Jump to a key chapter
Photon Counting Definition
Photon counting is an essential concept in science and technology, particularly in fields like physics, chemistry, and biology. It involves measuring the number of photons, which are particles of light, that hit a detector over a certain period of time. This method is pivotal in ensuring accurate measurements in various experimental and practical applications.
Photon Counting in Biology
In biological sciences, photon counting is applied in several domains:
- Fluorescent Microscopy: This technique helps in imaging cells and tissues by detecting fluorescent signals that are emitted by labeled molecules when they are hit by photons.
- Bioluminescence Imaging: Used to study cellular and molecular processes in organisms, this technique measures light produced by biochemical reactions within living organisms.
- DNA Sequencing: Advanced sequencing technologies use photon counting to detect and analyze signals emitted during the sequencing processes.
Photon counting offers significant advantages in terms of sensitivity and precision, making it a powerful tool for biological research.
Photon Counting: A technique for measuring the number of photons in a given sample or emitted from a light source, often used for high-precision measurements in scientific research.
Example of Photon Counting: A researcher studying the effectiveness of a new drug might use photon counting to track the expression of a luminescent gene in response to the treatment. By measuring the light emitted, the researcher can deduce gene expression levels.
Deep Dive into Photon Counting Technology:Photon counting technology employs various types of detectors. These detectors are sensitive to light and produce an electronic signal when they are struck by a photon. Detectors like the photomultiplier tube (PMT) and avalanche photodiode (APD) are commonly used. A PMT works by amplifying the photon signal through a cascade effect, resulting in significant gain and increased sensitivity. The APD, on the other hand, utilizes the avalanche effect to multiply the electron signal generated by a single photon, thereby offering high gain and speed, albeit with increased noise compared to PMTs.Applications of photon counting extend beyond biology to include astrophysics, where it is used to observe faint astronomical objects, and quantum optics, where it aids in experiments involving quantum entanglement and other phenomena.
Hint: Photon counting is not only about counting photons; it’s about achieving precise and accurate measurements that enhance scientific research and discoveries.
Photon Counting Principle
The photon counting principle is integral to many fields, allowing for the precise measurement of light particles over a given timeframe. This measurement is crucial in experiments requiring high sensitivity and accuracy.
Understanding Photon Counting Mechanism
Photon counting involves capturing and counting individual photons using specialized detectors. These detectors convert the photon energy into an electrical signal, which is then digitized and counted. This process ensures that even low levels of light can be measured accurately, which is particularly beneficial in applications like spectroscopy and astronomy.
One fundamental aspect of photon counting is its exceptional signal-to-noise ratio (SNR). By accurately counting individual photons, photon counting minimizes noise interference. This capability is represented mathematically by the Poisson distribution:
Given an average photon arrival rate \(\bar{n}\), the probability of detecting \(n\) photons in a given time frame is expressed as:
\[ P(n) = \frac{{\bar{n}^n \cdot e^{-\bar{n}}}}{{n!}} \]
Example of Photon Counting in Practice:Consider a telescope observing distant stars. By using photon counting detectors, astronomers can gather data on the time and intensity of light received. This capability allows them to study celestial phenomena, like supernovae, with remarkable precision.
Deep Dive into Photon Counting Applications:The versatility of photon counting extends beyond the immediate scientific fields. For instance, in medical imaging, photon counting is used to improve the resolution and diagnostic capabilities of imaging technologies such as PET scans. Photon counting detectors, such as silicon photomultipliers (SiPMs), offer high resolution and sensitivity. This technology works on the principle of multiplying the signal generated by incoming photons. The equations governing the gain factor in photomultipliers are critical for understanding how amplification improves signal fidelity:
\[ \text{Gain} = \frac{{I_{\text{output}}}}{{I_{\text{input}}}} \]
Where \(I_{\text{output}}\) is the current at the output, and \(I_{\text{input}}\) is the current due to initial photon arrival.
Hint: Photon counting techniques are highly efficient in environments where light levels are extremely low, making them ideal for dark sky observatories.
Photon Counting Technique
The photon counting technique plays a significant role in various scientific and technological applications. Its ability to detect even the smallest amounts of light makes it indispensable for high-precision research and analysis.
Working Mechanism of Photon Counting
Photon counting relies on detecting and counting each individual photon using sensitive detectors, such as photomultiplier tubes (PMTs) and avalanche photodiodes (APDs). These detectors are designed to convert the energy of each photon into an electronic signal, which can then be quantified.
The key steps in the photon counting process include:
- Photon arrival at the detector
- Conversion of photon energy to an electronic signal
- Amplification of the signal
- Counting and recording of the signal
Mathematically, the photon detection can be described by increasing the signal-to-noise ratio (SNR), crucial for accurate measurements:
\[ \text{SNR} = \frac{S}{N} \]
Where \(S\) denotes the signal power, and \(N\) indicates the noise power.
Photon Counting: A highly sensitive technique for measuring the number of photons detected over time, crucial for precise optical measurements.
Example: In medical imaging, particularly PET scans, photon counting enhances the quality of images by ensuring that only true signals from radioactive tracers are counted, reducing background noise and improving diagnostic accuracy.
Deep Dive into Detector Technology: Photon counting systems often use silicon photomultipliers (SiPMs). These detectors are solid-state devices with high gains and are capable of detecting individual photons. SiPMs operate by converting each detected photon into multiple electrons, which are collected to form an electronic signal. The output current \( I_{\text{output}} \) is enhanced by the gain factor of the SiPM, which can be expressed as:
\[ I_{\text{output}} = \text{Gain} \times I_{\text{photon}} \]
Where \( I_{\text{photon}} \) is the current generated by the initial photon interaction. These detectors are key in applications requiring high sensitivity and expediency, such as particle physics and optical communication.
Hint: The fundamental advantage of photon counting is its ability to count single photons, which is crucial when working with low-light levels.
Biological Applications of Photon Counting
Photon counting is a valuable technique in biological sciences, providing insights into cellular processes, molecular interactions, and structural details that are often not visible through conventional methods. By accurately measuring the number of photons emitted or absorbed, researchers can study intricate biological phenomena with high sensitivity.
Examples of Photon Counting in Biology
In the realm of biology, photon counting is pivotal in several applications. Some notable examples include:
- Fluorescence Coincidence Analysis: This technique utilizes photon counting to study the dynamics of fluorescence-tagged molecules in living cells. It helps in understanding the movement and interaction of proteins.
- Single-Molecule Detection: Uses photon counting to observe individual molecules, allowing detailed study of molecular behavior under various conditions.
- Fluorescence Lifetime Imaging Microscopy (FLIM): This method involves measuring the time a fluorescent molecule remains in an excited state before emitting a photon, helping to map biochemical environments within cells.
In these applications, the precision and low noise levels of photon counting provide invaluable data that enhances scientific understanding of biological systems.
Fluorescence Lifetime: The time a molecule retains energy from a photon before emitting it again, crucial for mapping cellular environments.
Example: In single-particle tracking, photon counting enables researchers to follow the movement of a single protein across the cell membrane, revealing its interaction with other cellular structures.
Deep Dive into Fluorescence Lifetime Imaging: FLIM's reliance on photon counting allows differentiation between states of molecules within a cell based on fluorescence lifetime. Using the equation for decay processes:
\[ I(t) = I_0 e^{-t/\tau} \]
Where \( I(t) \) is the intensity at time \( t \), \( I_0 \) is the initial intensity, and \( \tau \) is the fluorescence lifetime. This equation helps in calculating the time-dependent change in fluorescence, providing insights into cellular pH, viscosity, or molecular binding events.
Time Correlated Single Photon Counting
Time Correlated Single Photon Counting (TCSPC) is an advanced photon counting technique used to measure the time interval between the excitation of a sample and the detection of an emitted photon. This method is crucial for gathering detailed temporal data in experiments where timing is essential, such as fluorescence decay studies.
TCSPC exploits the relationship between time and photon emission to provide high-resolution data about molecular interactions and dynamics. The data gathered from TCSPC can be visualized in a time correlation graph to aid interpretation. The underlying principle of TCSPC is mathematically expressed as:
\[ N(t) = N_0 e^{-t/\tau} \]
Where \( N(t) \) is the number of photons detected at time \( t \), \( N_0 \) is the initial photon count, and \( \tau \) is the time constant representing the system or sample's response.
Hint: Photon counting, particularly TCSPC, is integral in time-resolved spectroscopy, providing essential data for analyzing rapid biochemical processes.
photon counting - Key takeaways
- Photon Counting Definition: Measuring the number of photons, light particles, detected over time for accurate scientific experiments.
- Photon Counting Principle: Precise measurement of light particles using detectors for high sensitivity and accuracy, crucial in various scientific fields.
- Time Correlated Single Photon Counting (TCSPC): An advanced technique measuring the time between photon emission and detection, useful in fluorescence decay studies.
- Biological Applications of Photon Counting: Important for fluorescent microscopy, bioluminescence imaging, DNA sequencing, and more, offering sensitivity and precision.
- Examples of Photon Counting in Biology: Used in fluorescence coincidence analysis, single-molecule detection, and fluorescence lifetime imaging microscopy (FLIM).
- Photon Counting Technique: Involves photon detection and counting via sensitive detectors, beneficial for accurate scientific research.
Learn faster with the 12 flashcards about photon counting
Sign up for free to gain access to all our flashcards.
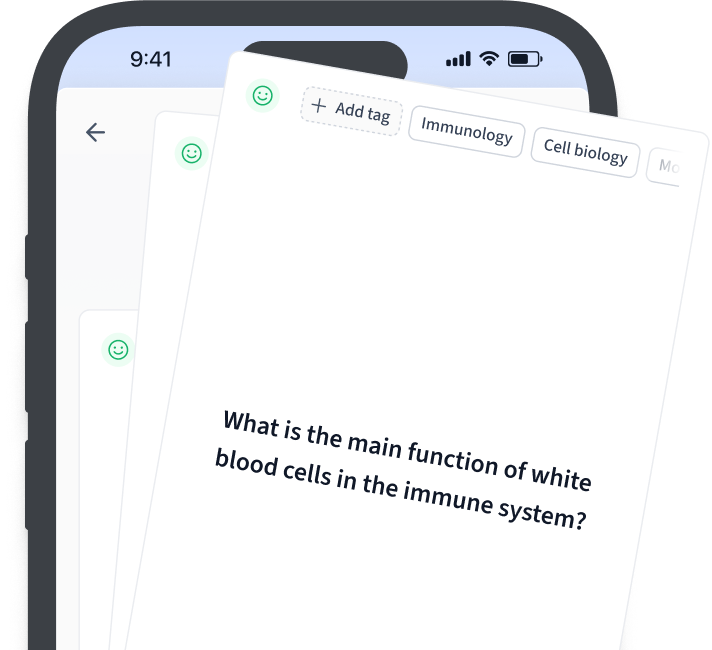
Frequently Asked Questions about photon counting
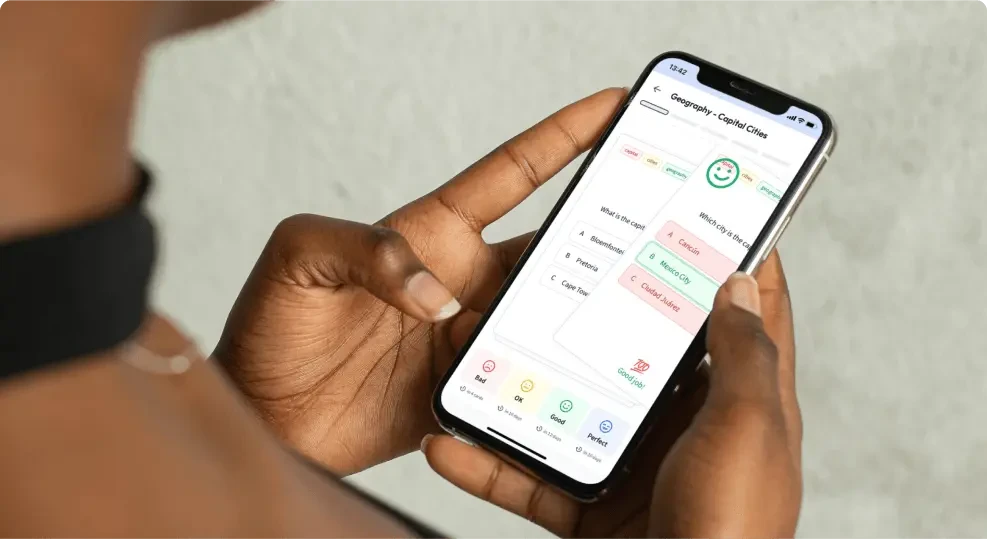
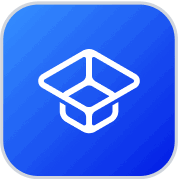
About StudySmarter
StudySmarter is a globally recognized educational technology company, offering a holistic learning platform designed for students of all ages and educational levels. Our platform provides learning support for a wide range of subjects, including STEM, Social Sciences, and Languages and also helps students to successfully master various tests and exams worldwide, such as GCSE, A Level, SAT, ACT, Abitur, and more. We offer an extensive library of learning materials, including interactive flashcards, comprehensive textbook solutions, and detailed explanations. The cutting-edge technology and tools we provide help students create their own learning materials. StudySmarter’s content is not only expert-verified but also regularly updated to ensure accuracy and relevance.
Learn more