Jump to a key chapter
What is Elimination Reaction in Organic Chemistry?
Elimination Reaction in organic chemistry is a fascinating topic that involves the removal of elements from a reactant molecule to form an unsaturated compound. This fundamental process occurs in various types of chemical reactions and serves as the basis for several synthesis reactions.Understanding the Concept of Elimination Reaction
Within the scope of organic chemistry, elimination reaction, or more specifically,beta-elimination reaction, is a process whereby a hydrogen atom and a substituent are removed from the neighbouring atoms (usually carbon) of a molecule.
- Formation of a base from KOH.
- The base abstracts a proton from the β-carbon atom.
- The electrons from the carbon-hydrogen bond move to form a π-bond.
- Simultaneously, the carbon-bromine bond breaks, releasing a bromide ion.
Although it seems like these four steps happen in a sequence, they actually occur in a concerted process. All of them are happening in one step! It's this fascinating element of organic chemistry that makes it so intriguing.
Importance of Elimination Reaction in Organic Chemistry
Elimination reaction holds a significant place in organic chemistry due to several reasons. Here's why they're so crucial:- They allow synthesis of alkenes and alkynes, unsaturated hydrocarbons that act as precursors in many industrial applications.
- The reactions are often predictable, thereby making it easier to plan synthesis in the lab or industry.
- Specific isomers can be targeted depending on the reaction conditions, making the reactions more efficient.
Consider the production of phenol from chlorobenzene, utilising elimination reaction. Here, NaOH acts as the base and extracts a hydrogen from chlorobenzene. It subsequently causes the elimination of a chlorine atom and introduces a hydroxyl (-OH) group, forming phenol. The reaction looks as follows: \[ C_{6}H_{5}Cl + NaOH \rightarrow C_{6}H_{5}OH + NaCl \] This reaction demonstrates how one functional group can be replaced by another using elimination.
Getting to Know the Elimination Reaction Mechanism
In the crucial world of organic chemistry, understanding the mechanism of the elimination reaction gives you a window into how chemical reactions occur at a fundamental level and how one type of compound is transformed into another. The mechanism of elimination reaction involves the removal of atoms, usually a proton (a hydrogen ion, \(H^+\)) and a leaving group, from a molecule, which results in the formation of a \(\pi\) bond.Detailed Explanation of the Elimination Reaction Mechanism
The mechanism of an elimination reaction is typically a two-step process. The sequence of these steps can differ, leading to a classification of elimination reactions into two types: E1 and E2. E1 mechanism: In an E1 (First order elimination) mechanism, the reaction rate depends only on the concentration of the substrate (molecule from which atoms are removed). The elimination process in E1 mechanism primarily takes place in two stages.- The leaving group detaches from the substrate producing a carbocation (a positively charged molecule).
- A base (a compound that tends to accept a proton) abstracts a proton from the carbocation, resulting in a \(\pi\) bond.
- A base abstracts a proton from the substrate, and simultaneously the leaving group departs, forming a \(\pi\) bond.
Real-life Elimination Reaction Example
Elimination reactions are fundamental components of many chemical processes that occur both in the lab and in large-scale industrial operations. A real-world application is the dehydration of ethanol to produce ethene. Here, concentrated sulfuric acid \(H_{2}SO_{4}\) acts as a dehydrating agent. The reaction mechanism follows the E1 pathway. But it's important to note that \(H_{2}SO_{4}\) isn't just a catalyst; it's a part of the mechanism. Table showing the process:Step | Action |
1 | Ethanol gets protonated by \(H_{2}SO_{4}\). |
2 | Water, a good leaving group, departs, forming a carbocation. |
3 | A base removes a proton from the carbocation, forming a \(\pi\) bond. |
Basics of Addition Elimination Reaction
Addition elimination reaction or \( \text{add-elim} \) also known as condensation reaction, is an organic reaction that forms a larger molecule through the combination of two smaller ones, while simultaneously losing a small molecule.Various Scenarios in Addition Elimination Reaction
Addition elimination reactions play out differently depending on the type of reactants involved, the reaction conditions, and the catalysts present. Among the scenarios commonly encountered are the formation of esters from carboxylic acids and alcohols under acidic conditions and the creation of amides from amines and acyl chlorides under basic conditions. The formation of an ester from a carboxylic acid and an alcohol is a classical scenario referred to as Fischer Esterification. The reaction equation is: \[ R-COOH + R'-OH \rightarrow R-COO-R' + H_{2}O \] Key steps of Fischer Esterification include:- The carboxylic acid is first protonated, enhancing its reactivity.
- Nucleophilic attack by alcohol.
- Proton transfers lead to the formation of a tetrahedral intermediate.
- Elimination of water and regeneration of the catalyst.
- The acyl chloride group reacts with the amine leading to a tetrahedral intermediate.
- Chloride ion is expelled, a step that is highly favourable since chloride ion is a good leaving group.
- Amide bond formation occurs.
Understanding Addition Elimination Reaction with Diagrams
Diagrammatic representations bring clarity to the complex interaction of atoms and bonds in these organic reactions. Take, for example, the formation of acetamide from methanoyl chloride and ammonia. Firstly, the nitrogen atom in the ammonia attacks the carbonyl carbon. This forms a tetrahedral intermediate and transfers a proton from the nitrogen to one of the chlorine atoms:


Studying the Elimination Reaction of Haloalkanes
Haloalkanes, also referred to as alkyl halides, are organic compounds wherein halogen (fluorine, chlorine, bromine, or iodine) is bonded to a carbon atom. They are prime candidates when studying elimination reactions, given their chemical reactivity and the diverse mechanisms they showcase.Designing the Reaction Pathway of Haloalkanes
To fully comprehend the elimination reactions of haloalkanes, it's crucial to map out the reaction pathway. This involves identifying the reactants, products, and the steps that connect them. Let's consider a simple case: the conversion of 2-bromopropane to propene, an E2 reaction. In the presence of a strong base like potassium hydroxide (\( KOH \)), 2-bromopropane loses a proton and a bromide ion, forming a pi-bond between the remaining carbon atoms. The reaction equation is: \[ CH_{3}CHBrCH_{3} + KOH \rightarrow CH_{3}CH=CH_{2} + KBr + H_{2}O \] The reaction pathway involves two coordinated steps that occur simultaneously:- The base abstracts a proton from an adjacent carbon atom.
- The bromide ion departs from its carbon atom, simultaneously forming the double bond.
Examples of Elimination Reaction of Haloalkanes
Expanding your understanding of how haloalkanes react, let's look into varied types of elimination reactions, namely E1 and E2. E1 Example: An E1 reaction involves the formation of a carbocation intermediate. It thus favours substrates that can form a stable carbocation. Consider the following dehydration of tert-butyl bromide: \[ (CH_{3})_{3}CBr + H_{2}O \rightarrow (CH_{3})_{3}C^{+} + H_{2}O + Br^{-} \] \[ (CH_{3})_{3}C^{+} + H_{2}O \rightarrow (CH_{3})_{3}COH + H^{+} \] \[ (CH_{3})_{3}COH \rightarrow (CH_{3})_{2}C=CH_{2} + H_{2}0 + H^{+} \] E2 Example: The reaction of 2-bromobutane with potassium ethoxide is an example of an E2 reaction. In this bimolecular reaction, a strong base like potassium ethoxide is provided in a solvent like ethanol. This reaction follows: \[ BrCH_{2}CH_{2}CH_{2}CH_{3} + C_{2}H_{5}OK \rightarrow CH_{2}=CHCH_{2}CH_{3}+ KBr + C_{2}H_{5}OH \] The reaction involves the simultaneous loss of the bromine atom and the base-induced removal of an adjacent proton. This reaction is stereoselective: the reactant and the base should be in an anti-coplanar conformation for the pi bond to form computationally. Studying these varied scenarios will give you a rich understanding of the elimination reactions and how they function under different conditions. This knowledge is essential in numerous fields, including synthesising complex organic compounds and understanding biochemical reaction pathways.The Role of Alcohol in Elimination Reaction
When venturing into the realm of organic chemistry, the role of alcohol in elimination reactions quickly becomes apparent. Alcohols, characteristically possessing an \(OH\) group, can readily lose water in a process known as dehydration, which falls under the elimination reaction mechanism. The product of this dehydration process is an alkene. The reaction proceeds typically under the influence of a strong acid, facilitating the removal of the \(OH\) group, which is a poor leaving group under normal circumstances.Alcohol Elimination Reaction: A Step-by-Step Guide
The mechanism of alcohol elimination hinges on the principle of protonation, coupled with the departure of a good leaving group. Before delving into the specifics, it's important to understand what happens during this protonation. In essence, protonation refers to the addition of a proton (\(H^+\)) to a molecule, which usually occurs in the presence of an acid. Consider the dehydration of ethanol (C\(_2\)H\(_5\)OH), a simple alcohol, to ethene (C\(_2\)H\(_4\)): \[ C_{2}H_{5}OH \rightarrow C_{2}H_{4} + H_{2}O \] Table detailing the step-by-step process:Step | Action |
Step 1 | The reaction begins with the protonation of ethanol by an acid, transforming the \(OH\) group into a better leaving group, \(H_{2}O\). This generates a positively charged and unstable carbocation. |
Step 2 | The water molecule departs leaving behind a carbocation. |
Step 3 | An adjacent hydrogen atom is then abstracted by a base present in the medium, leading to the formation of an alkene. |
The Impact of Different Types of Alcohol on Elimination Reaction
The type of alcohol does indeed have a profound effect on the outcome of the elimination reaction. The structural differences present in primary, secondary, and tertiary alcohols influence the reaction mechanism and product distribution. Primary Alcohols: Primary alcohols have the hydroxy group bound to a carbon that is in turn attached to no more than one other carbon atom. In these alcohols, the elimination majorly follows the E2 mechanism, as the formation of a primary carbocation is highly unstable. The reaction equation for this elimination is: \[ C_{2}H_{5}OH + H_{2}SO_{4} \rightarrow CH_{2}=CH_{2} + H_{2}O + H_{2}SO_{4} \] Secondary and Tertiary Alcohols: In contrast, secondary and tertiary alcohols, where the alcohol carbon is bound to two and three carbons respectively, favour the E1 mechanism. This predilection is because secondary and especially tertiary carbocations are much more stable. A classic example is the dehydration of tert-butyl alcohol: \[ (CH_{3})_{3}COH + H_{2}SO_{4} \rightarrow (CH_{2}C(CH_{3})_{2}=CH_{2} + H_{2}O + H_{2}SO_{4} \] In some cases, however, both E1 and E2 pathways might be facilitated, depending upon experimental conditions like temperature and type of acid used. Understanding how the structural aspects of different alcohols influence elimination reactions gives you insight into reaction kinetics and organic synthesis. With this knowledge, you can predict the course and outcome of an elimination reaction involving alcohol.Key Conditions for Efficient Elimination Reaction
Understanding the right conditions for any chemical reaction, not least for an elimination reaction, is pivotal for successful outcomes. The conditions dictate the progression of the reaction, influencing the reaction mechanism, the ease of the reaction, and the nature of the products formed.Defining Ideal Conditions for Elimination Reaction
The effectiveness of an elimination reaction depends on several factors. These include the concentration and nature of the reactants, the temperature, the reagent used, and the reaction medium. Each of these parameters heavily impacts the reaction pathway. Reactants: The reactivity of a haloalkane, which serves as the usual substrate in elimination reactions, depends on the halogen and the nature of the alkyl group. The rate of the reaction inflates with the increasing size of the halogen, i.e., iodine > bromine > chlorine, owing to the effectiveness of the leaving group. Additionally, as a rule of thumb, increased branching of the alkyl group accelerates the reaction due to the enhanced stability of the intermediate carbocation. Reaction Medium: The medium, particularly the solvent used in elimination reactions, is pivotal. Protic solvents favour E1, whereas aprotic solvents facilitate the E2 mechanism. Temperature: Elevated temperature is typically essential for elimination reactions. A higher temperature not only increases the kinetic energy of the reactant molecules but also tends to favour the production of alkenes—end products of elimination reactions—following Le Chatelier's principle. Reagent: The reagent type also governs the elimination reaction. Acidic conditions usually lead to the E1 mechanism, while a strong base typically leads to the E2 mechanism. The following table illustrates some reactant-reagent combinations and the resultant mechanisms:Reactant | Reagent | Condition | Predominant Mechanism |
Primary alkyl halide | Weak or strong base | Hot | E2 |
Secondary alkyl halide | Weak base or acid | Warm | E1 |
Secondary alkyl halide | Strong base | Hot | E2 |
Tertiary alkyl halide | Weak base or acid | Warm | E1 |
Tertiary alkyl halide | Strong base | Hot | E2 |
The Impact of Reaction Conditions on the Outcome
Changing the conditions - either the temperature, the solvent, or the choice of the base - can dramatically alter the course and outcome of the elimination reaction. Temperature: A higher temperature favours more branched alkenes through the elimination reaction. This trend is in accordance with the Zaitsev's rule. This rule states that in an elimination reaction, the most substituted, i.e., the most stable, alkene is the major product. Solvent: The choice of solvent can switch the mechanism from E1 to E2. Polar, aprotic solvents like dimethyl sulfoxide (DMSO) favour the E2 mechanism by stabilizing the negatively charged base. Conversely, polar, protic solvents such as water or alcohol favour carbocation formation and thus catalyse the E1 reaction. Base: The strength and the size of the base influence not only the rate but also the products of the reaction. Bulky bases promote the E2 mechanism and are likely to lead to less substituted alkenes due to steric hindrance, contravening Zaitsev's rule. This phenomenon is termed as the Hoffmann elimination. To conclude, reaction conditions have profound impacts on the elimination reactions, dictating their path and determining their products. Hence, a comprehensive knowledge of these effects is vital when designing experimental setups in the lab or when predicting reaction outcomes in theoretical problems.Elimination Reaction - Key takeaways
- Elimination reactions in organic chemistry are significant as they underpin the complexity and versatility of the field. Mastery of these reactions is crucial for becoming an accomplished chemist.
- The mechanism of elimination reaction involves the removal of atoms from a molecule, resulting in the formation of a pi bond. It usually involves a proton and a leaving group.
- Elimination reactions are classified into two types: E1 and E2, depending on the reaction rate. E1 reactions depend only on the concentration of the substrate, while E2 reactions depend on both the substrate and the base.
- Real-life application of elimination reactions includes the dehydration of ethanol to produce ethene, where concentrated sulfuric acid acts as a dehydrating agent following the E1 pathway.
- Understanding the alcohol elimination reaction, the product of this dehydration process is an alkene. The reaction proceeds under the influence of a strong acid. The type of alcohol (primary, secondary, or tertiary) influences the reaction mechanism and product distribution.
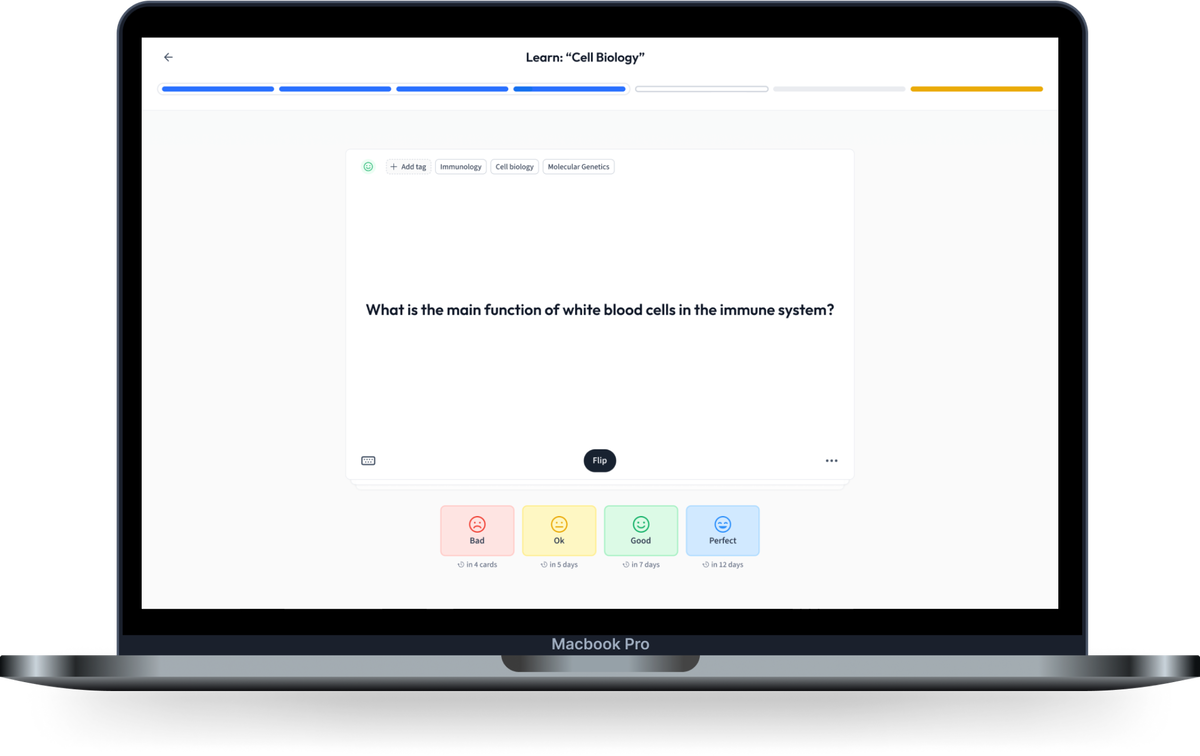
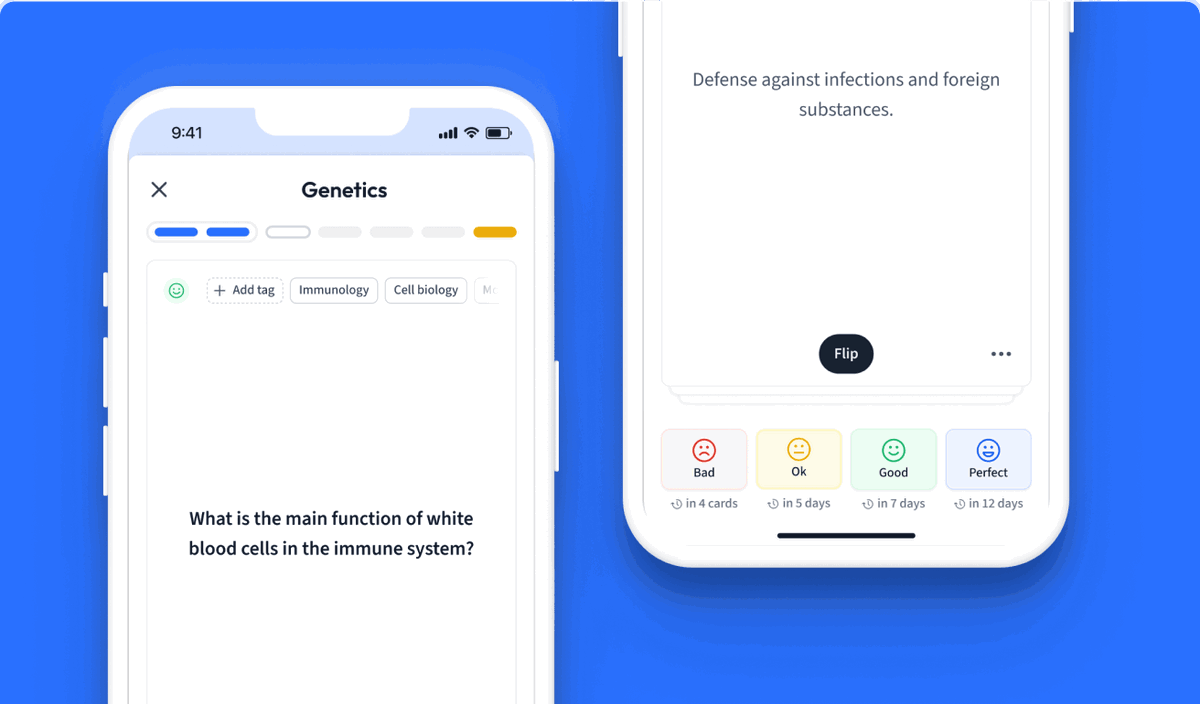
Learn with 12 Elimination Reaction flashcards in the free StudySmarter app
We have 14,000 flashcards about Dynamic Landscapes.
Already have an account? Log in
Frequently Asked Questions about Elimination Reaction
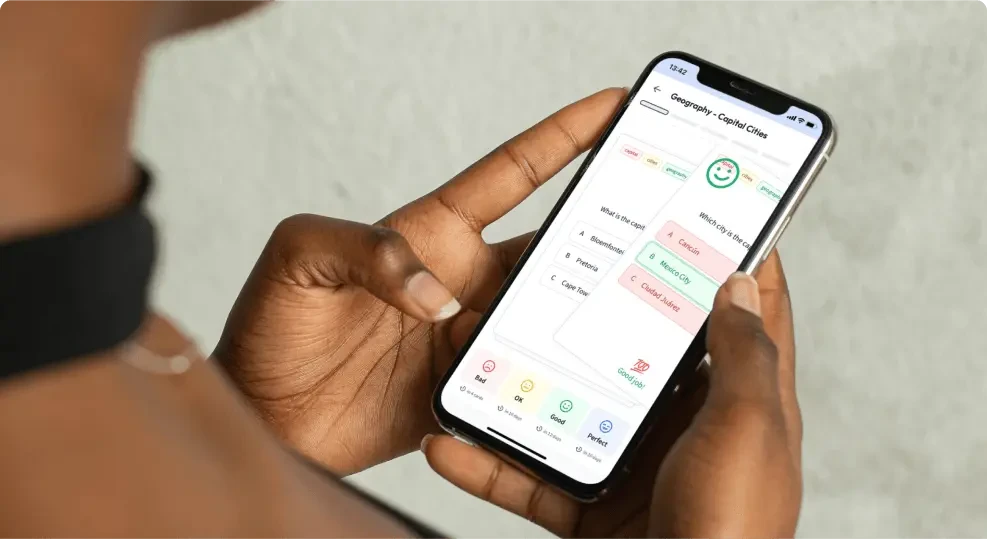
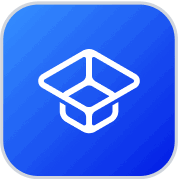
About StudySmarter
StudySmarter is a globally recognized educational technology company, offering a holistic learning platform designed for students of all ages and educational levels. Our platform provides learning support for a wide range of subjects, including STEM, Social Sciences, and Languages and also helps students to successfully master various tests and exams worldwide, such as GCSE, A Level, SAT, ACT, Abitur, and more. We offer an extensive library of learning materials, including interactive flashcards, comprehensive textbook solutions, and detailed explanations. The cutting-edge technology and tools we provide help students create their own learning materials. StudySmarter’s content is not only expert-verified but also regularly updated to ensure accuracy and relevance.
Learn more