Jump to a key chapter
Anomer Definition and Its Significance in Organic Chemistry
Chemistry, specifically organic chemistry, is a field of study brimming with numerous concepts and terminologies. One such terminology that you may encounter is 'anomer'. You might be wondering, what is an anomer? You'll soon understand why this term is crucial in the vast world of organic chemistry.
Basic Anomer Definition
Anomer refers to each of two isomers of a glycoside or a saccharide whose molecules differ only in the configuration about the hemiacetal or hemiketal carbon atom. This carbon atom is often referred to as the anomeric carbon.
On a more straightforward note, anomers are carbohydrates that differ at the new stereogenic center formed on ring closure.
- α-anomers: If the hydroxyl group is below the plane of the sugar ring, it is an alpha anomer. It's denoted as \( \alpha \).
- β-anomers: If the hydroxyl group is above the plane of the sugar ring, it is a beta anomer. It's denoted as \( \beta \).
Imagine a flat ring; anything 'below' is meant to be sticking out of the back of the ring, while 'above' means sticking out from the front.
Importance of Anomer in Organic Chemistry
Now you have basic knowledge of what anomer is, you might be curious about why it is significant. You see, understanding anomers is fundamental in the study, particularly in the structures and functions of biologically important molecules, like nucleotides and carbohydrates. Moreover, the anomer's concept is also necessary when learning about reactions and mechanisms involving carbohydrates.
Role in Carbohydrates | Anomers play an essential role in determining the structure and stereochemistry of carbohydrates, which are crucial components of many biological molecules. |
Role in Reactions | The concept of anomerism is pivotal to understand mutarotation, a characteristic reaction of cyclic hemiacetals and hemiketals, which are the types of compounds carbohydrates generally are. |
For instance, when glucose is dissolved in water, it exists in two forms: alpha-glucose and beta-glucose. These two forms continually interconvert in a process known as mutarotation. If you know about anomers, it will be easy to understand this process and the optical activity of glucose solution!
Anomers undoubtedly play a vital role in organic chemistry, offering a dynamic outlook on how even such small changes can influence the whole structure and function of significant biological molecules. That's why it's so crucial not just to memorise their definitions, but to really understand what they are and why they matter.
Practical Anomer Examples in Chemistry
Anomer understanding isn't just academic; it also has practical applications in everyday life and the field of health and medicine. Let's explore some of these real-world instances where the concept of anomers comes into action.
Anomer Example in Everyday Chemistry
One can find remarkable instances of anomers in the food you consume. Consider lactose, the sugar present in milk. This compound consists of glucose and galactose units linked by a glycosidic bond, a type of chemical bond that joins a carbohydrate molecule to another group, which may or may not be another carbohydrate. Now, the glucose here can exist either in its alpha or beta form – essentially, they are anomers of each other.
The interesting part is that the nature of this glucose anomer in lactose affects many people's digestive systems. Certain individuals are unable to digest lactose when the glucose is in its beta form, a condition know as lactose intolerance. It is a clear indication of how the alpha or beta configuration of glucose (anomers) can significantly impact biological processes.
Anomer in Glucose: An Important Example
Glucose is the classic example used to explain anomers. So, delve into a more detailed appreciation of this instance. A glucose molecule has an aldehyde functional group (\( \textrm{CHO} \)) and multiple hydroxyl groups (\( \textrm{OH} \)). In its straight-chain form called aldohexose, one of the hydroxyl groups can react with the aldehyde group to form a hemiacetal. This interconversion between straight-chain and ring form is known as ring-closure.
Upon ring closure, a new chiral centre is created at the carbon atom that was part of the aldehyde group. This new chiral centre is termed as the anomeric carbon, and its configuration determines whether the anomer formed is alpha or beta. If the \( \textrm{OH} \) group at the anomeric carbon points down (or to the right in Fisher projection), it is the alpha anomer, also known as α-glucopyranose. Conversely, if the \( \textrm{OH} \) group points up (or to the left in Fisher projection), the anomer is the beta form, referred to as β-glucopyranose.
For a more exciting exploration of this phenomenon, consider the sweetener Splenda. It is a chlorinated sugar where three (OH) groups are replaced by three (Cl) atoms. This synthetic compound cannot be broken down by the body, so it passes through without increasing calorie intake. The success of Splenda lies in its 'anomeric trick' – its beta form provides the sweet taste, while the molecule's overall shape discourages the body from breaking it down.
A noteworthy aspect of glucose and many other sugars is that they can spontaneously flip between their alpha and beta forms when dissolved in water, a process known as mutarotation. This action is, in fact, a demonstration of anomerization, the interconversion between anomers.
Appreciating the concept of anomers and its implementation helps in understanding not only organic chemistry and biochemistry but also the onset of various health conditions and the structural principles of synthetic compounds.
Anomer vs Epimer: Understanding the Difference
In your exploration of organic chemistry, you will encounter a myriad of terms, and two such terms that you might find intriguing and perhaps confusing are "anomers" and "epimers". While both are related to the isomerism, they represent different aspects of it in molecular structure.
Defining Epimers in Relation to Anomers
What are epimers? Like anomers, epimers are a type of stereoisomer, but the distinguishing factor differs. Epimers are diastereomers that differ in configuration at only one chiral carbon other than the anomeric carbon. This definition would indicate that all anomers are epimers, but not all epimers are anomers.
To simplify, imagine you have a molecule with several chiral carbons. If you switch the position of two groups around just one of these carbons (besides the anomeric carbon), but leave everything else in the molecule the same, the resulting molecule is an epimer of the first.
However, beware that there's an exception to this rule: the anomeric carbon. If two molecules differ in configuration solely at the anomeric carbon, they are anomers, not epimers. If they are different at the anomeric carbon and at least one other chiral carbon, then they are epimers and also anomers. This subtlety is what causes much of the confusion between these terms.
Clear Examples: Anomer vs Epimer
It's time now to clarify the differences between anomers and epimers with some clear examples. As you know well by now, chemists often turn to carbohydrates to illustrate these concepts, given their wealth of chiral carbons.
- Anomer Example: An anomeric example comes from glucose. The alpha and beta forms of glucose are anomers of each other. They differ only at the anomeric carbon.
- Epimer Example: An example of an epimer will be glucose and mannose. If you take a look at their structures, you can see that they are identical except for the configuration around carbon 2. This single difference is the hallmark of an epimer.
These examples illustrate the real crux of the difference between the two terms:
Anomers: | Consider only the anomeric carbon and are a special case of epimer. |
Epimers: | This broader category includes any molecules that differ at a single carbon excluding the anomeric carbon. |
This scrutiny into the details of molecular configuration brings foundational understanding to the study of organic chemistry and biochemistry. By appreciating the slight adjustments at each carbon centre, it gives an insight into how each molecule behaves and responds - thereby shaping the overall reactions and biological processes that drive life as we know it.
Effect of Anomer on Chemical Properties
Understanding the effect of anomers on chemical properties is an integral part of organic chemistry, particularly when examining the properties of carbohydrates, such as sugars. The configuration of the anomeric carbon – whether in an alpha or beta state – can significantly impact the molecule's chemical reactivity and potential interactions.
Analysing the Anomer effect on properties
To grasp the effect of anomers on chemical properties, let's delve into the world of monosaccharides, the simplest form of carbohydrates. You're likely familiar with the concept of alpha and beta anomers. These isoforms differ at the anomeric carbon, an idea that extends back to a molecule's cyclisation pattern.
- The \( \alpha \)-anomer has the \( \text{OH} \) (hydroxyl) group on the anomeric carbon in an axial position, meaning it points down.
- The \( \beta \)-anomer, conversely, has the \( \text{OH} \) group on the anomeric carbon in an equatorial position or pointing up.
It might seem like a minor alteration, but this twist at the anomeric carbon can significantly alter intermolecular interactions, sugar stability, and chemical reactivity.
Take the example of glucose. The beta form of glucose is more stable than the alpha form. The primary reason is that the axial position of the \( \text{OH} \) in alpha-glucose creates more steric hindrance – essentially, molecular crowding – which destabilises the molecule. On the other hand, the equatorial position of the \( \text{OH} \) in beta-glucose minimises steric strain, diaxial interactions, and results in more stable chair conformation.
Furthermore, the different positions of the hydroxyl group in the anomers also affect their intermolecular interactions, such as hydrogen bonding. Beta-glucose, for instance, can form intermolecular hydrogen bonds more effectively than its alpha counterpart. This increased capability plays an integral role in enhancing the solubility of beta-glucose in water.
The Impact of Anomeric Configurations on Chemical Behaviour
Digging even deeper, anomeric configurations not only affect the physical properties of molecules but also their chemical behaviour. In particular, the alpha and beta configurations influence the reactivity of the anomeric carbon. This property is vital when considering reaction mechanisms involving glycosidic linkage formation or breakage, as seen in condensation reactions or hydrolysis.
To illustrate this point, let's revisit the formation of the disaccharide lactose from glucose and galactose. During this process, an \( \text{OH} \) group on the glucose reacts with an \( \text{OH} \) group on the galactose to eliminate a water molecule and form a glycosidic bond.
A glycosidic bond is a covalent bond that holds a carbohydrate (glycoside) to another group.
Note the essential role of our friend, the anomeric carbon, in this reaction. As a hemiacetal (in monosaccharides) or hemiketal (in disaccharides) carbon, it can react with an alcohol to form an acetal or ketal, becoming a glycosidic linkage in the process. Moreover, the orientation of this hemiacetal or hemiketal at the anomeric carbon determines whether the resulting glycosidic bond is in the alpha or beta configuration. These different configurations result in distinct disaccharides with distinct biological functions.
Furthermore, in glycoproteins and glycolipids, which are key players in cell-cell recognition process, the exact position and orientation of the monosaccharide units bonded via glycosidic bonds plays a crucial role. Hence, anomeric configurations reflecting in their chemical behaviours are of considerable biological significance.
Emphasising these effects on a molecular level, the study of anomers unravels the fascinating complexity and specificity characterising chemical and biological systems. As you continue to explore the thrilling world of organic chemistry, bear in mind the versatility and influence these different configurations bring to the table, shaping the structures, reactivities and ultimate functions of the molecules we encounter.
Anomer in the Context of Cyclic Structures
Anomer is a vital term, especially within the domain of cyclic structures. Organic molecules, such as carbohydrates, often exist in cyclic forms, and this is where anomers come into play. However, the concept of anomers is not limited to carbohydrates; it extends to any cyclic molecule that involves a hemiacetal or hemiketal group.
Role of Anomer in Cyclic Structures
If you are interested in understanding how certain organic molecules behave, particularly when they are in their cyclic forms, then you will need to familiarise yourself with anomers.
So what exactly are anomers? Well, in cyclic structures such as carbohydrates, an anomer is a type of stereoisomer, or to be specific, a type of diastereomer. In carbohydrate chemistry, anomers are distinct forms of a sugar that differ only in their configuration around the \( \text{hemiacetal} \) or \( \text{hemiketal} \) carbon. This carbon is commonly referred to as the anomeric carbon.
The arrangement of the substituent at this anomeric carbon determines whether the carbohydrate is in an alpha or beta form. In the alpha anomer, the \( \text{OH} \) group at the anomeric carbon is positioned trans to the CH_2OH group, the so-called anomeric reference group. In the beta anomer, the \( \text{OH} \) group is cis to the CH_2OH group. As simple as this distinction may seem, it greatly influences the behaviour and characteristics of the molecule.
Molecules that can switch between the alpha and beta configurations can undergo a process called mutarotation, altering their physical properties like the specific rotation. Sometimes this change can affect the taste of a sugar, its fermentation capacity, and other properties, making the study of anomeric configurations essential in food and fermentation industries.
How does an Anomer Form in Cyclic Structures?
When a molecule forms a cyclic structure, it entails one of the molecule's hydroxyl groups reacting with an aldehyde or ketone group present within the same molecule. As a result, these functional groups transform into a \( \text{hemiacetal} \) or \( \text{hemiketal} \), and the carbon atom of this newly formed functional group is our focus of interest - the anomeric carbon.
During the cyclisation process, the former carbonyl carbon (an aldehyde or ketone) transforms into a new chiral centre, the anomeric carbon, leading to the creation of two distinct configurations, alpha and beta. This is a significant step known as anomerisation.
What prompts these configurations? It comes down to the spatial orientation of the substituent on the anomeric carbon - the \( \text{OH} \) group. The molecule can undergo a conformational change – a rotation about the bond between the oxygen atom and the anomeric carbon – causing this hydrogen atom to swing from a position on one side of the plane of the ring (axial position) to a position on the other side (equatorial position).
An axial position: the group extends approximately perpendicular to the plane of the ring.
An equatorial position: the group extends roughly along the plane of the ring.
Take the example of glucose: Glucose can cyclise into a six-membered ring (a pyranose structure). During the process, the aldehyde at carbon 1 (C1) can react with the \( \text{OH} \) group at C5. This forms a cyclic hemiacetal, and at this juncture, there's a development of an additional chiral centre at C1, the anomeric carbon. Depending on the final position of the \( \text{OH} \) group, we get either alpha or beta glucose.
So next time, when you encounter a cyclic structure, remember that it might have an alpha or a beta anomer, depending on the orientation of the group attached to the anomeric carbon. Consider the profound effects these arrangements can have on the properties of the molecule, an understanding that we owe to the subtlety of anomers.
Anomer - Key takeaways
- Anomer definition: An anomer is a type of diastereomer, specifically in the context of cyclic structures such as carbohydrates. Anomers are distinct forms of a sugar that differ only in their configuration around the hemiacetal or hemiketal carbon, which is referred to as the anomeric carbon.
- Anomer example: An instance of anomer is seen in lactose, a sugar present in milk, composed of glucose and galactose units. Here, glucose can exist in either its alpha or beta form – these are essentially anomers of each other. Another classic example is the alpha and beta forms of glucose.
- Anomer vs epimer: While both anomers and epimers are related to the concept of isomerism, they represent different aspects in molecular structure. Anomers only consider the anomeric carbon whereas epimers include any molecules that differ at a single carbon excluding the anomeric carbon. All anomers are epimers, but not all epimers are anomers.
- Anomer effect on properties: The configuration of the anomeric carbon can significantly impact the chemical reactivity and potential interactions of the molecule. This affects intermolecular interactions, stability of sugar, and chemical reactivity.
- Anomer in cyclic structures: The concept of anomer extends to any cyclic molecule involving a hemiacetal or hemiketal group. The orientation of the substituent at the anomeric carbon determines whether the carbohydrate is in an alpha or beta form, greatly influencing the molecule’s behaviour and characteristics.
Learn faster with the 15 flashcards about Anomer
Sign up for free to gain access to all our flashcards.
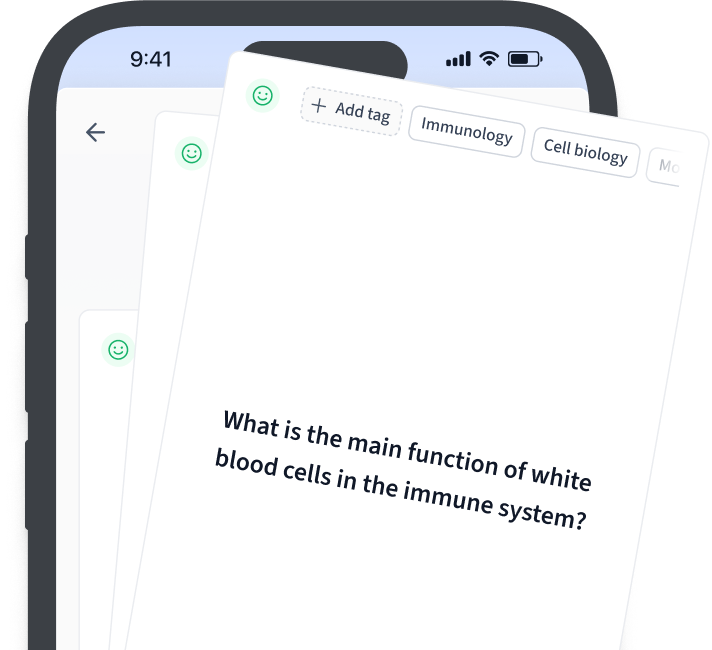
Frequently Asked Questions about Anomer
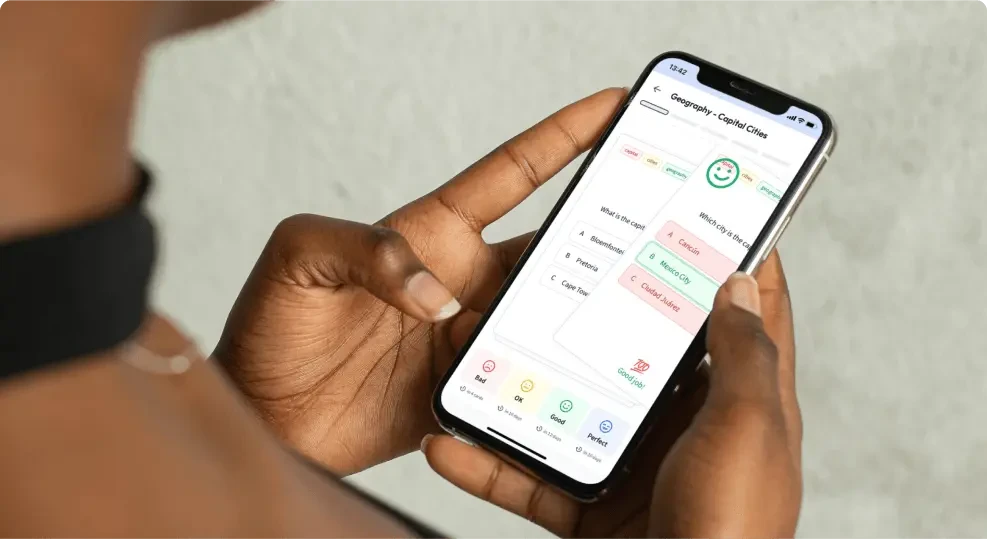
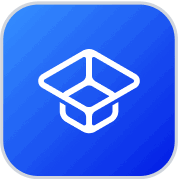
About StudySmarter
StudySmarter is a globally recognized educational technology company, offering a holistic learning platform designed for students of all ages and educational levels. Our platform provides learning support for a wide range of subjects, including STEM, Social Sciences, and Languages and also helps students to successfully master various tests and exams worldwide, such as GCSE, A Level, SAT, ACT, Abitur, and more. We offer an extensive library of learning materials, including interactive flashcards, comprehensive textbook solutions, and detailed explanations. The cutting-edge technology and tools we provide help students create their own learning materials. StudySmarter’s content is not only expert-verified but also regularly updated to ensure accuracy and relevance.
Learn more