Jump to a key chapter
What is Enzyme Stereospecificity?
Enzyme stereospecificity plays a vital role in many biochemical reactions. But you may wonder exactly what is it? Put simply, it refers to the property of an enzyme to act on a specific stereoisomer.Stereoisomers are compounds that have the same molecular formula but different spatial arrangement. They could be mirror images of each other (like your hands) or could be oriented differently around a double bond.
Enzyme Stereospecificity Definition
By now you may understand that enzymes are not 'one-size-fits-all'. They show preferences for certain types of isomers. A basic definition of enzyme stereospecificity is:The exclusive or preferential interactions of enzymes with certain stereoisomers over others.
This is a bit like a lock and key mechanism where the lock (enzyme) opens only for the right key (isomer). Enzymes can precisely identify these isomers due to their own intricate 3D structures which include several active sites. These active sites are like contours of the 'key' it is looking for.
Factors Affecting Enzyme Stereospecificity
Several factors can affect the stereospecificity of an enzyme. Let's understand some of these:Molecular structure: The 3D structure of an enzyme and its substrate greatly influences the interactions between them.
Conditions of reaction: Factors like pH, temperature, and presence of other molecules could alter how an enzyme interacts with its substrate.
Nature of the substrate: Certain substrates have chemical properties that make them more or less likely to interact with specific enzymes.
Let's consider the example of the enzyme Alcohol Dehydrogenase (ADH). ADH preferentially acts on primary alcohols and shows little to no activity for secondary or tertiary alcohols. That's because the structure of secondary and tertiary alcohols doesn't fit onto the active sites of the enzyme. So, even if the feet are the same size, the wrong shoe (substrate) will not fit into the foot (enzyme).
Breaking Down Enzyme Stereospecificity
Enzyme stereospecificity isn't just a whimsical preference of enzymes; it's a biochemical necessity. To efficiently manage the countless biochemical reactions happening every second, enzymes must be specific in their interactions, ensuring reactions occur appropriately and at the right pace.Enzyme Stereospecificity Explained
To deepen your understanding of enzyme stereospecificity, you should familiarise yourself with some key concepts. Chirality in chemistry refers to the asymmetrical structure of molecules. It's like the mirror image issue in your hands; you can't superimpose one hand perfectly on to the other. Molecules having such a property are called chiral molecules, and the different forms are called enantiomers.Enantiomers are pairs of molecules that are mirror images of each other, just like your right and left hands.
Role of Stereospecific Enzymes in Biological Reactions
Enzyme stereospecificity serves a plethora of roles in biological reactions. From the digestion of food to the synthesis of DNA, enzymes with their stereospecificity act as controllers of biochemistry.In digestion, where the enzyme amylase breaks down starch into simpler sugars, enzyme stereospecificity ensures that only specific linkages in the starch molecule are broken down. This generates simpler sugars that are ideal for our bodies to absorb and utilise for energy.
Chemical Aspects of Enzyme Stereospecificity
Let's now delve deeper into the chemical aspects of enzyme stereospecificity, specifically how enzymatic reactions follow certain stereochemical principles. Any reaction catalysed by an enzyme involves the formation of transition states and intermediates. An enzyme’s active site is complementary not just to the substrate, but also to these transition states and intermediates. A calculated chemical reaction model for an enzyme might look like this: \[ \text{Enzyme} + \text{Substrate} \xrightarrow[\text{}]{\text{Transition state}} \text{Enzyme-Substrate} \xrightarrow[\text{}]{\text{Transition state}} \text{Enzyme} + \text{Product} \] In these models, the focus is usually on the substrate and product ignoring the crucial transition states. However, these transition states actually direct the course of the reaction, and the enzyme is designed accordingly to stabilise them. Enzymes doing so lowers the activation energy of the reaction, making the reaction more fast and efficient. This elucidates why enzymes are stereospecific. Even small changes in the orientation (stereoisomerism) of the substrate might lead to the formation of different transition states, which the enzyme might not be equipped to handle. Thus, enzymes are custom made for specific substrates, contributing to their stereospecificity.Examples of Enzyme Stereospecificity
As you delve deeper into the fascinating world of enzymes and their stereospecific nature, it's important to explore some examples to illustrate how this specificity functions in the real biochemical world. These examples will enable you to fully appreciate the extent and importance of enzyme stereospecificity.Stereospecific Enzyme Example in Biochemistry
The examples of enzyme stereospecificity are innumerable from the realm of biochemistry, with enzymes precisely picking and choosing their substrates among a multitude of compounds. Let's explore a few notable instances: 1. Dehydrogenase enzymes: If you've peeped into organic chemistry, you'd know the subtle yet significant difference between an R and S configured compound. But guess what? Enzymes know it too. For instance, L-lactate dehydrogenase specifically acts on L-lactate and not on D-lactate. It's a bit like having a specific screwdriver for a specific type of screw. 2. DNA polymerase: This enzyme is responsible for the duplication of DNA. It follows a strict rule while adding nucleotides to the growing DNA strand, which is stereospecific. Because of this principle, it selects deoxyribose sugar over its isomer ribose, ensuring the synthesis of DNA and not RNA. 3. Aminoacyl-tRNA Synthetase: This enzyme adds a specific amino acid to its corresponding tRNA during protein synthesis. It selects only the L-isomer of amino acids, rejecting the D-isomers. What's fascinating is how these enzymes manage this high level of specificity. For each substrate, the enzyme has a unique docking site designed just for that, rejecting substrates that show even slight differences in structure. Understanding this requires a detailed look at the nature of the interactions between an enzyme and its substrate, and that's precisely what we'll delve into next.Case Study: Stereospecific Enzyme Reaction
To paint a clearer, more precise picture of enzyme stereospecificity, a deep dive into a single enzyme can be immensely helpful. Let's dive into a vivid case study featuring Alcohol Dehydrogenase (ADH), an enzyme critical for alcohol metabolism. ADH targets ethanol, converting it to acetaldehyde. It's a reduction-oxidation reaction, where ethanol is oxidised and a coenzyme NAD+ is reduced. However, ADH doesn't stop at ethanol. It also acts on a variety of other primary alcohols. But, the reaction speed or efficiency is different for each alcohol. ADH is stereospecific, indeed. What explains this behaviour is the unique 'key-lock' fit of the enzyme and its substrate. ADH's stereochemistry consists of a finely shaped active site that fits different molecules differently. This causes different reaction rates. However, not all alcohols are treated equally. Primary alcohols adhere closely to the ADH's active site, leading to a fast reaction. However, secondary and tertiary alcohols do not 'fit' into the site as perfectly. The enzyme is unable to position these alcohols efficiently for the catalysis, leading to a slow reaction or, in some cases, no reaction at all. The following table gives a better understanding:Alcohol Type | Reaction with ADH |
Primary Alcohols | Fast reaction |
Secondary Alcohols | Slow or no reaction |
Tertiary Alcohols | Slow or no reaction |
Enzyme Stereospecificity in Catalysis
Appreciating enzyme stereospecificity requires acknowledging its role in catalysis. The action of enzymes is not random; instead, it's intricately designed, resulting in precise control over the biochemical reactions, facilitating efficient catalysis.Enzyme Stereospecificity and Catalysis: A Detailed Look
Before we delve into the specifics of catalysis in relation to enzyme stereospecificity, it's essential to understand catalysis itself. Enzymes accelerate reactions by acting as a catalyst. A catalyst is a substance that speeds up a chemical reaction but remains unchanged at the end of the reaction. Enzymes, being biological catalysts, enhance the reaction rate by providing an alternative reaction pathway that requires lower activation energy. Another crucial aspect of enzyme catalysis is what is commonly referred to as the enzyme-substrate complex. An enzyme binds to its substrate, reacts it, and then releases the product. This can be represented by a simple equation as: \[ \text{enzyme (E)} + \text{substrate (S)} \rightarrow \text{enzyme-substrate complex (ES)} \rightarrow \text{enzyme (E)} + \text{product (P)} \] In the context of stereospecificity, the substrate isn't any random molecule; instead, the enzyme is particular about the substrate it binds with, highlighting the enzyme's substrate specificity. The 'lock and key’ model of enzyme action explains this well. In this analogy, the enzyme is represented by a lock and the substrate as a key. Unless the right key is used, the lock cannot be opened. Similarly, an enzyme can catalyse a reaction only if the substrate 'fits' correctly into its active site. But how does this substrate specificity translate to stereospecificity? The answer lies in the spatial arrangement of atoms in a molecule, which is referred to as stereochemistry. Substrate molecules can exist as mirror-image forms, known as enantiomers, depending on the spatial arrangement of the functional groups (like -OH, -NH2, -COOH) attached to a carbon atom.Understanding Catalysis through Stereospecific Enzyme Reactions
Enzymes, in their quest for the right substrate, don't merely look at the substrate's chemical nature; they are also concerned with the spatial configuration of the substrate. This attribute extends our earlier 'lock-key' analogy to a more refined '3D lock-3D key' relationship. To envision this, let's consider an enzyme that is stereospecific for the R-enantiomer of a compound but does not act on the S-enantiomer of the same compound. Although chemically both these enantiomers are the same, their spatial arrangement is different, much like our right hand is different from our left hand (despite both being hands). The enzyme can differentiate between these mirrored molecules and 'prefers' one over the other to catalyse the reaction.Stereospecificity means the enzyme is highly specific not just in the type of reaction it catalyses but also in the stereochemistry of the substrates and products.
Stereospecificity: A Catalyst for Selectivity and Precision in Enzyme Action
A crucial aspect of enzyme stereospecificity in catalysis becomes evident when we look beyond the substrate-enzyme interaction and towards the overall biochemical pathway. The high degree of selectivity an enzyme demonstrates contributes to the effective functioning of these complex networks of reactions. The specificity ensures that the reaction proceeds in the desired direction and produces the correct product. For example, consider the situation where a molecule can undergo two different reactions leading to two different products. If enzymes were not stereospecific, they might catalyse both the reactions, resulting in a mix of products. Such a situation would create chaos within the cell, defeating the purpose of having enzymes and metabolic pathways.Keeping Unwanted Reactions at Bay
Linked to the aspect of immediate specificity, enzyme stereospecificity also operates as a crucial gate-keeping function in biochemistry, preventing unwanted reactions.- Prevents creation of unwanted substances
- Avoids energy waste through unproductive reactions
- Controls overall progress of metabolic pathways
Advanced Concepts Related to Enzyme Stereospecificity
Delving into the domain of enzyme stereospecificity, you'll find there are other interconnected advanced concepts that bear significance. Understanding these can enhance your grasp of enzyme stereospecificity and its influential role in the realm of biochemistry.
Impacts of Enzyme Stereospecificity on Cellular Processes
A captivating examination of enzyme stereospecificity would be incomplete without appreciating its profound impacts on cellular processes. These specialised bio-machines facilitate vital biochemical reactions, acting as meticulous and reliable gatekeepers within the cell. Let's explore the overarching themes regarding how enzyme stereospecificity impacts cellular processes:Controlled Metabolic Pathways: Cellular metabolism is not a haphazard sequence of reactions; it's a coordinated series of enzymatic reactions. Enzyme stereospecificity ensures that only the correct substrates enter these pathways, preventing erroneous reactions, maintaining the integrity and efficiency of metabolic pathways, and contributing to the overall regulation of metabolism.
Regulation of Cellular Energy: Biochemical reactions can involve the transfer of energy. Enzyme stereospecificity comes into play here as well. It ensures that the catalysed reactions are thermodynamically favourable and support the cell's energy needs. This contributes to maintaining the energy balance within the cell.
Accuracy in Genetic Information Processing: Enzyme stereospecificity plays a crucial role in the processes of replication, transcription, and translation, which are foundational in transmitting and interpreting genetic information. This accuracy is vital in maintaining the integrity of the genetic information, with implications for growth, development, and overall survival of the organism.
Recent Research Advances in Enzyme Stereospecificity
Research in the realm of enzyme stereospecificity is ongoing, pushing the boundaries of our understanding and discovery of new realms of biochemical specificity. The following aspects represent some of the fascinating recent advances:Unravelling the Complexity of Enzyme Structure: Recent studies have uncovered the intricate details of enzyme active sites, leading to a deeper understanding of factors governing enzyme stereospecificity. The breakthroughs in bioinformatics, computational biology, and structural biology have made these insights possible, providing a comprehensive ‘3D view’ of the enzymatic activity.
Exploring 'Promiscuous' Enzymes: While enzymes are known for high selectivity, some enzymes, known as 'promiscuous' enzymes, can cater to multiple substrates or catalyse different reactions. Recent research focusing on these enzymes hints at a flexibility within the enzyme stereospecificity paradigm.
Understanding Enzyme Evolution: Advances have also been made in understanding how enzymes evolved their specificity and efficiency. Research suggests that enzymes might have started as promiscuous entities that gradually evolved specificity, providing fascinating insights into the evolutionary aspect of enzyme stereospecificity.
Enzyme Stereospecificity - Key takeaways
- Enzyme stereospecificity refers to the specificity of enzymes in their interactions to catalyse correct biochemical reactions efficiently and at the right pace.
- Chirality refers to the asymmetrical structure of molecules, leading to chiral molecules and different forms called enantiomers. Enzymes show preference for one enantiomer over another due to their three-dimensional structure, leading to enzyme stereospecificity.
- Enzyme stereospecificity is important in various biological reactions, such as the digestion of food or the synthesis of DNA, where stereospecific enzymes act as controllers of biochemistry.
- Enzyme stereospecificity also comes into play in catalysis where enzymes, acting as catalysts, expedite reactions by providing an alternative pathway requiring lower activation energy.
- Examples of enzyme stereospecificity in biochemistry include dehydrogenase enzymes, which act on specific isotopes, and DNA polymerase, which follows strict rules when adding nucleotides to a growing DNA strand.
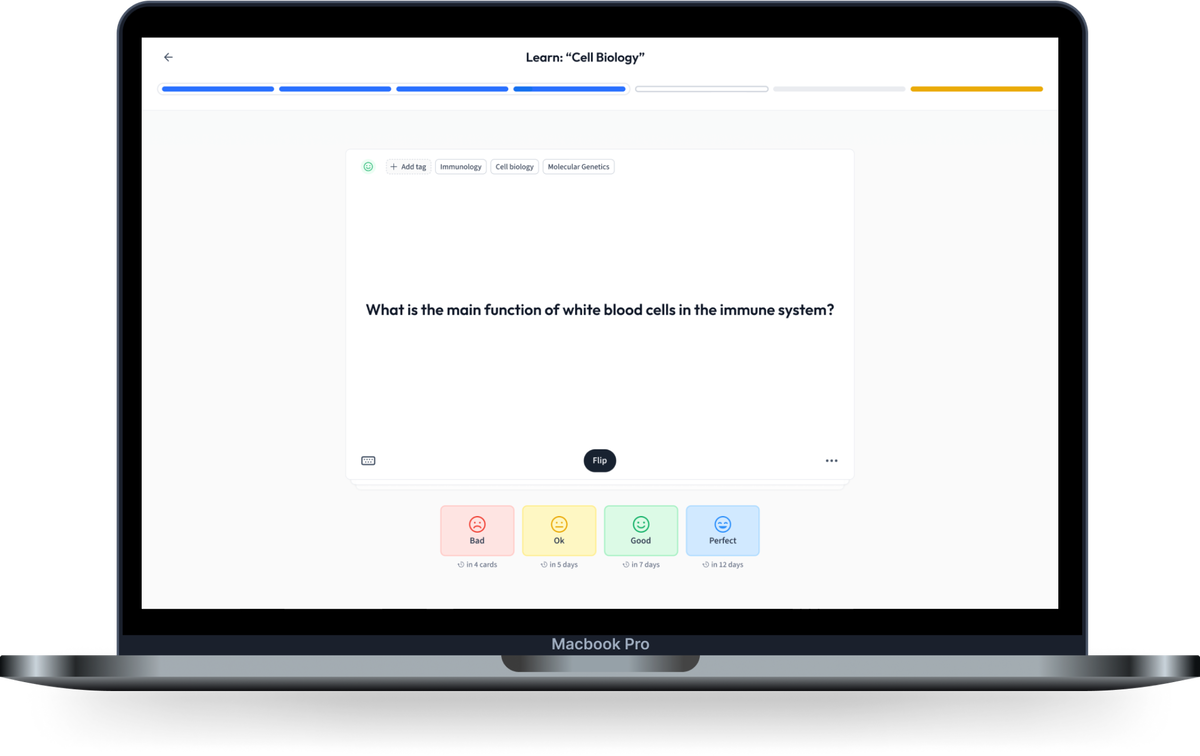
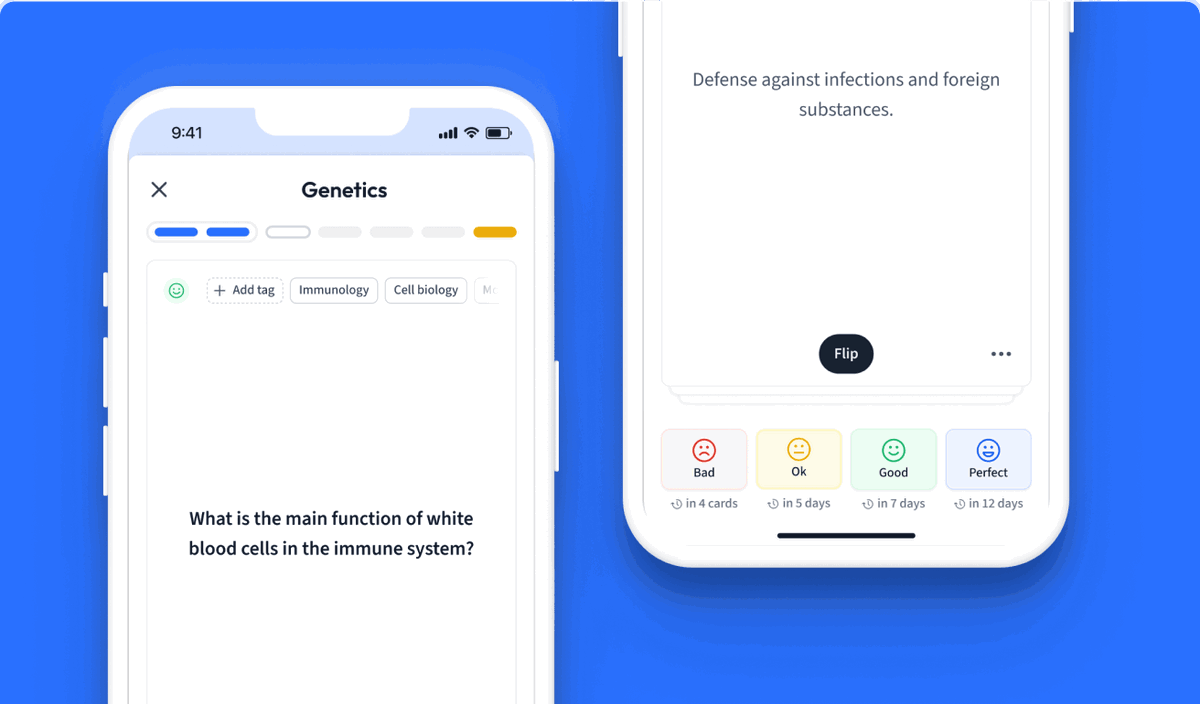
Learn with 15 Enzyme Stereospecificity flashcards in the free StudySmarter app
We have 14,000 flashcards about Dynamic Landscapes.
Already have an account? Log in
Frequently Asked Questions about Enzyme Stereospecificity
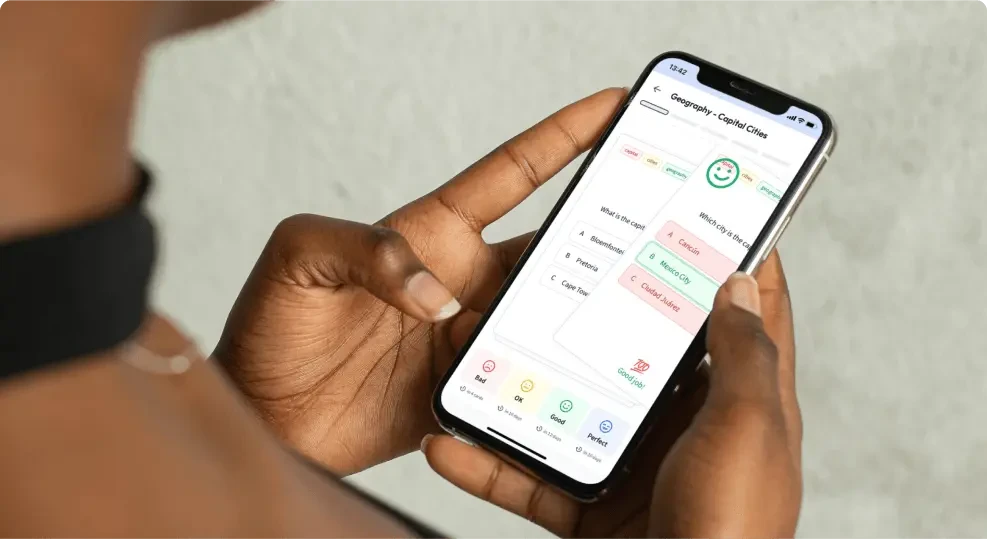
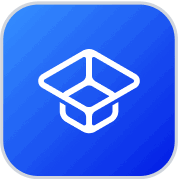
About StudySmarter
StudySmarter is a globally recognized educational technology company, offering a holistic learning platform designed for students of all ages and educational levels. Our platform provides learning support for a wide range of subjects, including STEM, Social Sciences, and Languages and also helps students to successfully master various tests and exams worldwide, such as GCSE, A Level, SAT, ACT, Abitur, and more. We offer an extensive library of learning materials, including interactive flashcards, comprehensive textbook solutions, and detailed explanations. The cutting-edge technology and tools we provide help students create their own learning materials. StudySmarter’s content is not only expert-verified but also regularly updated to ensure accuracy and relevance.
Learn more