Jump to a key chapter
Introduction to Drainage Design
Drainage design is an essential component of architecture and civil engineering. It involves strategizing and implementing systems that efficiently remove excess water from an area to avoid damage and flooding. Proper drainage systems are crucial for maintaining the longevity and structural integrity of buildings and infrastructure.
Components of Drainage Systems
A well-designed drainage system comprises several vital components each serving a unique purpose:
- Surface drains: Collects surface water and directs it to designated drainage areas.
- Subsurface drains: Installed below ground to manage groundwater.
- Culverts: Channels water below physical obstacles like roads.
- Gutters and downspouts: Collect and direct water from rooftops.
Surface Drain: A drainage element designed to collect and channel surface-level water to a designated area, preventing pooling and waterlogging.
Mathematical Modeling in Drainage Design
Mathematical models play a vital role in drainage design, providing engineers with tools to predict and analyze water flow. Formulas such as the Manning's equation are used to assess the flow rate of water through channels.For example, the Manning's equation is:\[ Q = \frac{1}{n}A R^{2/3}S^{1/2} \]Where:
- \(Q\) is the flow rate
- \(n\) is the Manning's roughness coefficient
- \(A\) is the cross-sectional area
- \(R\) is the hydraulic radius
- \(S\) is the slope of the energy grade line
To illustrate Manning's equation:Imagine a rectangular channel with a width of 2 meters and a depth of 1 meter. If the slope \(S\) is 0.001 and the roughness coefficient \(n\) is 0.015, you can calculate the flow rate \(Q\) as follows:\[ A = 2 \, m \, \times \, 1 \, m = 2 \, m^2 \]\[ R = \frac{2 \, m}{1 + 2 \, m \, \times \, 1 \, m / 2 \, m} = 0.6667 \, m \]\[ Q = \frac{1}{0.015} \, \times \, 2 \, m^2 \, \times \, 0.6667^{2/3} \, \times \, 0.001^{1/2} \approx 3.62 \, m^3/s \]This demonstrates using mathematical techniques in drainage design.
Considerations in Drainage Design
When designing a drainage system, consider the following factors:
- Topography: The natural landscape slope impacts water flow and drainage direction.
- Soil type: Soil permeability dictates the amount of water absorbed or directed away.
- Climate: Weather patterns influence drainage requirements, particularly in high rainfall areas.
- Urbanization: Infrastructure can alter natural water flow, demanding innovative drainage solutions.
Remember, while designing drainage, it is essential to balance between environmental impact and infrastructural needs.
The complexity of drainage systems often requires a multidisciplinary approach, involving hydrologists, civil engineers, and environmental planners. Emerging technologies, such as smart drainage systems, integrate sensors and automation to optimize water flow management in real-time. These systems analyze weather data, predict flooding, and adjust drainage operations accordingly, enhancing resilience against climatic changes. Moreover, innovative materials like permeable concrete are being increasingly used to allow water to pass through surfaces. This decreases runoff and aids groundwater recharge, showcasing a shift towards sustainable urban design. The integration of green infrastructure, such as rain gardens and bioswales, supports natural water infiltration, further aligning urban drainage solutions with ecological principles.
Architectural Impact of Drainage Design
In architecture, drainage design plays a pivotal role in ensuring that structures remain safe and long-lasting. Drainage systems not only protect buildings from water damage but also add value by enhancing their functionality and aesthetics.
Understanding Its Role in Architecture
The role of drainage design in architecture can be understood through its contribution to various aspects:
- Structural Integrity: Proper drainage prevents soil erosion and shifts in the foundation.
- Safety: Efficient drainage reduces the risk of water damage, mold growth, and structural failures.
- Compliance: Meeting building codes and regulations often requires effective drainage planning.
- \(Q\) is the peak discharge
- \(C\) is the runoff coefficient
- \(i\) is the rainfall intensity
- \(A\) is the drainage area
Rational Method: A formula used in civil engineering to estimate the peak discharge from a catchment area by considering factors like rainfall intensity, area, and runoff characteristics.
A deeper exploration into drainage reveals that advancements in technology are shaping innovative drainage solutions. Permeable surfaces are one such innovation, allowing water to infiltrate and reduce runoff. Integrating smart drainage systems that leverage IoT for real-time monitoring and adjustments is becoming increasingly popular. Smart sensors can adjust drain flow based on weather forecasts, optimizing water management. Furthermore, green roofs and rain gardens are examples of eco-friendly solutions that complement urban drainage systems by incorporating vegetation that absorbs rainwater, reducing runoff and promoting biodiversity in urban settings.
Aesthetic and Functional Considerations
Drainage systems must balance functionality with aesthetics. Thoughtful design can enhance a building's visual appeal while providing crucial water management. Considerations include:
- Design Integration: Incorporating drainage elements that blend seamlessly with the structure, like concealed gutters or stylish rain chains.
- Material Selection: Utilizing materials that are both durable and aesthetically pleasing, such as decorative grates or stone channels.
- Landscaping: Using greenery and water features to naturally manage water flow and add visual interest.
When designing drainage systems, remember that the choice of materials and integration with the landscape can significantly influence aesthetic outcomes.
Roadside Drainage Ditch Design
The design of roadside drainage ditches is critical to managing water runoff and preventing flooding on roads. These ditches help maintain the safety and longevity of transportation infrastructure by efficiently directing water away from surfaces. Effective design incorporates key features and benefits while addressing common challenges.
Key Features and Benefits
Roadside drainage ditches have several essential features that serve specific functions:
- Shape and Slope: Ensures effective water flow and prevents erosion. Typically, a trapezoidal shape with a gentle side slope is used.
- Vegetation: Helps stabilize soil and manage water flow by promoting infiltration.
- Lining Material: Use of concrete, riprap, or grass to prevent erosion and blockages.
- Connectivity: Ditches are connected to culverts and stormwater systems to facilitate water movement.
- Flood Prevention: Reducing the risk of water pooling on roadways increases safety.
- Soil Stabilization: Prevents erosion and roadbed deterioration.
- Environmental Impact: Minimizes negative effects on local ecosystems by managing water runoff effectively.
Consider a roadside ditch with a width of 3 meters at the top and 1 meter deep. If the side slopes are 1:3 (vertical:horizontal), the area can be calculated as:\[ A = \left( B + Z_1 \times L + Z_2 \times L \right) \times d \]where:
- \(B = 3 \text{ m}\)
- \(Z_1 = Z_2 = 3\)
- \(d = 1 \text{ m}\)
Common Challenges and Solutions
Designing roadside drainage ditches can present several challenges, but effective solutions exist:
- Challenge: Erosion from High Flow RatesSolution: Employ ditch linings and check dams to manage velocity and protect soil.
- Challenge: Sediment AccumulationSolution: Regular maintenance and sediment trapping features help in keeping ditches clear.
- Challenge: Vegetation OvergrowthSolution: Implement a maintenance schedule to trim overgrowth and maintain ditch capacity.
Riprap: A layer of large stones or concrete used to protect soil from erosion, especially in areas exposed to high flow rates.
Regular inspection of roadside ditches helps identify early signs of failure, allowing timely interventions.
Beyond basic design, current trends in drainage ditch design integrate green infrastructure and climate resiliency strategies. Engineers are exploring innovative materials that mimic natural landscapes, such as bioengineered banks, which use plants and biodegradable materials to stabilize slopes while enhancing habitat quality. In urban settings, permeable ditches double as green spaces to absorb runoff and filter pollutants, contributing to urban heat reduction and biodiversity. Additionally, using GIS and remote sensing technology in planning helps model water flow pathways, predict erosion patterns, and optimize ditch layouts for varying rain events. This integration of technology not only improves performance but also aligns with sustainable urban development goals.
Drainage Design Theory
Understanding the theory behind drainage design is crucial for architects and engineers as it involves the strategic removal and management of water to protect structures and the environment. Learning the principles and historical context aids in grasping its importance and application.
Principles and Concepts
Drainage design revolves around several core principles that ensure efficiency and functionality:
- Hydraulic Efficiency: The system must effectively manage water flow to prevent flooding and related damage.
- Environmental Impact: Protect and enhance the surrounding ecosystem by considering sustainable practices.
- Maintenance and Durability: Systems should be easy to maintain and have a long lifecycle to maximize reliability.
- \(Q\) is the peak flow rate
- \(C\) is the runoff coefficient, reflecting the nature of the surface
- \(i\) is the rainfall intensity
- \(A\) is the area of the catchment
An application of the Rational Formula involves a parking lot: Consider a paved area of 5,000 square meters, a runoff coefficient (\(C\)) of 0.9 for impermeable surfaces, and a rainfall intensity (\(i\)) of 12 mm/hour, the peak flow rate \(Q\) can be calculated as:\[ Q = 0.9 \times 12 \text{ mm/hour} \times 5000 \text{ m}^2\approx 54,000 \text{ liters/hour}\]Such calculations allow for designing a drainage system that manages stormwater effectively.
Remember to adjust runoff coefficients based on the permeability of surfaces to improve formula accuracy.
Some fascinating advancements in drainage design involve simulating complex hydrological scenarios using computer models. These models factor in detailed landscape data, predicting flood behavior under various climatic conditions. By using Geographic Information Systems (GIS), designers can visualize drainage patterns in three dimensions, improving decision-making. Moreover, the application of machine learning technologies allows for the analysis of vast datasets, providing insights into weather patterns and drainage performance over time. Such technologies contribute to developing smarter systems that enhance urban resilience against water-related issues.
Historical Development of Drainage Design
The evolution of drainage design mirrors advances in technology and understanding of hydrology. Historically, drainage systems were simple and localized, often influenced by cultural and local needs
- In ancient Rome, aqueducts and sewer systems marked some of the earliest engineered drainage infrastructures.
- During the Middle Ages, settlements often relied on natural streams, with little formal drainage design.
- The industrial revolution spurred advancements in materials and construction techniques, allowing for larger, more efficient drainage networks.
- Modern times have introduced eco-friendly practices, emphasizing sustainability and environmental consideration
Drainage Design Examples
Exploring real-world drainage design examples provides valuable insights into effective strategies and innovations. It is crucial to analyze successful case studies to understand the principles and methods applied in diverse scenarios.
Notable Case Studies
Several noteworthy case studies highlight the importance and diversity of drainage design approaches:
- Singapore's ABC Waters Programme: This initiative focuses on integrating drainage and water management into the urban landscape. Utilizing strategies like bioretention swales and river naturalization, it showcases sustainable urban drainage systems.
- London's Thames Barrier: Protecting against tidal surges, this large movable barrier demonstrates the scale at which drainage infrastructure operates in safeguarding urban areas from flooding.
- Netherlands' Delta Works: A sophisticated network of dams, sluices, and storm surge barriers that represent holistic flood management, safeguarding areas below sea level.
In Singapore's ABC Waters Programme, bioretention swales are used to treat runoff water naturally. Here’s how their utility is evaluated:The volume of runoff (\ref{x}) managed by the swales can be calculated using:\[ V = C_i A \times t \]Where:
- \( C_i \) is the inflow concentration reduced by treatment efficiency.
- \( A \) is the catchment area managed by the swale.
- \( t \) is the retention time for water purification.
Behind impressive drainage projects like the Netherlands' Delta Works lies extensive research in hydrodynamics. Engineers employ complex simulation models to understand the behavior of water systems under stress scenarios such as heavy rainfall and sea level rise. The integration of modern technology such as infrastructure resilience assessments using digital twins – virtual replicas of physical assets – helps in predicting maintenance needs and potential failure points. By continuously monitoring and updating these models, engineers and planners can equip their designs with better adaptable solutions for climate change, making them robust and future-ready.
Lessons Learned
Analyzing these drainage design examples teaches several important lessons that inform future planning and execution:
- Importance of Local Context: Effective drainage design requires a thorough understanding of local environmental conditions and societal needs.
- Integration with Urban Planning: Combining drainage infrastructure with urban development plans improves resilience and optimizes land use.
- Use of Technology: Advanced modeling and real-time data integration are essential for planning and maintaining robust drainage systems.
- Sustainability: Incorporating natural elements like green roofs and permeable pavements enhances ecological balance and integrates drainage systems with the environment.
Residential Drainage Swale Design
Drainage swales are vital elements in residential design for managing stormwater runoff effectively. They are specially structured channels that facilitate water movement away from critical areas, minimizing the risk of flooding and erosion.
Designing for Efficiency
To ensure residential drainage swales are efficient, several design considerations are important:
- Grading and Slope: Proper grading is crucial for controlling water flow direction. The slope is typically gentle to guide water slowly, preventing erosion and allowing for water infiltration into the soil.
- Vegetation: Planting grasses or native plants within the swale can stabilize soil, slow water flow, and increase infiltration.
- Shape and Depth: A parabolic or trapezoidal shape is often used to handle varying water volumes while maintaining stability.
- \(Q\) is the flow rate
- \(n\) is the roughness coefficient
- \(A\) is the cross-sectional area
- \(R\) is the hydraulic radius
- \(S\) is the slope
Consider a swale with a width of 1.5 meters at the bottom, side slopes of 1:4, and a depth of 0.5 meters. The slope of the channel (\(S\)) is 0.005, and the roughness coefficient (\(n\)) is 0.04 due to vegetation.Calculate the cross-sectional area \(A\):\[ A = \left( B + ZD \right) D = (1.5 + 4 \cdot 0.5) \cdot 0.5 = 1.75 \text{ m}^2 \]Substitute into Manning's equation to estimate flow rate.
In-depth exploration of swale design often involves integrating ecological benefits. Swales can serve as essential habitats for wildlife, promoting biodiversity while managing stormwater. Techniques such as bioswales incorporate soil and sand layers to filter pollutants from runoff naturally. The selection and arrangement of plant species play a critical role not only in water management and erosion prevention but also in enhancing the aesthetic and ecological value of residential areas. Using swales creatively in landscape architecture can transform urban and rural spaces, aligning with sustainable environmental practices.
Urban vs Rural Swale Design
The design of drainage swales varies significantly between urban and rural environments due to differences in land use, water flow requirements, and space availability.Urban Swale Design:
- Typically narrower to fit within urban spaces.
- Focus on controlling high volumes of surface runoff efficiently.
- Frequent use of reinforced materials to prevent erosion.
- Usually wider with gentle slopes to accommodate larger natural landscapes.
- Emphasizes natural vegetation for stabilization and filtration.
- Designed to blend seamlessly with the rural environment, enhancing the landscape while managing water.
When designing swales, consider local climate and soil conditions to enhance water management efficiency and ecological benefits.
Excavation Techniques in Drainage Design
Excavation plays a crucial role in drainage design, providing the necessary foundation and shape for drainage systems like channels, trenches, and pipes. Understanding effective excavation techniques ensures the stability and efficiency of the drainage infrastructure.
Tools and Equipment
Successful excavation for drainage design requires the use of specialized tools and equipment suited to different site conditions and project scales. Commonly used excavation tools include:
- Excavators: Versatile machines available in various sizes, used for large-scale earth moving and trench digging.
- Backhoes: Ideal for digging narrow trenches, especially in urban and confined spaces.
- Trenchers: Specifically designed to dig trenches for laying drainage pipes, offering precision and efficiency.
- Shovels and Spades: Essential for manual digging and fine-tuning trench dimensions.
For instance, on a soft soil site where compact trenches are needed for pipe installation, a trencher can be used to achieve precise cutting and consistent depth. A trencher can efficiently handle soil displacement, minimizing manual labor and ensuring the trench is ready for subsequent drainage pipe placement.
Always assess the soil conditions and project requirements before selecting excavation equipment to improve efficiency and safety on site.
Best Practices in Excavation
Best practices are essential to ensure that excavation for drainage systems is carried out safely, efficiently, and sustainably. Key practices include:
- Site Assessment: Conduct thorough assessments to understand soil types, water table levels, and potential hazards.
- Precision Planning: Use detailed site plans and markers to outline the exact excavation areas and depths to avoid over-excavation.
- Safety Protocols: Implement and adhere to safety guidelines to protect workers from potential risks like landslides or equipment accidents.
- Environmental Considerations: Plan to minimize environmental impact by managing displaced soil and avoiding natural habitat disruption.
Engaging in sustainable excavation practices involves considering advanced techniques such as using geotextiles and erosion control measures. For instance, geotextiles can stabilize the excavated area, preventing erosion and improving the structural integrity of the drainage system. Technology like GPS and laser guidance systems further enhance precision, ensuring that excavation adheres strictly to design specifications. Such innovations reduce material waste, decrease environmental disruption, and improve overall efficiency.
drainage design - Key takeaways
- Drainage Design: Planning and implementing systems to remove excess water, ensuring the longevity and structural integrity of buildings and infrastructure.
- Architectural Impact of Drainage Design: Enhances building safety, structural integrity, and compliance with regulations through efficient water management.
- Roadside Drainage Ditch Design: Critical for managing road runoff and preventing erosion, utilizing features like shape, slope, and vegetation.
- Drainage Design Theory: Incorporates hydraulic efficiency, environmental impact, and maintenance, utilizing mathematical models like the Manning's equation and Cubic Rational Formula.
- Residential Drainage Swale Design: Uses elements like grading and vegetation to manage stormwater, incorporating ecological and landscape design principles.
- Excavation Techniques in Drainage Design: Utilizes specialized equipment and best practices for site assessment, precision planning, and sustainability in drainage infrastructure.
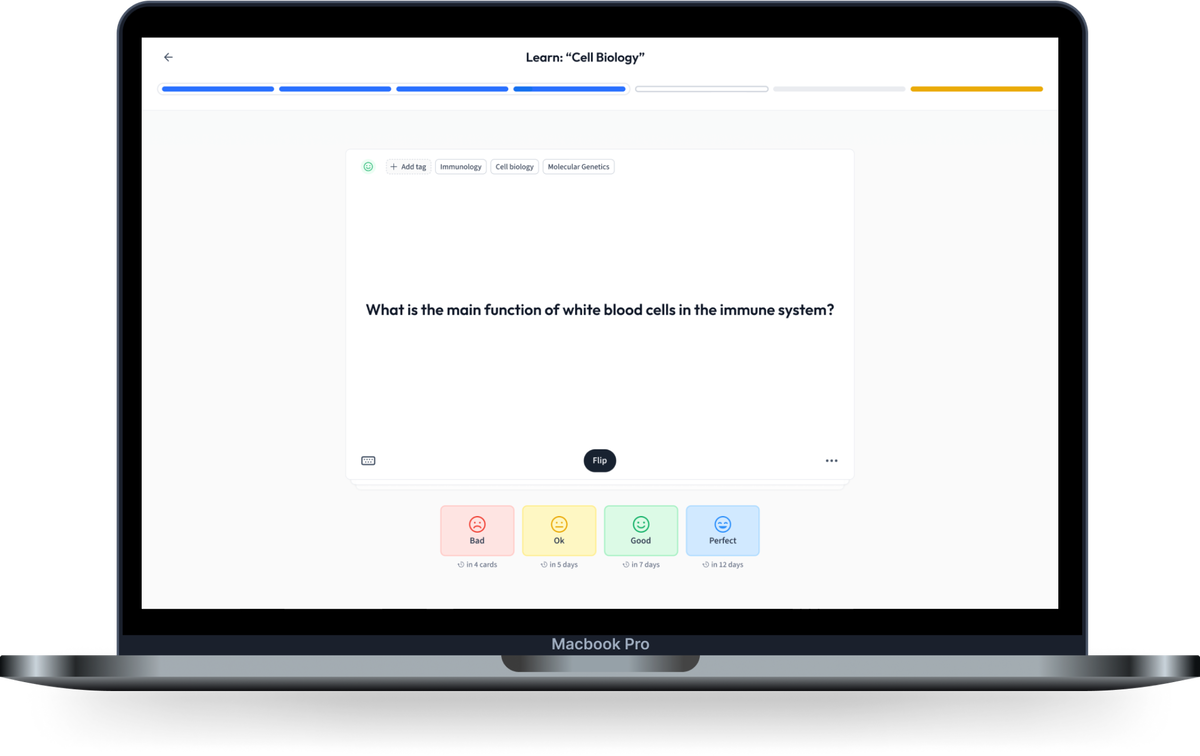
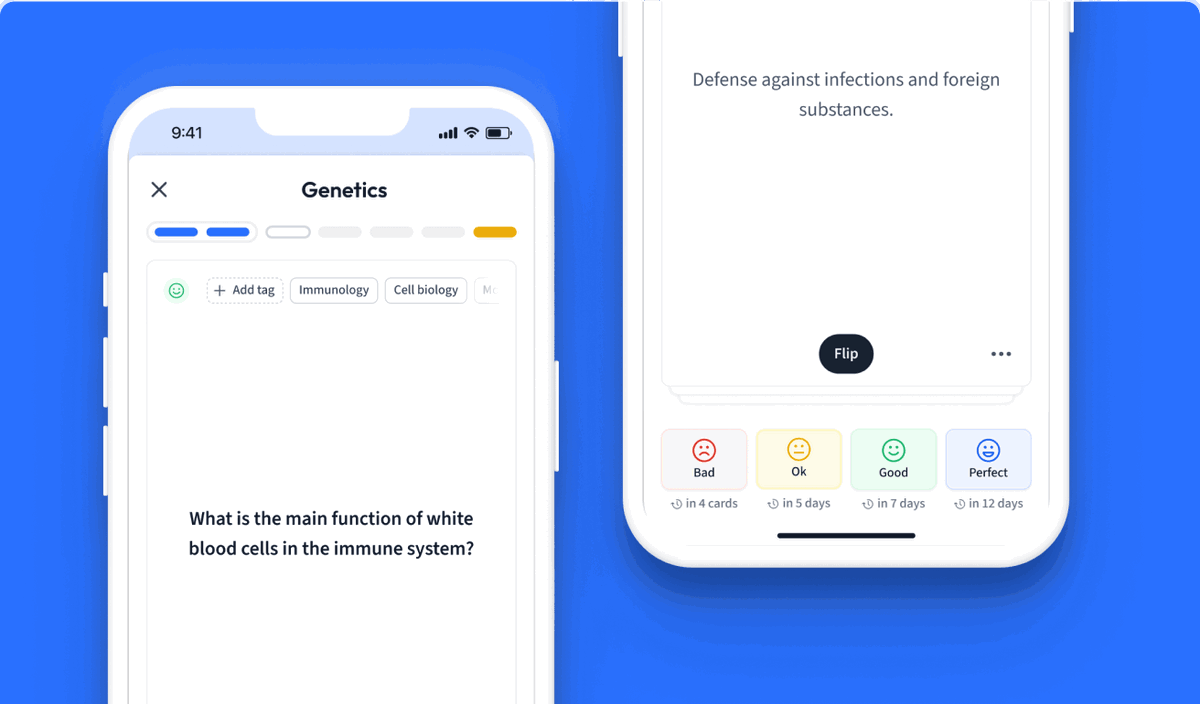
Learn with 14 drainage design flashcards in the free StudySmarter app
Already have an account? Log in
Frequently Asked Questions about drainage design
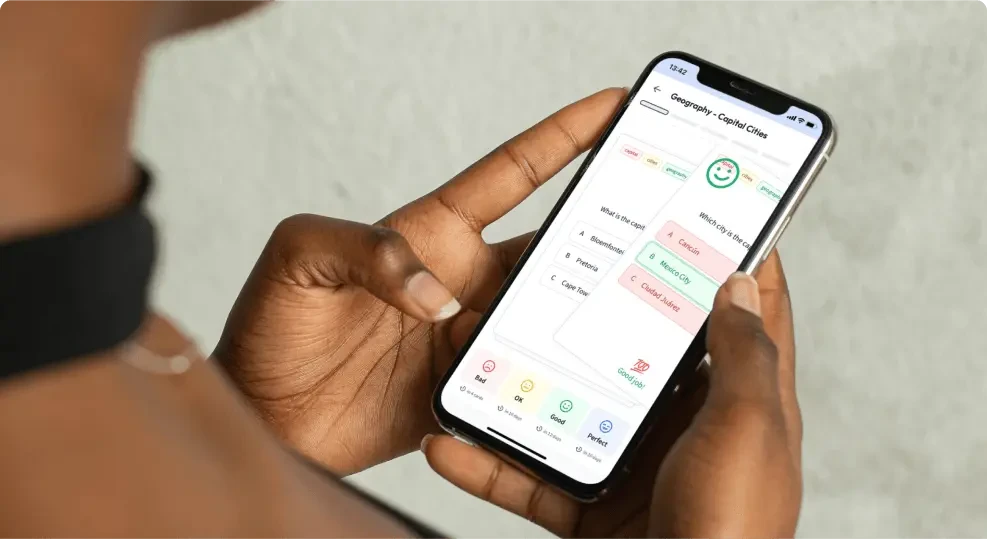
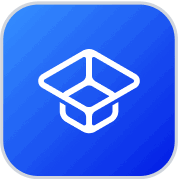
About StudySmarter
StudySmarter is a globally recognized educational technology company, offering a holistic learning platform designed for students of all ages and educational levels. Our platform provides learning support for a wide range of subjects, including STEM, Social Sciences, and Languages and also helps students to successfully master various tests and exams worldwide, such as GCSE, A Level, SAT, ACT, Abitur, and more. We offer an extensive library of learning materials, including interactive flashcards, comprehensive textbook solutions, and detailed explanations. The cutting-edge technology and tools we provide help students create their own learning materials. StudySmarter’s content is not only expert-verified but also regularly updated to ensure accuracy and relevance.
Learn more