing buildings that prioritize sustainability and minimize environmental impact.
Green architecture is increasingly essential in modern construction as it seeks to harmonize human living spaces with the natural environment. This involves employing practices and materials that conserve energy and resources, reduce pollution and waste, and utilize environmentally friendly designs. Key green architecture principles include:
- Energy Efficiency: Designing structures that use less energy, such as implementing solar power systems.
- Water Conservation: Incorporating systems like rainwater harvesting to reduce water usage.
- Material Sustainability: Using materials that are renewable or have a minimal environmental impact.
- Indoor Environment Quality: Ensuring good ventilation and natural lighting to enhance occupant health.
Core Green Architecture Principles form the foundation of eco-friendly building practices.
The core principles that define green architecture involve several interrelated strategies designed to foster sustainability: Site Selection and Building Orientation: Choosing locations and orientations that take advantage of natural sunlight and wind patterns reduces energy needs. Resource Efficiency: This involves minimizing the use of water, energy, and materials by employing high-efficiency systems and sustainable building processes. Waste Reduction: Designing with a lifecycle perspective limits the waste generated by construction and demolition activities.Moreover, green roofs, which are living vegetation covers, help in managing urban heat and improving insulation.
An example of green architecture is the One Central Park in Sydney, which integrates hanging gardens, efficient water systems, and renewable energy sources to create a self-sustaining urban environment.
Implementing Green Architecture in Projects requires coordination between various stakeholders.
To effectively implement green architecture in projects, several steps need to be taken:
- Incorporate Experts Early: Engage sustainability consultants and environmental engineers during the planning phase.
- Use Eco-Friendly Materials: Select sustainable materials that have been sourced responsibly.
- Adopt Renewable Energy: Utilize solar panels, wind turbines, or geothermal energy systems.
- Monitor and Optimize Performance: Install systems to monitor energy and water use, and adjust for improvements over time.
Involving local communities in green architecture projects can ensure that the buildings meet both ecological needs and cultural expectations.
Green Architecture vs. Traditional Architecture explores distinctions in design, materials, and impact.
The differences between green and traditional architecture are stark in terms of both objectives and outcomes:
Green Architecture | Traditional Architecture |
Emphasizes sustainable resource use | Tends to utilize conventional construction methods |
Focuses on energy efficiency and renewable sources | Often relies on non-renewable energy |
Prioritizes occupant well-being with natural lighting and ventilation | May prioritize aesthetics and budget over environmental concerns |
Aims to reduce overall carbon footprint | May overlook long-term environmental impacts |
Digging deeper into the differences, green architecture reflects a holistic approach known as 'cradle to cradle' design. This framework posits that all materials and resources used in construction can be continuously reused in future projects, mimicking natural ecosystems where nothing goes to waste. Traditional architecture often follows a 'cradle to grave' approach, where materials are used once and then discarded. Understanding these frameworks is crucial for those striving to create truly sustainable built environments.
Carbon Footprint in Construction refers to the total greenhouse gas emissions caused directly and indirectly by construction processes.
In the construction sector, understanding the carbon footprint is essential. It encompasses the entire lifecycle of construction projects, from material extraction to demolition. Addressing carbon emissions in construction is important for reducing overall environmental impact. Understanding these emissions helps in crafting strategies to combat climate change and enhance sustainability.
Understanding Carbon Emissions in Construction involves analyzing various stages of building activities.
Construction carbon emissions primarily arise from:
- Material Production: The manufacture of materials like cement and steel is heavily carbon-intensive.
- Transportation: Emissions from moving materials and equipment to and from the building site add to the carbon footprint.
- On-site Construction Activities: Heavy machinery and equipment used during construction are significant contributors.
- Energy Consumption: The energy used in heating, cooling, and lighting buildings during operation contributes to emissions.
Choosing locally sourced materials can substantially decrease transportation emissions.
For example, using recycled steel instead of new steel can reduce carbon emissions by up to 80%, showcasing effective material management.
Strategies to Reduce Carbon Footprint in Construction focus on minimizing emissions throughout a building's lifecycle.
Reducing the carbon footprint in construction is pivotal for sustainable development. Effective strategies include:
- Utilizing Low-Carbon Materials: Switch to materials with lower embodied carbon, such as timber or recycled components.
- Energy-Efficient Designs: Optimize design for energy conservation through insulation and passive solar design.
- Adopting Green Building Technologies: Implement renewable energy technologies like solar panels and wind turbines.
- Efficient Waste Management: Minimize waste during and after construction via recycling and reusing materials.
A deeper examination into the realm of carbon reduction reveals the potential of carbon offsetting. This strategy allows construction firms to invest in environmental projects elsewhere, such as reforestation or renewable energy installations, to balance out their emissions. By purchasing carbon credits, companies can make strides toward a net-zero carbon status. The crux of this approach is that it promotes both reducing the initial emissions and contributing to wider sustainability goals.
Innovations Reducing Construction Carbon Footprint are essential for advancing sustainable building practices.
Recent technological advancements have paved the way for innovative solutions to curb carbon emissions in construction:
- 3D Printing: With precise material usage and reduced waste, 3D printed construction significantly lowers carbon output.
- Smart Building Technology: Automates building management systems to optimize energy use and reduce emissions.
- Green Concrete: Innovatively substitutes traditional cement to lower carbon dioxide emissions during production.
- Modular Construction: Prefabricated components reduce on-site activity and waste, leading to emission reduction.
Innovation | Impact on Emissions |
3D Printing | Decreases material waste |
Smart Technology | Optimizes energy consumption |
Green Concrete | Reduces CO2 during production |
Modular Construction | Minimizes onsite emissions |
Resource Consumption in Building involves a significant focus on the efficient use of materials, energy, and water to minimize environmental impacts and support sustainability.
Efficient Resource Management in Buildings requires strategic planning and implementation of sustainable practices.
Efficient resource management in buildings is essential to reduce the negative environmental impacts associated with construction and operation. This involves a comprehensive approach that includes:
- Energy Efficiency: Implementing energy-saving measures such as improved insulation, smart lighting systems, and high-efficiency HVAC systems.
- Water Conservation: Installing low-flow fixtures, and rainwater harvesting systems and reusing greywater for irrigation.
- Material Optimization: Careful selection and use of sustainable materials that are durable, low-impact, and recyclable.
Resource Management refers to the strategic allocation and use of resources, such as energy, water, and materials, to maximize efficiency and sustainability in building operations.
For example, the installation of solar panels on a building can substantially reduce reliance on fossil fuels, diminishing energy consumption and lowering greenhouse gas emissions.
Incorporating native plant species into landscaping can conserve water and support local biodiversity.
An emerging trend in resource management is the concept of 'net-zero' buildings. These structures are designed to produce as much energy as they consume through renewable sources, thereby reducing their reliance on external energy and minimizing their environmental impact. Achieving net-zero status involves a combination of rigorous energy-saving measures, on-site renewable energy generation, and sometimes, energy storage solutions. Such buildings not only contribute to environmental conservation but also offer cost savings over time through reduced utility expenses. This holistic approach to resource management sets a benchmark for sustainable building design, encouraging further innovation and application in the construction industry.
Impact of Resource Consumption on Ecological Footprints highlights the environmental consequences of resource use in buildings.
Resource consumption in buildings directly affects their ecological footprint, which measures how much biologically productive land and water area is needed to supply the resources a population consumes and to absorb associated waste. The impact is significant because:
- Energy Use: High energy consumption leads to increased carbon emissions and depletion of fossil fuels, exacerbating climate change.
- Water Usage: Excessive water consumption can lead to water shortages, affecting local ecosystems and communities.
- Waste Generation: Inefficient use of materials results in increased waste, contributing to landfill accumulation and environmental degradation.
Using energy-efficient appliances and renewable energy sources can significantly reduce a building's carbon footprint.
Sustainable Materials and Resource Consumption are crucial for reducing the environmental impact of buildings.
The selection of sustainable materials is a key aspect of minimizing the ecological footprint of buildings. These materials offer several benefits:
- Recyclability: Materials that can be easily recycled or upcycled reduce waste and conserve resources.
- Durability: Long-lasting materials reduce the need for frequent replacements, saving both resources and energy.
- Non-Toxicity: Using non-toxic materials is better for indoor air quality and occupant health.
Sustainable Material | Benefits |
Bamboo | Fast-growing and renewable, reduces deforestation |
Recycled Steel | Reduces need for new material production |
Rammed Earth | Provides natural insulation and is abundant |
Exploring deeper into sustainable material use, the concept of 'closed-loop material cycles' is gaining traction. This approach involves designing materials and products so that they can continually circulate within production systems, eliminating waste and the need for new raw material extraction. It aligns with the principles of a circular economy, where materials are kept in use for as long as possible, maximizing value and minimizing impact. Closed-loop systems propose a revolutionary shift in how we perceive material usage, encouraging industries to innovate in both design and production processes to create eco-friendly solutions that stand the test of time.
Energy Efficiency in Buildings focuses on optimizing energy consumption, reducing waste, and creating environmentally friendly structures.
Energy efficiency in buildings is crucial as it significantly reduces overall energy consumption, contributing to the mitigation of climate change. Employing energy-efficient practices in building design and operation can lead to cost savings, reduce the carbon footprint, and enhance the comfort and health of occupants. Aiming for high energy efficiency standards promotes sustainable development.
Importance of Energy Efficiency for Ecological Footprints highlights the role energy use plays in environmental impact.
Energy efficiency in buildings directly influences their ecological footprint, which measures the impact on the natural environment. Here are key reasons why energy efficiency is important:
- Reduction of Carbon Emissions: Minimizes the greenhouse gases released into the atmosphere.
- Conservation of Resources: Less energy use reduces dependency on fossil fuels.
- Cost Savings: Lower energy bills due to reduced consumption.
Implementing energy-efficient appliances can immediately reduce a building's ecological footprint.
Consider a building using double-glazed windows, which significantly reduces the need for heating and cooling, thereby lowering energy use and emissions.
Techniques to Enhance Energy Efficiency in Buildings involve a variety of innovative practices and technologies.
To enhance energy efficiency, buildings can incorporate several techniques:
- Insulation Improvements: Properly insulating walls, roofs, and floors to minimize heat loss.
- Passive Solar Design: Maximizing natural sunlight to reduce reliance on artificial lighting.
- Smart Thermostats: Automatically regulating heating and cooling for optimal energy use.
- Energy-Efficient Lighting: Utilization of LED and sensor-based systems to manage lighting efficiently.
Energy Efficiency in buildings is achieved by reducing the amount of energy required to maintain indoor comfort, promote sustainability, and minimize environmental impacts.
A detailed exploration into energy efficiency reveals the significance of the building envelope— the physical separator between the interior and the exterior environments. By enhancing the thermal performance of the envelope through superior materials and construction techniques, it is possible to reduce heating and cooling loads significantly. This approach synergizes with techniques like the use of phase change materials (PCMs) that absorb or release heat as they change state, offering additional temperature regulation without external energy inputs.
Energy Efficient Building Technologies include groundbreaking innovations designed to maximize energy conservation.
The rise of new technologies has pushed boundaries in energy-efficient building practices:
- Photovoltaics (PV): Solar panels that convert sunlight directly into electricity, reducing dependence on grid energy.
- Geothermal Systems: Utilize the stable temperature of the ground to efficiently heat and cool buildings.
- Building Automation Systems (BAS): Integrate and automate climate control, lighting, and security to optimize energy use.
- Advanced Glazing Technologies: Windows that dynamically adjust their transparency for solar control and insulation benefits.
Technology | Benefit |
Photovoltaics | Reduced reliance on grid with renewable energy |
Geothermal Systems | Efficient temperature regulation |
BAS | Streamlined energy management |
Advanced Glazing | Enhanced thermal comfort |
ecological footprints - Key takeaways
- Ecological Footprint Definition: Measures the land and water area required by a population to produce resources and absorb waste, crucial in architecture for environmental impact assessment.
- Sustainability in Architecture: Focuses on designing buildings that minimize environmental impact, maximize resource efficiency, and promote occupant wellbeing.
- Green Architecture Principles: Include energy efficiency, water conservation, sustainable materials, and enhancing indoor environmental quality.
- Carbon Footprint in Construction: Refers to greenhouse gas emissions from building activities including material production, transportation, and energy consumption.
- Resource Consumption in Building: Entails efficient use of energy, materials, and water to reduce environmental impacts and promote sustainability.
- Energy Efficiency in Buildings: Optimizing energy use to lower carbon emissions, conserve resources, and support sustainable architecture.
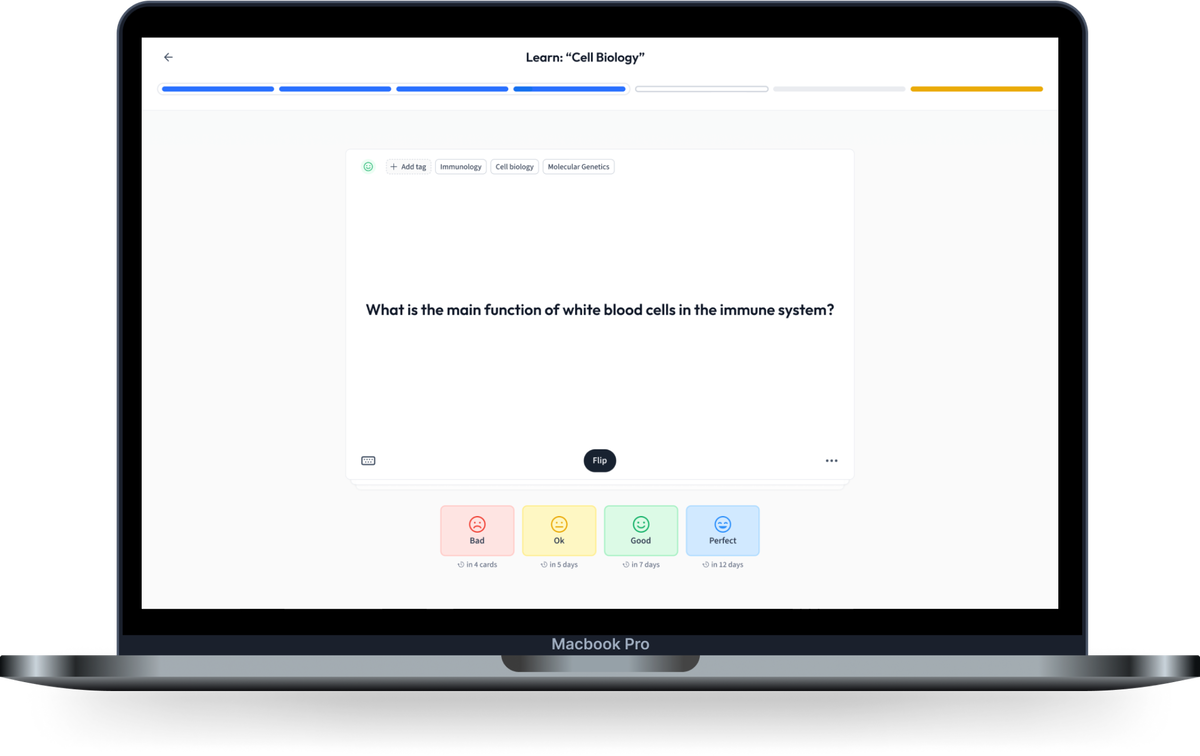
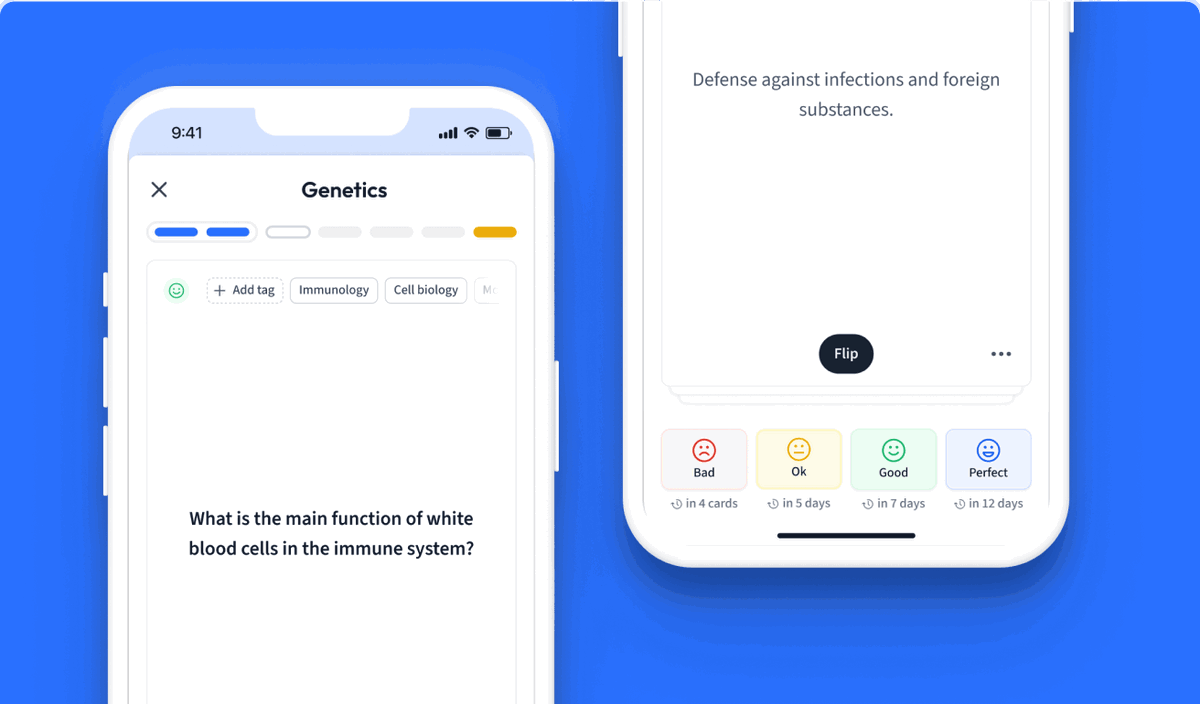
Learn with 12 ecological footprints flashcards in the free StudySmarter app
Already have an account? Log in
Frequently Asked Questions about ecological footprints
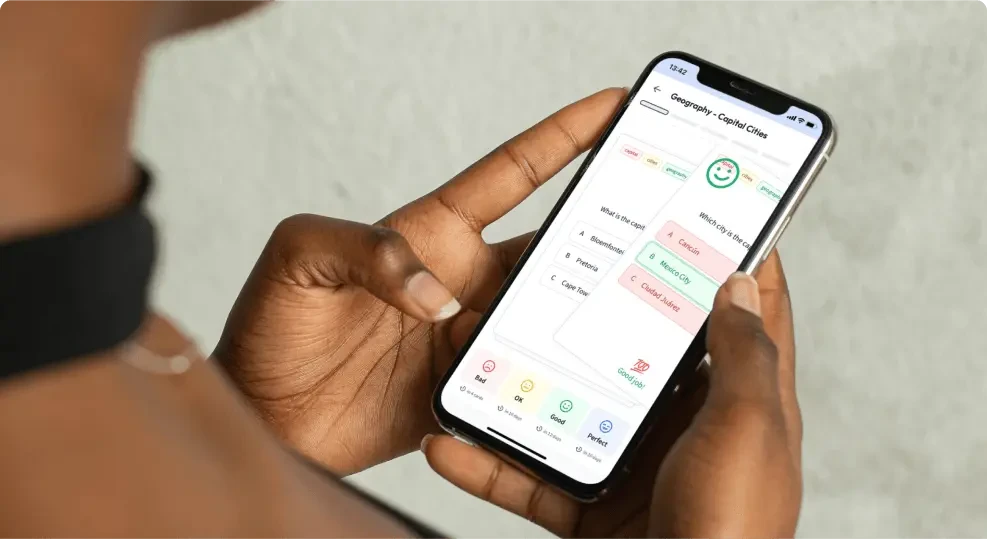
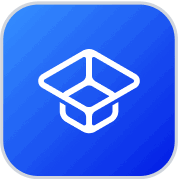
About StudySmarter
StudySmarter is a globally recognized educational technology company, offering a holistic learning platform designed for students of all ages and educational levels. Our platform provides learning support for a wide range of subjects, including STEM, Social Sciences, and Languages and also helps students to successfully master various tests and exams worldwide, such as GCSE, A Level, SAT, ACT, Abitur, and more. We offer an extensive library of learning materials, including interactive flashcards, comprehensive textbook solutions, and detailed explanations. The cutting-edge technology and tools we provide help students create their own learning materials. StudySmarter’s content is not only expert-verified but also regularly updated to ensure accuracy and relevance.
Learn more