Jump to a key chapter
Definition of Structural Resilience
Structural resilience refers to the ability of a structure to withstand and recover from unforeseen events such as natural disasters, accidents, or other disruptions. It is an essential consideration in architecture, ensuring buildings and infrastructure maintain their functionality and safety. By designing structures with resilience in mind, architects and engineers can reduce the potential impact of catastrophic events.
Key Components of Structural Resilience
When discussing structural resilience, there are several critical components to consider that contribute to the overall resilience of a building or structure. These include:
- Redundancy: This involves duplicating critical components within a structure so that if one part fails, another can take over.
- Robustness: The ability of a structure to withstand external forces without experiencing failure.
- Resourcefulness: The capacity to identify problems and implement solutions rapidly during unforeseen events.
- Recovery: The ability to quickly return to normal operations after an incident or disruption.
Structural Resilience: The capability of a structure to endure and recover from disturbances while preserving essential functions.
An example of structural resilience can be seen in earthquake-resistant buildings. These buildings are designed with flexible foundations and reinforced materials to absorb and dissipate seismic energy, allowing them to remain standing even during intense shaking. As a result, they minimize potential damage and ensure the safety of occupants.
Structural resilience is not just about the physical attributes of a building but also involves comprehensive planning and analysis. Risk assessments are performed to identify potential vulnerabilities and impact scenarios. Data collected from past disasters are analyzed to improve design standards and materials. Building codes are continuously updated to enhance structural resilience. Moreover, structural modeling helps in predicting how different designs will behave under stress, contributing to the development of more resilient structures. Through these strategies, the odds of buildings withstanding severe conditions are greatly increased.
Did you know that some of the world's tallest skyscrapers are specially designed to sway during strong winds to enhance their structural resilience?
Importance of Structural Resilience in Architecture
Structural resilience plays a critical role in the field of architecture by safeguarding buildings and infrastructure against unforeseen challenges. In today's world, where environmental and human-made threats are prevalent, it is more important than ever to construct buildings that can endure these challenges without compromising their integrity or function. Structural resilience ensures that you, the occupants, remain safe and services continue to operate effectively.
Benefits of Structural Resilience
Understanding the benefits of structural resilience can help you appreciate the necessity of incorporating it into architectural designs. Some of the primary benefits include:
- Safety: Structural resilience improves the safety of buildings, minimizing the risk of harm to individuals during and after disastrous events.
- Cost-effectiveness: While initial construction costs may be higher, resilient buildings save money over time due to reduced damage and repair costs.
- Continuity of Operations: Resilient structures ensure that businesses and other essential services can continue functioning during crises.
- Environmental Protection: Reducing the need for reconstruction contributes to less waste and resource consumption.
Structural Resilience: The ability of a structure to withstand and recover from abrupt impacts like natural disasters, maintaining its function and safety.
Consider flood-resistant homes in coastal areas. These homes are designed with elevated foundations, water-resistant barriers, and robust drainage systems. This design approach not only prevents water ingress during floods but also ensures that homes remain safe and operational, highlighting a practical application of structural resilience.
The importance of structural resilience extends beyond individual structures to entire communities. Urban planners and architects collaborate to create resilient cities capable of withstanding wide-scale disruptions. This involves planning for infrastructure such as power supplies and transportation that can quickly recover or adapt. By utilizing advanced modeling and simulations, professionals can predict future scenarios and incorporate features into designs to enhance resilience. The integration of technology, like sensors and automated response systems, also contributes to real-time damage assessment and adaptive responses during emergencies.
Resilient architecture is vital for historic buildings as it preserves cultural heritage by incorporating modern technologies while maintaining the original aesthetics.
Techniques for Achieving Structural Resilience
To achieve structural resilience, architects and engineers must utilize a variety of techniques that enhance a building's ability to withstand and recover from disruptions. These approaches ensure that buildings remain safe, functional, and adapted to their specific environment.
Design Innovations for Resilience
Designing for resilience involves several innovative techniques that can significantly boost a structure’s durability:
- Flexible Materials: Using materials that can bend without breaking enhances resilience, particularly against earthquakes and high winds.
- Energy Dissipation Devices: Installing devices such as dampers and base isolators reduces stress by absorbing energy from movements like tremors.
- Modular Construction: Prefabricating components allows for rapid assembly and repair, benefiting recovery efforts.
An iconic example is the Taiwan Tower, which uses tuned mass dampers to counteract the swaying motion caused by typhoons and earthquakes. This innovative approach keeps the building stable and ensures the safety of its occupants.
Material Selection and Use
Choosing the right materials is crucial for enhancing structural resilience, as different materials offer varying levels of strength and flexibility.
Material | Key Property |
Concrete | High compressive strength |
Steel | Excellent tensile strength |
Wood | Natural flexibility |
Material Science advancements continue to play a pivotal role in structural resilience. Researchers are developing self-healing concrete that automatically repairs cracks. Such innovations minimize structural degradation and extend the lifespan of buildings. In addition, bio-inspired designs are leading to materials that better mimic nature's resilience. For example, some new materials emulate the lightweight yet sturdy structure of bones, offering unprecedented strength-to-weight ratios. These advancements provide exciting opportunities to further strengthen the resilience of future constructions.
Incorporating locally sourced materials in construction not only enhances sustainability but can also improve resilience by choosing materials that naturally withstand local environmental conditions.
Examples of Resilient Structures
Resilient structures demonstrate exceptional ability to endure and recover from disruptions such as natural disasters. These structures serve as models of effective architectural design that prioritize safety and sustainability in unpredictable environments.
Disaster Resilient Structures
Designing for disaster resilience involves incorporating features that help buildings withstand extreme conditions such as earthquakes, floods, and hurricanes. This approach ensures that architectural designs prioritize the safety of inhabitants and the continuity of operations.
A well-known example of a disaster resilient structure is the Burj Khalifa in Dubai. This skyscraper incorporates a Y-shaped triple buttressed core, designed to resist powerful winds and seismic activity. Its robust foundation and high-strength concrete give it the flexibility and strength required for such a tall structure.
Disaster Resilience: The ability of a structure to efficiently withstand, adapt to, and rapidly recover from severe natural events.
- Robust Foundations: Buildings in earthquake-prone areas often feature deep foundations and base isolators that absorb vibrations.
- Flood Barriers: Structures at risk of flooding incorporate elevated designs and water-resistant materials to prevent water ingress.
- Wind Load Engineering: In regions prone to hurricanes, buildings are designed with aerodynamic shapes and reinforced materials to withstand strong winds.
Advanced technologies are used to simulate disaster scenarios, improving the ability to engineer structures that anticipate and mitigate potential damage. For instance, computational fluid dynamics is used to model how storm surges could impact a building's design. Innovations in smart materials that adjust their properties in response to environmental stimuli are also being explored. These materials offer the potential for real-time adaptations, providing a dynamic response to disaster conditions. This cutting-edge research enhances our understanding of how to create structures that not only survive but thrive amidst adversity.
In resilient construction, strategic landscaping can also aid in disaster management, such as using vegetation to reduce flood impact and soil erosion.
structural resilience - Key takeaways
- Structural Resilience: The ability of a structure to endure and recover from disruptions, maintaining its function and safety.
- Importance in Architecture: Ensures buildings withstand challenges without compromising integrity; essential for safety and operational continuity.
- Resilient Structures: Examples include earthquake-resistant buildings with flexible foundations and disaster-resistant designs like the Burj Khalifa.
- Disaster Resilient Structures: Incorporate robust foundations, flood barriers, and wind load engineering to withstand natural events.
- Structural Resilience Techniques: Utilize redundancy, robustness, resourcefulness, and recovery along with design innovations like flexible materials and energy dissipation devices.
- Examples and Techniques: Use of tuned mass dampers and modular construction for resilience; material selection crucial for strength and flexibility.
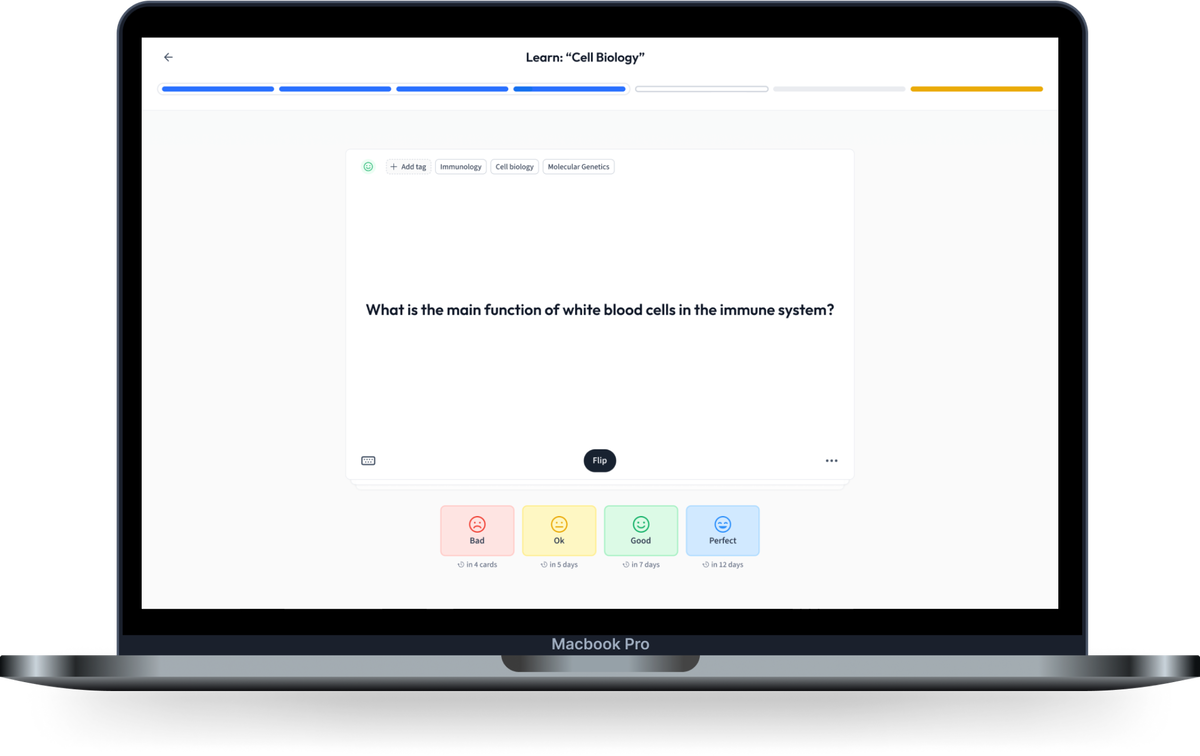
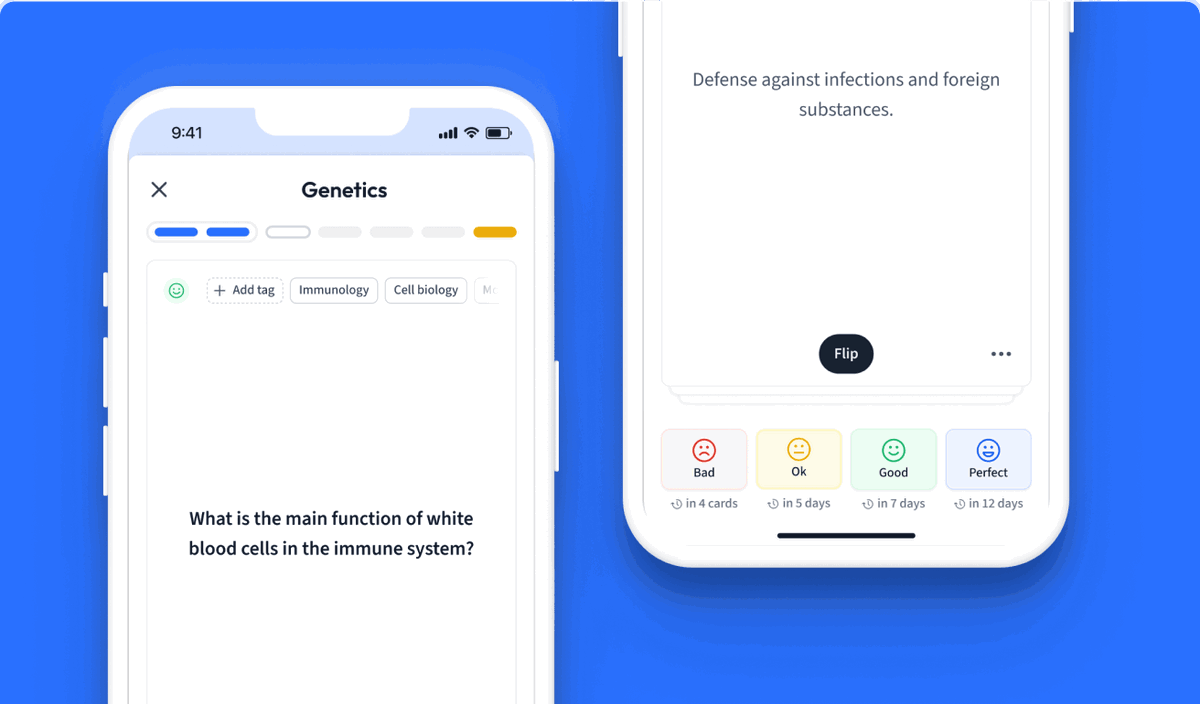
Learn with 12 structural resilience flashcards in the free StudySmarter app
Already have an account? Log in
Frequently Asked Questions about structural resilience
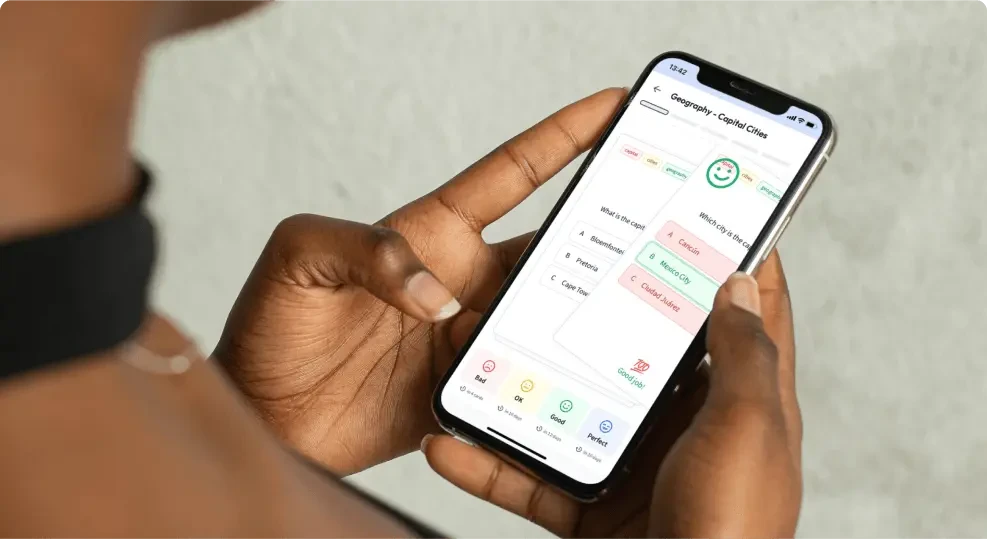
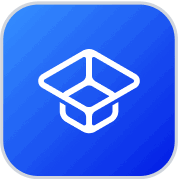
About StudySmarter
StudySmarter is a globally recognized educational technology company, offering a holistic learning platform designed for students of all ages and educational levels. Our platform provides learning support for a wide range of subjects, including STEM, Social Sciences, and Languages and also helps students to successfully master various tests and exams worldwide, such as GCSE, A Level, SAT, ACT, Abitur, and more. We offer an extensive library of learning materials, including interactive flashcards, comprehensive textbook solutions, and detailed explanations. The cutting-edge technology and tools we provide help students create their own learning materials. StudySmarter’s content is not only expert-verified but also regularly updated to ensure accuracy and relevance.
Learn more