Jump to a key chapter
What are Electrocyclic Reactions?
An electrocyclic reaction represents an elementary cornerstone in organic chemistry. Essentially, it is a type of molecular rearrangement where the breaking and formation of sigma bonds result in a cyclic compound. This fascinating reaction is largely powered by the electrostatic forces present among the atomic components of the given molecule.
Basic Definition of Electrocyclic Reactions
At its core, an electrocyclic reaction is a concerted process, meaning it occurs in one step without intermediates. It evolves through a cyclic transition state, leading to the formation or breaking of a pi bond and concomitant formation or breaking of a sigma bond.
An electrocyclic reaction can occur in two possible modes: conrotatory and disrotatory.
- In the conrotatory mode, the terminal ends of the conjugated system rotate in the same direction!
- Conversely, in the disrotatory mode, the terminal ends rotate in opposite directions.
The direction of rotation is governed by the Hückel's rule, and can be predicted using the Möbius–Hückel treatment for electrocyclic reactions.
The Möbius–Hückel concept categorises systems as being Hückel if they possess \(4n+2\) π electrons, and Möbius if they possess \(4n\) π electrons (where \(n\) is any whole number).
Importance in the World of Organic Chemistry
Electrocyclic reactions hold a remarkable significance in the field of organic chemistry due to their ability to facilitate molecule transformations in a unique and effective manner. Using these reactions, you can convert a simple molecule to a significantly more complex structure efficiently and without creating waste products.
For instance, the synthesis of Vitamin D from dehydrocholesterol is a compelling example of a natural electrocyclic reaction.
Vitamin D Synthesis | Dehydrocholesterol to Vitamin D |
Electrocyclic Reaction | Natural |
Moreover, the principles of electrocyclic reactions provide a solid foundation upon which you can understand and interpret other pericyclic reactions, including cycloadditions and sigmatropic reactions.
Pericyclic reactions refer to the class of concerted reactions that include electrocyclic reactions, cycloadditions, and sigmatropic rearrangements.
Overall, electrocyclic reactions provide an indispensable tool both for the laboratory preparation of many complex organic molecules and also for understanding the synthetic pathways used by Nature.
Understanding Conrotatory and Disrotatory Electrocyclic Reactions
To fully grasp electrocyclic reactions, you need to get to grips with their unique terminologies and characteristics, centring mainly on the terms conrotatory and disrotatory. These elective terms actually depict the mechanism of ring closure or ring opening, and subsequently the stereochemistry of the products formed.
Conrotatory Electrocyclic Reactions Explained
A Conrotatory ring closure or opening describes a mechanism where the p orbitals at the ends of the system rotate in the same direction. Similarly, if the ends rotate clockwise, the reaction will push electrons in the clockwise direction along the molecule. As a result, the substituents on the ends of the molecule that were previously cis to each other become trans in the product, and vice versa.
The Conrotatory mode of electrocyclic reaction is most commonly observed in thermal reactions with an odd number of electron pairs in the system. For instance, in thermal reactions, the 4n+2 electron systems undergo Conrotatory ring closures.
Here is a bit more detail about the rule indicating whether a reaction will occur in the Conrotatory mode:
Number of pairs of electrons | Thermal conditions | Photochemical conditions |
Odd | Conrotatory | Disrotatory |
Even | Disrotatory | Conrotatory |
Practical Examples of Conrotatory Reactions
A prime example of a Conrotatory process is the electrocyclic ring closure of butadiene to form cyclobutene. This occurs under thermal conditions and involves four pi electrons, following the rule outlined previously.
During the closure, the terminal carbon atoms rotate in the same direction, making it a Conrotatory reaction.
Disrotatory Electrocyclic Reactions Explained
Contrasting with Conrotatory reactions are Disrotatory reactions. In these reactions, the p orbitals at the ends of the system rotate in opposite directions. As a result, the substituents that were previously trans to each other become cis in the product, and vice versa.
The Disrotatory mode of electrocyclic reaction is most often observed in thermal reactions with an even number of electron pairs. In terms of Hückel's rule, the 4n electron systems undergo Disrotatory closures under thermal conditions.
Here is a recap of the rule indicating whether a reaction will occur in the Disrotatory mode, as previously stated:
Number of pairs of electrons | Thermal conditions | Photochemical conditions |
Odd | Conrotatory | Disrotatory |
Even | Disrotatory | Conrotatory |
Practical Examples of Disrotatory Reactions
An excellent illustration of Disrotatory transformations is the photochemical reaction of cis-1,3,5-hexatriene which undergoes a six pi electron, Disrotatory electrocyclic ring-closure to form cyclohexadiene. The terminal p orbitals rotate in opposite directions, classifying the reaction as Disrotatory.
This particular reaction abides by the general rule that reactions with 4n pi electrons perform Disrotatory ring closures under photochemical conditions.
Correlation Diagram of Electrocyclic Reaction
A vital tool for visualizing and understanding the dynamics of electrocyclic reactions is the correlation diagram. It outlines the molecular orbitals involved and helps decipher how pi orbitals transform throughout an electrocyclic process.
Understanding the Correlation Diagram
A correlation diagram, also referred to as an energy level diagram, provides a graphical depiction of the energy levels of the molecular orbitals of reactant and product in an electrocyclic reaction. The diagram presents an elegant way of reconciling the disrotatory and conrotatory terminology with the conservation of orbital symmetry principle, lending an intuitive approach to discern the stereochemical outcomes of such processes.
A correlation diagram is a visual representation designed to display the correlation between the energies of reactants and products in a chemical reaction.
In an electrocyclic reaction, the diagram plots the individual molecular orbitals of the conjugated system across a potential energy surface, helping you better understand the movement and reorganization of pi electrons during the reaction. It helps monitor the continuous transformation of bonding, nonbonding, and antibonding orbitals as the system evolves from the reactant to the product state.
For instance, in the correlation diagram of butadiene’s electrocyclic reaction, the y-axis represents the energy levels of the molecular orbitals while the x-axis indicates the course of the reaction. The arrows show the movement of pi electrons, and the classification into conrotatory or disrotatory depends on the direction of the arrows.
A crucial aspect of these correlation diagrams is that they follow the principle, known as the Woodward-Hoffmann rules of orbital symmetry, guiding the stereochemical outcomes of the pericyclic reactions. Therefore, the diagram itself acts as a theoretical foundation validating these rules.
The Woodward-Hoffmann rules suggest that reactions occur in a way that conserves orbital symmetry, meaning reactants' molecular orbitals must have the same symmetry representation as the products.
Correlation Diagram in Electrocyclic Reactions for 4n and 4n2 System
The correlation diagram is particularly enlightening when it comes to predicting the behaviour of different electrocyclic reactions. Particularly, it is useful for understanding why 4n electron systems behave differently from 4n+2 systems.
The electronic state of a 4n electron system, such as 1,3,5-hexatriene, starts in the gerade symmetry, with no orbital node on the reaction coordinate at right angles to the ring plane. Therefore, under thermal conditions, they undergo disrotatory ring closures. Conversely, under photochemical activation, their electronic state switches to the ungerade, leading to conrotatory ring closures.
On the flip side, 4n+2 electron systems like 1,3-cyclohexadiene start in the ungerade symmetry, leading to conrotatory ring closures under thermal conditions or disrotatory closures under photochemical conditions.
The terms Gerade and Ungerade refer to the symmetry or antisymmetry of a molecule's wavefunction under inversion through the point of symmetry in the molecule.
These behaviours are nicely portrayed in the correlation diagrams of conrotatory and disrotatory electrocyclic reactions, for both 4n and 4n+2 electron systems. The diagrammatic representations enable the visualisation of the processes and support the deductions made through Hückel's rule and Woodward-Hoffman rules.
In a nutshell, correlation diagrams assist in connecting the concepts of molecular orbital theory and stereochemistry, providing a groundbreaking theoretical framework for delving deeper into the world of electrocyclic reactions.
The Mechanism of Electrocyclic Reaction
In the broad spectrum of organic chemistry reactions, electrocyclic reactions hold a distinctive place due to their unique mechanism characterised by the movement of pi electrons. This intriguing mechanism paves the way for ring closure or ring opening processes, leading to transforming molecular structures and stereochemistry.
Basic Electrocyclic Reaction Mechanism
The basic mechanism of an electrocyclic reaction centres on the rearrangement of pi electrons in a conjugated system, driving the structural transformation of the molecule. The ring closure or ring opening processes primarily distinguish this mechanism, where a straight-chain pi system converts into a cyclic one and vice versa. The movement of the pi electrons during this process defines whether the reaction is either conrotatory or disrotatory.
In conrotatory ring closure or opening, both ends of the pi system rotate in the same direction. Conversely, in a disrotatory transformation, the rotation of pi system ends is in opposite directions.
During an electrocyclic reaction, the conjugated pi electron system either forms a new sigma bond (ring-closure) or breaks an existing sigma bond (ring-opening). A crucial facet of these transformations is that they conserve orbital symmetry, as suggested by the Woodward-Hoffmann rules.
Let's consider the basic electrocyclic ring-closure mechanism of butadiene forming cyclobutene:
Butadiene undergoes an electrocyclic reaction in which the conjugated pi electrons are reshuffled, resulting in the formation of a new sigma bond. This transformation proceeds via a cyclic transition state, maintaining the continuous overlap of the p orbitals throughout the reaction.
The stereochemical outcome of the reaction is controlled by whether the rotation follows a conrotatory or disrotatory pathway, as discussed earlier. The specific mode of rotation results in differing product stereochemistry - either cis or trans configuration.
During the reaction, the terminal carbon atoms rotate in the same, leading to a conrotatory ring closure with a simultaneous conversion from the cis geometric isomer of the reactant to a trans configuration in the product.
Mechanisms in Conrotatory and Disrotatory Reactions
When you delve deeper into the mechanisms of conrotatory and disrotatory reactions, you'll find that the key differentiator is the direction of rotation. The direction affects the ultimate stereochemistry of the product, depending on whether it is a cis or trans isomer.
In conrotatory reactions, the terminal lobes rotate in the same direction. For instance, consider an electrocyclic reaction involving butadiene. When heated, the ends of the molecule rotate in the same direction, and the cis substituents on the ends become trans in the product. Therefore, the product's stereochemistry changes from cis to trans upon heating.
On the other hand, disrotatory reactions involve the terminal lobes rotating in opposite directions. This characteristic can be seen in an electrocyclic reaction involving cis-1,3,5-hexatriene. When illuminated, the ends of the molecule rotate in opposite directions, switching the trans substituents on the ends to a cis configuration in the product.
The specific conditions under which these reactions occur depends on the number of electron pairs involved. Generally, under thermal conditions, the reactions with an odd number of electron pairs undergo conrotatory ring closures, while those with an even number of electron pairs exhibit disrotatory ring closures. The reverse is true under photochemical conditions.
To illustrate, consider a thermal reaction involving cyclobutene, a molecule having two electron pairs (an even number). The mechanism involves a disrotatory opening where the terminal atoms rotate in opposite directions, forming the trans isomer of butadiene.
Understanding these mechanisms and the stacking of pi orbitals assists in grasping stereochemistry's nuances and the role played by the direction of rotation in shaping the product's molecular structure and properties.
No matter what type of reaction it is or the conditions under which it occurs, the change in molecular structure during conrotatory and disrotatory reactions highlights the dynamic nature of organic molecules and their potential for transformation.
Practical Examples of Electrocyclic Reactions
Looking into practical examples of electrocyclic reactions allows for a comprehensive and tangible understanding of these fascinating organic transformations. The examples clearly illustrate how these reactions operate in different scenarios, effectively showcasing the stereochemical outcomes and their dependence on various factors, such as the number of electron pairs and the condition (thermal or photochemical) under which the reactions take place.
Electrocyclic Reactions Examples
A variety of organic compounds undergo electrocyclic reactions, including dienes, hexatrienes, and tetraenes, among many others. The stereochemistry of the resulting products significantly varies, further highlighting the dynamic and interesting nature of these reactions.
Consider the following example of a thermal electrocyclic reaction:
The thermal ring-closure of butadiene forms cyclobutene. Under heat, the butadiene molecule executes a conrotatory rotation, forming a new sigma bond and adopting a cyclic structure to form cyclobutene.
Another noteworthy example involves the ring-opening of cyclobutene under thermal conditions:
Under heat, cyclobutene executes a disrotatory ring-opening, transforming back into its acyclic, straight-chain isomer - butadiene.
Ring-opening and ring-closure reactions can also be observed in hexatriene reactions. For instance:
Under thermal conditions, 1,3,5-hexatriene undergoes conrotatory ring-closure to form 1,3-cyclohexadiene.
Role of Light in Electrocyclic Reactions
Light, specifically in photochemical conditions, plays an interesting and critical role in reversing the course of the electrocyclic reactions. Consider the following examples:
Under photochemical conditions, 1,3,5-hexatriene undergoes a disrotatory ring-closure, unlike the conrotatory process seen under thermal conditions.
Similarly,
Butadiene, under photochemical conditions, undergoes a disrotatory ring-closure to form cyclobutene, differing from the conrotatory process seen under thermal conditions.
Reviewing Real Life Scenarios of Electrocyclic Reactions
To truly appreciate the electrocyclic reactions, it's useful to regard real-life scenarios utilising these organic transformation principles. A particularly cogent illustration of electrocyclic reaction is given by the biosynthesis of cholesterol.
In the process of cholesterol biosynthesis, squalene, a linear 30-carbon compound, is first converted to squalene epoxide with the use of oxygen and NADPH. Then, the squalene epoxide is transformed into lanosterol through a series of electrocyclic reactions.
The squalene epoxide first undergoes a disrotatory ring-closure to form a five-membered ring in a process known as the 2,3-squalene epoxide cyclisation. This forms a compound known as 2,3-oxidosqualene. Thereafter, two more successive conrotatory ring closures create two more rings, leading to the production of lanosterol, a key molecule in cholesterol biosynthesis.
This process offers a compelling demonstration of how electrocyclic reactions are employed by nature itself, conveying their fundamental importance in organic transformations.
Becoming familiar with these examples helps in understanding not only the mechanics and function of electrocyclic reactions, but also their wide-ranging applications and importance in synthetic and natural contexts.
Electrocyclic Reactions - Key takeaways
- Pericyclic reactions: A class of concerted reactions including electrocyclic reactions, cycloadditions, and sigmatropic rearrangements.
- Conrotatory and Disrotatory Electrocyclic Reactions: Terminologies defining the mechanism of ring closure/opening in electrocyclic reactions. Conrotatory describes a mechanism where the p orbitals at the ends of the system rotate in the same direction. Disrotatory reactions involve the p orbitals rotating in opposite directions.
- Correlation Diagram of Electrocyclic Reaction: A visual tool representing the energy levels of molecular orbitals of reactants and products in an electrocyclic reaction. It helps decipher how pi orbitals transform throughout an electrocyclic process.
- Electrocyclic Reaction for 4n and 4n+2 System: The behaviour of electrocyclic reactions in 4n and 4n+2 electron systems can be predicted using correlation diagrams. 4n electron systems undergo disrotatory ring closures under thermal conditions, while 4n+2 electron systems undergo conrotatory ring closures under the same conditions.
- Electrocyclic Reaction Mechanism: The process involves the rearrangement of pi electrons in a conjugated system, causing structural transformations of molecules. The movement of pi electrons determines if a reaction is conrotatory or disrotatory.
- Examples of Electrocyclic Reactions: Practical examples include the electrocyclic ring closure of butadiene in a conrotatory process and the photochemical reaction of cis-1,3,5-hexatriene in a disrotatory process.
Learn faster with the 15 flashcards about Electrocyclic Reactions
Sign up for free to gain access to all our flashcards.
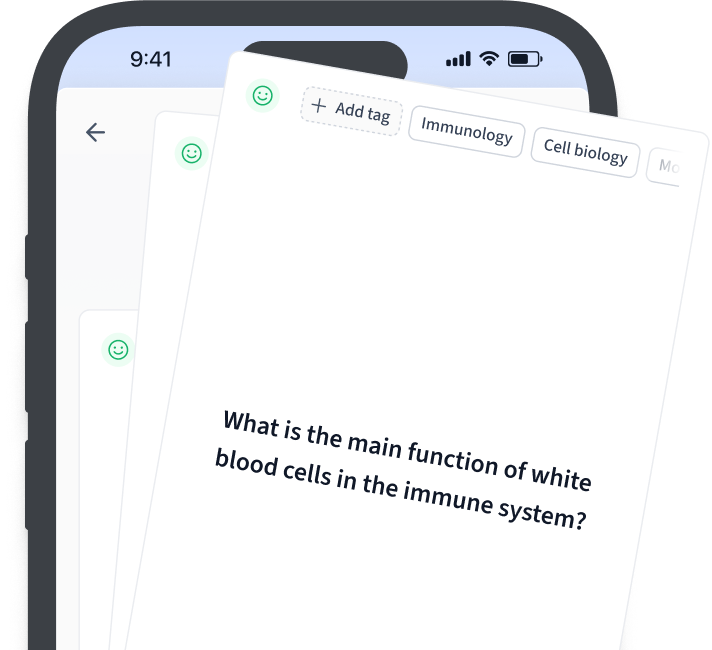
Frequently Asked Questions about Electrocyclic Reactions
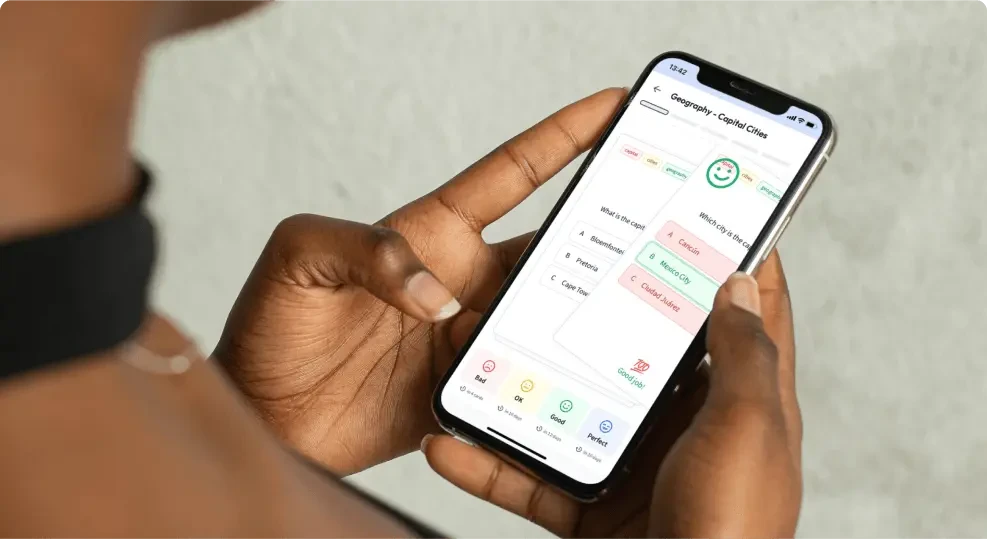
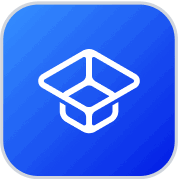
About StudySmarter
StudySmarter is a globally recognized educational technology company, offering a holistic learning platform designed for students of all ages and educational levels. Our platform provides learning support for a wide range of subjects, including STEM, Social Sciences, and Languages and also helps students to successfully master various tests and exams worldwide, such as GCSE, A Level, SAT, ACT, Abitur, and more. We offer an extensive library of learning materials, including interactive flashcards, comprehensive textbook solutions, and detailed explanations. The cutting-edge technology and tools we provide help students create their own learning materials. StudySmarter’s content is not only expert-verified but also regularly updated to ensure accuracy and relevance.
Learn more