Jump to a key chapter
Introduction to Chiral Pool
Learning about the chiral pool is like embarking on an essential journey into the depths of organic chemistry. Essentially, the chiral pool refers to a set of readily available and naturally occurring compounds that possess chiral centers and can be utilized as starting materials in the synthesis of more complex chiral entities. Mostly, these are amino acids, sugars, and terpenes.Understanding the Chiral Pool Definition
In organic chemistry, the term 'chiral pool' refers to a group of readily available, enantiomerically pure substances from natural resources that can be utilized in organic synthesis. Chirality is a fundamental concept in organic chemistry dealing with the geometry of molecules. A molecule is chiral if it cannot be superimposed onto its mirror image, just like your left and right hands.
C /|\ H R XThe chirality of a molecule impacts its physical and chemical properties, especially its interaction with plane-polarized light and other chiral compounds.
For example, a common chiral substance is the amino acid, L-Arginine, used frequently in the chiral pool. Other examples include sugars like D-Glucose and D-Ribose as well as terpenes like (-)-Menthol.
Importance of Chiral Pool in Organic Chemistry
The chiral pool is vital in the field of organic chemistry for several reasons:- It provides a starting point for the synthesis of complex chiral substances.
- It allows the production of enantiomerically pure substances, an important aspect in pharmaceuticals.
- It bridges the gap between theoretical study and practical application.
Difference | Chiral Pool Synthesis | Synthesis without Chiral Pool |
Waste Production | Minimal | High |
Cost Effectiveness | High | Low |
Time Factor | Less time-consuming | More time-consuming |
Did you know? Synthesising substances via the Chiral Pool often involves the use of enzymes, which are also chiral in nature. These enzymes can selectively catalyze reactions on one enantiomer over the other, leading to enantioselective processes. This exemplifies the integration of biology and chemistry, another fascinating aspect of the chiral pool.
Delving into Chiral Pool Synthesis
Diving deeper into chiral pool synthesis reveals a world of complexity, precision and beauty, representative of the wonders of organic chemistry. By harnessing the natural chirality found in abundant compounds, chiral pool synthesis provides an ideal route for obtaining enantiomerically pure substances, which is often a necessity in the development of pharmaceutical treatments, aroma compounds, and more.Chiral Pool in Asymmetric Synthesis: An Overview
The use of the chiral pool in asymmetric synthesis signifies an interesting aspect of organic chemistry. Asymmetric synthesis is essentially a method that results in a preferential formation of one enantiomer over the other. This is immensely useful given that different enantiomers of a compound can vary greatly in their biological activity. In the context of chiral pool synthesis, the term 'asymmetric' denotes the synthesis processes that maintain or enhance the enantiomeric purity of the starting materials from the chiral pool. When synthesising using the chiral pool, emphasis is placed on maintaining the integrity of the chiral centres, with any manipulations being carefully conducted to prevent racemisation - a process which would lead to a mixture of enantiomers rather than a single, pure one. This is often achieved by choosing the right reaction conditions and reagents. Some important aspects of a successful chiral pool synthesis involve:- Ensuring the choice of suitable starting material from the chiral pool.
- Preserving or enhancing the enantiomer excess ie., the difference in amounts of \( R \) and \( S \) enantiomers.
- Avoiding racemisation through careful selection of reaction conditions.
Key Steps in Chiral Pool Synthesis
Breaking down the process, there are certain key steps typically followed in a chiral pool synthesis:- Selection of the Starting Material: The choice of starting material is crucial and is usually an enantiomerically pure substance from the chiral pool. The chosen compound usually features structural similarity or a convenient synthetic route to the target molecule.
- Protecting Group Strategies: Protecting the functional groups is often an important step in chiral pool synthesis. Protecting groups guard sensitive functional groups to prevent them from reacting prematurely. The choice of protecting group depends on its compatibility with the rest of the molecule and the subsequent reaction conditions.
- Synthetic Manipulation: The sequence of reactions used to convert the starting material to the target compound forms the core of chiral pool synthesis. These reactions aim to install new functional groups, build complexity or alter the stereochemistry as required. This step requires careful planning and execution as the reaction conditions should not lead to racemisation.
- Deprotection and Purification: In the final steps, protecting groups are removed, and the desired product is purified. Depending on the complexity of the target compound, several rounds of protection, synthesis, deprotection and purification may be needed.
The Role of Chiral Pool Molecules
Chiral pool molecules serve crucial roles within the realm of chemistry, quite notably in the preparation of complex chiral substances. More than just a collection of chemical starting materials, these molecules embody the beauty and intricate nature of organic chemistry, showcasing the versatility of nature's own resources. These enantiomerically pure molecules, which form the chiral pool provide a starting point for designing creative and less wasteful pathways to synthesise complex, chiral compounds. These naturally occurring molecules encompass a wide range of structures and functionalities, with everything from carbohydrates, amino acids, to terpenes, making the process of synthesis flexible and diverse. Through the manipulation of bonds, the use of protection and deprotection strategies, and the careful selection of reagents, a molecular transformation is possible, with countless variations and combinations available to achieve the desired chiral product.Notable Examples of Chiral Pool Molecules in Organic Chemistry
One of the many fascinating aspects of chiral pool molecules in organic chemistry is their sheer diversity. There are numerous examples of molecules that form part of the chiral pool, each with its unique structural characteristics and properties. Being derived from natural sources, these molecules come from various biological molecules that exhibit chirality. One of the prominent examples of such molecules includes D-Glucose, an enantiomerically pure sugar. D-Glucose provides an abundance of chiral centres and is often used in the creation of complex chiral molecules. Showing five chiral carbons, D-Glucose offers a multiplicity of stereoisomers, presenting chemists with fertile ground for synthesis.For instance, the list below illustrates the structure and application of D-Glucose:
- Structural Formula: HOCH2(CHOH)4CH2OH
- Application: It's used in medicine, food industry, and in the synthesis of high-value chiral building blocks.
The characteristics of L-Alanine include:
- Structural Formula: CH3CH(NH2)COOH
- Application: In proteins production, and a key molecule in metabolism.
How Chiral Pool Molecules Interact in Chemical Reactions
Chiral pool molecules, given their inherently chiral nature, play a significant role in chemical reactions, particularly in the field of organic synthesis. The key underlying concept guiding all interactions in chemical reactions involving chiral pool molecules is the fundamental principle of stereoselectivity. Essentially, stereoselectivity is a property of a chemical reaction wherein a single reactant forms an unequal mixture of stereoisomers during the non-catalytic creation or transformation of a chemical bond. If the reaction under consideration involves a chiral pool molecule as the reactant or catalyst, the selectivity can greatly swing towards one stereoisomer, leading to a preferential formation of one enantiomer over the other—a concept termed "enantioselectivity". Typically, chiral pool molecules are involved in reactions where the goal is to generate new stereocentres with a high degree of control over the stereochemical outcome. These molecules, due to their inherent chirality, can provide a good sense of direction to reactions and help dictate the stereochemical course of the reaction. An enantiomerically pure molecule from the chiral pool can interact with achiral or racemic reactants to form new chiral centres. If these reactions are accomplished under thermodynamic or kinetic control, the new chiral centres are generated with preferential orientation - a process known as asymmetric induction. For reactions involving chiral pool molecules, careful consideration is given to avoid any undesired reactions that could lead to a loss of stereochemical integrity. For instance, certain reagents or reaction conditions might cause racemisation, where the chiral centre is lost due to the equal formation of both enantiomers.Racemisation is a process in chemistry where the (R)- and (S)- enantiomers of a chiral molecule are formed in equal amounts, leading to a racemic mixture.
Utilising Chiral Pool Reagents
In the marvels of chemistry, you'll find the chiral pool, an abundant resource of enantiomerically pure reagents that await the creative touch of chemists. These reagents are perfect starting points for synthesising complex chiral products. They can be manipulated, bonded, blended, and synthesised in countless ways to yield the desired product. Working with chiral pool reagents, therefore, requires considerable understanding and knowledge about their properties and how those properties influence their potential applications.Signature Properties of Chiral Pool Reagents
Chiral pool reagents, featuring unique properties that steer how they interact and profile within any given chemical reaction, are indispensable tools in the field of organic synthesis. These distinctive properties are largely focused around their state of chirality, a pivotal quality that manipulates their interactions with other chemical substances, their geometrical disposition in space, and ultimately determines their bioactivity.Chirality: This term describes how the structure of a molecule cannot be superimposed onto its mirror image. This property is typically apparent in molecules featuring a carbon atom attached to four distinct substituents, resulting in different spatial arrangements of atoms and hence different enantiomers.
- Stereochemical Integrity: Preservation of the chiral centre(s) plays a significant role in reactions, avoiding undesired side reactions and racemisation.
- Reactivity: The type of reactions these reagents can experience or trigger, which is crucial while selecting for specific step of the synthesis pathway.
- Availability: This property lies in the domain of practicality, given the requirement for these substances to be abundantly available for experimental setup.
Techniques for Implementing Chiral Pool Reagents
Implementing chiral pool reagents in chemical synthesis involve some precise techniques. Being well-versed with these methods and practices can open the door to efficient and effective synthesis of chiral products. A primary step in this method is the selection of starting material. Aided by the structural requirements of the target molecule, you choose a starting material from the chiral pool that closely resembles it, or on the other hand, that can be conveniently transformed into the desired product. It's an ideal scenario when the selected molecule itself is a substance readily available in large quantities (for instance, natural amino acids and sugars).Scenarios where these biomolecules serve as starting points considerably shorten the reaction pathway and reduce the number of steps required for the synthesis of the target molecule.
Protecting Group: A protecting group is a functional group that is introduced into a molecule by chemical modification to shield a reactive site during a specific reaction, preventing it from reacting prematurely. They are typically opted for when functional groups are incompatible with required reaction conditions or will interfere with a given chemical transformation.
Unpacking the Chiral Pool Technique
Exploring the technique of chiral pool synthesis provides a far-reaching perspective on how complex chiral molecules can be effectively and efficiently synthesised in the lab. This idea revolves around using readily available chiral molecules from nature (the 'chiral pool') as starting points for synthesis, instead of building chiral molecules from achiral substances—a process that often involves complex lab techniques and expensive catalysts.Developing Skills for the Chiral Pool Technique
Understanding and applying the chiral pool technique requires the development of specific skills and knowledge. A strong grasp of organic chemistry concepts, such as functional groups, reactions and their mechanisms, molecular structures, and chirality, is fundamental. In addition to this, understanding the principles of stereochemistry is essential, as maintaining the stereochemistry of the starting material throughout the synthesis is often a crucial concern in chiral pool synthesis.Stereochemistry: This branch of chemistry is concerned with the three-dimensional arrangement of atoms and molecules and the impact of this on chemical reactions.
- Understanding Chirality and Stereochemistry: Understanding in-depth the concept of chirality and the nuances of stereochemistry is essential to predict the end product's stereospecific outcome.
- Familiarity with Protecting Groups: Familiarity with protecting group strategies is beneficial in choosing the path of the reaction, as these influence the stereochemical outcome.
- Predicting Reactions: Being able to predict reaction outcomes based on the starting material structure and the reagents used is vital.
- Knowledge of Analytical Techniques: Proficiency in analytical techniques, such as spectral analysis, can help confirm the structure and stereochemistry of the synthesized product.
Example of Chiral Pool Synthesis in Practice
A practical example can best illustrate the concepts discussed above. Suppose the synthesis of the chiral molecule L-phenylalanine, an amino acid. L-phenylalanine can be synthesised using L-shikimic acid, a substance readily available from the chiral pool, as the starting material. The synthesis involves the following steps: 1. Advanced conversion of L-shikimic acid into the intermediate compound phenylpyruvic acid. This process involves protecting the alcohol group in L-shikimic acid using a suitable protecting group\(\) and subsequent dehydrogenation. 2. The phenylpyruvic acid is then converted to L-phenylalanine by a process called transamination. This reaction can be represented as: \[ \text{{L-shikimic acid}} \xrightarrow{{\text{{protecting}} \to \text{{dehydration}}}} \text{{phenylpyruvic acid}} \xrightarrow{{\text{{transamination}}}} \text{{L-phenylalanine}} \]
Reaction1 = {'Starting Material': 'L-shikimic acid ', 'Final Product' : 'phenylpyruvic acid'}
Reaction2 = {'Starting Material': 'phenylpyruvic acid', 'Final Product' : 'L-phenylalanine'}
This example demonstrates the simplicity and efficiency of chiral pool synthesis. The entire process is guided by the careful selection of starting material, strategic placement of protecting groups, and the wise choice of reactants. Additionally, it resonates with the benefits of chiral pool synthesis, such as fewer reaction steps, relatively high yield, and cost-effectiveness.
Remember, while this example is straightforward, real-world applications of chiral pool chemistry often involve complex molecules and multistep reactions. Each reaction is a puzzle that challenges your understanding of organic chemistry. So, dive deep, stay curious, and keep solving!Chiral Pool - Key takeaways
- Chiral pool synthesis involves the use of enantiomerically pure starting materials drawn from a 'chiral pool' of naturally occurring compounds. The aim is to maintain or enhance enantiomeric purity during synthesis, avoiding racemisation by choosing suitable reaction conditions and reagents.
- A successful chiral pool synthesis strategy includes the careful selection of the starting material, preserving/enhancing enantiomer excess, and avoiding racemisation by carefully selecting reaction conditions.
- Chiral pool molecules are enantiomerically pure and can be used to create complex chiral substances. These naturally occurring substances range from amino acids to carbohydrates, and their manipulation allows for a diverse range of synthesis possibilities.
- Key examples of chiral pool molecules include D-Glucose and L-Alanine. These molecules are often used in the synthesis of complex chiral molecules due to their enantiomerically pure nature and abundance of chiral centres.
- Chiral pool reagents are enantiomerically pure substances used in chiral pool synthesis. Their unique properties, like stereochemical integrity and reactivity, make them valuable tools in organic synthesis. Characteristics like availability and chirality are considered when synthesising with chiral pool reagents.
Learn faster with the 15 flashcards about Chiral Pool
Sign up for free to gain access to all our flashcards.
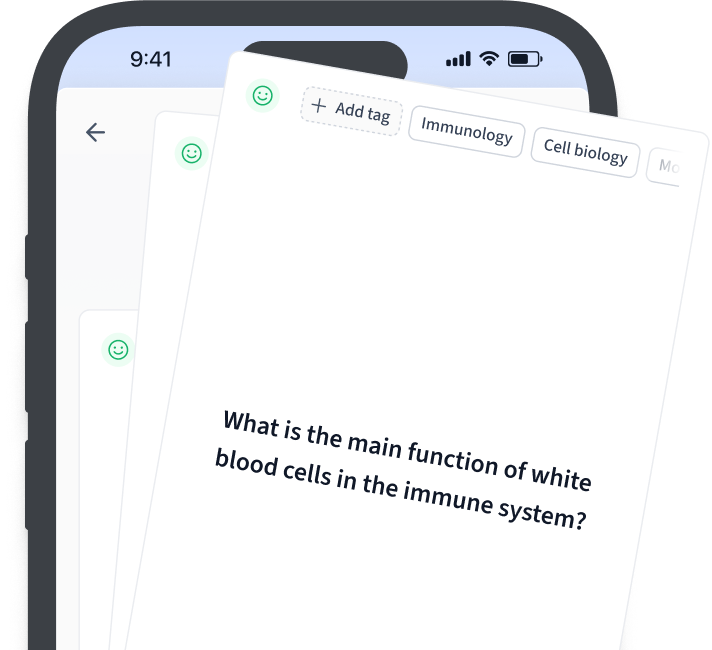
Frequently Asked Questions about Chiral Pool
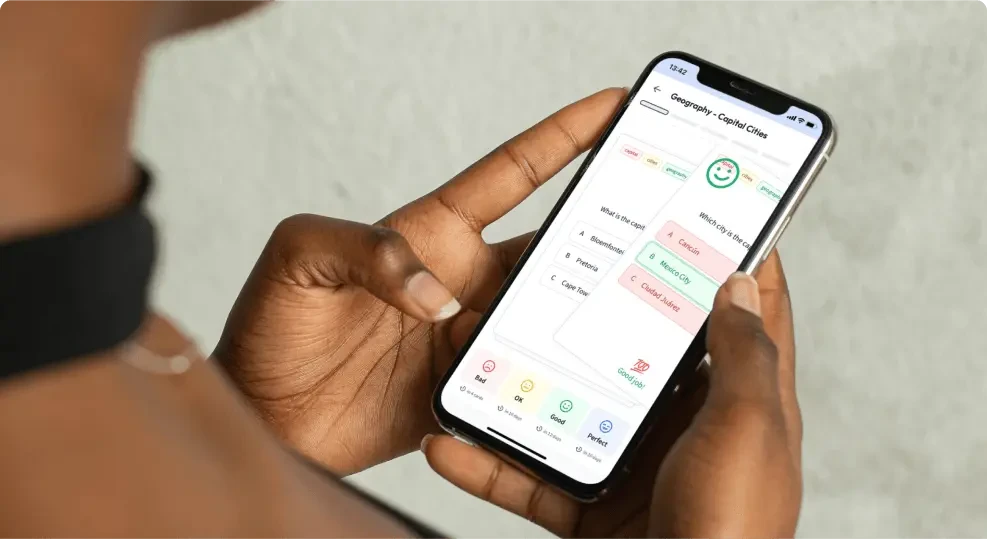
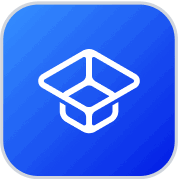
About StudySmarter
StudySmarter is a globally recognized educational technology company, offering a holistic learning platform designed for students of all ages and educational levels. Our platform provides learning support for a wide range of subjects, including STEM, Social Sciences, and Languages and also helps students to successfully master various tests and exams worldwide, such as GCSE, A Level, SAT, ACT, Abitur, and more. We offer an extensive library of learning materials, including interactive flashcards, comprehensive textbook solutions, and detailed explanations. The cutting-edge technology and tools we provide help students create their own learning materials. StudySmarter’s content is not only expert-verified but also regularly updated to ensure accuracy and relevance.
Learn more