Jump to a key chapter
Understanding Halogenation of Alkanes
Halogenation of alkanes holds a significant position in organic chemistry. This fascinating topic is a guide to understanding a crucial chemical process that involves halogen molecules. It will help you appreciate the beauty and logic of chemistry.
Dive into Halogenation of Alkanes Meaning
Halogenation of alkanes simply refers to the substitution reaction where a hydrogen atom in an alkane is replaced by a halogen (a group VII element).
An Alkane is a hydrocarbon containing only single covalent bonds. They are the simplest kind of hydrocarbons, and are also referred to as paraffins.
A Halogen is any of the elements found in Group VII of the periodic table. They include Fluorine (F), Chlorine (Cl), Bromine (Br), Iodine (I), and Astatine (At).
Several factors can influence the rate and success of halogenation reactions. To understand this topic well, it is necessary to break it down into its primary concepts.
Breaking Down Halogenation of Alkanes
Halogenation of alkanes unfurls across a series of steps, with distinct phases defined as initiation, propagation, and termination.
- Initiation: This is the very first step of halogenation. The process involves breaking the halogen-halogen bond by the application of heat or light, leading to the formation of two reactive halogen free radicals.
- Propagation: This is the longest and most important phase. A free radical formed from initiation reacts with an alkane molecule to form a new free radical.
- Termination: This process marks the end of the reaction. It happens when a bond forms between two free radicals generated in the propagation stage.
One major feature that distinguishes halogenation is the concept of reactive intermediates, which are atoms, ions, or molecules that exist in a reaction's transition state. Specific reactions have intermediates that are either more or less stable, affecting the overall energy of a reaction and thereby influencing the reaction rate.
The three stages of halogenation are reminiscent of a relay race. Initiation is akin to the first runner passing the baton to the second runner, who signifies propagation. Ultimately, the race ends when the final runner, signifying termination, crosses the finish line.
Unpacking the Theory of Halogenation of Alkanes
The theory of halogenation of alkanes plays a significant role in the field of organic chemistry. It helps explain why certain chemical reactions take place and what factors can influence these reactions.
For instance, the reactivity of a halogen in halogenation increases with its size and number of outer shell electrons. This is why, in general, iodine is more reactive than bromine, bromine is more reactive than chlorine, and chlorine is more reactive than fluorine.
Additionally, the intermediate free radicals' stability can also influence the course of reaction. Tertiary carbon radicals are typically more stable than secondary or primary carbon radicals due to the hyperconjugation effect. As such, tertiary alkanes will react faster than primary alkanes in halogenation.
For example, when propane is subjected to chlorination, the chlorine molecules dissociate into chlorine free radicals during initiation due to the application of heat or light. These chlorine free radicals then abstract a hydrogen atom from propane, thereby generating a propyl radical and a Hydrogen Chloride (HCl) molecule during propagation. In termination, two free radicals combine to form a molecule, thereby ending the reaction. Here, a propyl radical can combine with a chlorine radical to form a final product - Propyl Chloride.
Decoding the Key Concepts in Halogenation
When learning the theory of halogenation of alkanes, you will come across several key concepts that are central to understanding the topic. They include;
- Free radicals: These are atoms or groups of atoms that contain an unpaired electron.
- Hydrogen Abstraction: This process refers to the removal of a hydrogen atom from a molecule by a free radical.
- Disproportionation: It is a process in which a single reactant is transformed into two different products.
Understanding these key concepts will help you unravel the intricacies of the theory of halogenation of alkanes and appreciate the logic behind organic chemistry.
Practical Insights: Halogenation of Alkanes Examples
Chemistry is not just about theories and postulates. Every concept taught has far-reaching implications in practical applications, and the halogenation of alkanes is no exception. To gain comprehensive insight into halogenation of alkanes, let's move beyond the realm of textbook definitions and venture into the real-world examples of this pivotal chemical process.
The Relevance of Halogenation of Alkanes Examples
Understanding the halogenation of alkanes through examples not only cements your grasp on the underlying principles but also demonstrates its pervasive influence in various industries. Halogenated alkanes play crucial roles in the pharmaceutical, agricultural, and chemical sectors.
A Halogenated Alkane is an alkane in which one or more hydrogen atoms have been replaced by halogen atoms. Depending on the number of halogens, they could be classified as mono, di, or tri halogenated alkanes.
In the pharmaceutical industry, halogenation is a go-to method for drug synthesis. The introduction of halogens into organic compounds can alter the biological activity of these compounds, refining drug efficiency or reducing adverse side effects. For example, the anti-inflammatory drug, Flurbiprofen, is a chlorinated alkane.
In agriculture, halogenated alkanes are widely used as pesticides to protect crops from pests. A common example is 1,2-Dibromoethane, an effective soil fumigant and pesticide.
In industries, chlorinated alkanes are used as flame retardants, plasticizers, and coolant in metalworking operations. For example, Trichloroethane, a widely used industrial solvent.
For instance, in the halogenation of ethane with chlorine, you start with Ethane, \(C_2H_6\), and Chlorine, \(Cl_2\). Upon exposure to UV light, one chlorine atom swaps with a hydrogen atom in ethane, yielding chloroethane, \(C_2H_5Cl\), and Hydrogen Chloride, \(HCl\). The chemical equation is expressed as: \(C_2H_6 + Cl_2 \xrightarrow{UV} C_2H_5Cl + HCl\)
These examples convey the quintessential role of halogenation in creating beneficial products for various industries. Learning with examples will not only assist in better comprehension but also develop an appreciation of these concepts' practical implications.
Practical Application: Halogenation of Alkanes Examples
Moving forth, let's delve deeper into real-life examples that illustrate the significance of alkane halogenation.
Consider the reaction between methane \(CH_4\) and bromine \(Br_2\). In the presence of UV light or high temperatures (around 300-400°C), bromine radicals are formed which sets off a chain reaction yielding bromomethane \(CH_3Br\) and Hydrogen Bromide \(HBr\).
Its chemical equation is: \(CH_4 + Br_2 \xrightarrow{UV} CH_3Br + HBr\) This reaction signifies an example of monohalogenation where only one hydrogen atom of methane is substituted with a bromine atom. Furthermore, under certain conditions, the reaction can continue, leading to the replacement of all hydrogen atoms with bromine atoms, illustrating polyhalogenation. This results in tetrabromomethane \(CBr_4\).
Another relevant example involves the chlorination of ethane, a major constituent of natural gas. When ethane is reacted with chlorine in the presence of sunlight, you get chloroethane and hydrogen chloride. Chloroethane is employed in the production of ethylene, a prime compound in the petrochemical industry.
This reaction can be represented as: \(C_{2}H_{6} + Cl_{2} \xrightarrow{UV} C_{2}H_{5}Cl + HCl\)
These examples underline how halogenation of alkanes has profound utilitarian value in industry and agriculture. The real-world touch to halogenation allows you to observe how this seemingly abstract organic chemistry concept tangibly impacts everyday living.
Applications of Halogenation of Alkanes
The halogenation of alkanes, a cornerstone in organic chemistry, boasts a diverse array of applications. It's a fundamental process, bridging the molecular landscape of everyday life from pharmaceuticals to agrochemicals, from refrigerants to solvents. Through chemotherapy treatments, chemical accidents prevention, crop protection, and far beyond, halogenation touches multiple corners of human life.
Real-Life Implementation of Halogenation of Alkanes
The essence of scientific discovery lies not merely in the abstract knowledge it imparts but also its real-life implications. That's where the halogenation of alkanes comes into the frame, featuring robustly in practical applications around the world.
One of the most pronounced applications of halogenation of alkanes is in the pharmaceutical industry. Halogenated alkanes are the backbone of several medicinal drugs. For instance, incorporating a halogen in the molecular structure of a drug can improve its efficiency, reducing unwanted side effects. Drugs like Fluticasone (used to prevent asthma attacks), Vardenafil (for treating erectile dysfunction), and Prozac (for depression) all rely on halogenated alkanes.
Agrochemicals industry also employs halogenation of alkanes extensively. Many pesticides and herbicides contain halogenated compounds, which are more effective and potent due to their enhanced reactivity. Dicamba, a potent herbicide, and Methyl bromide, a powerful pesticide, are examples of halogenated agrochemicals.
In everyday life, halogenated alkanes play a substantial role in the production of refrigerants. The classic example here is Freon, a chlorofluorocarbon (CFC), which has been widely used as a refrigerant and aerosol propellant. Though, due to environmental considerations, its use is now under scrutiny.
However, it's important to note that though these compounds hold immense utility, sound management is vital due to their potential environmental and health impact. For instance, excessive use and improper disposal of certain halogenated compounds can result in ozone layer depletion and can lead to severe health issues.
Broadening Horizons: Halogenation of Alkanes Applications
Moving beyond the regular realms of life, the halogenation of alkanes also finds its utility in niche sectors of aerospace and material science.
In the aerospace industry, halogenated alkanes like Hydrochlorofluorocarbons (HCFCs) are extensively used as foam-blowing agents in the manufacture of rigid polyurethane foams, which subsequently act as insulation materials in space vehicles. Halogenated alkanes also prove crucial in fire suppression systems, extensively used in public places like cinemas, airports, and libraries.
Moreover, in material science, halogenated alkanes find their use in the development of advanced polymers. For instance, Polyvinyl Chloride (PVC), a widely used thermoplastic polymer, is based on a halogenated alkane.
The world of analytics and environmental monitoring too isn't untouched. Methyl chloride and Methyl bromide, halogenated alkanes, serve as comparative standards in gas chromatography, a technique used to separate and analyse volatile substances. They also assist in atmospheric studies, particularly in strategies aiming to protect our ozone layer.
Application | Example |
Pharmaceutical Industry | Fluticasone, Prozac |
Agriculture | Methyl bromide (pesticide), Dicamba (herbicide) |
Refrigerants | Freon |
Aerospace Industry | HCFCs (insulation materials) |
Material Science | Polyvinyl Chloride (PVC) |
As you can see, the applications of halogenation of alkanes are multifarious and deeply interwoven into the modern-day fabric of existence. Whether it's in the pill you take to treat a headache, the radio you use to stay updated on world affairs, or the refrigerator you store your food in, remember it's the halogenation at work!
An interesting case in point is Halon 1301, a Brominated alkane, employed in spacecraft fire extinguisher systems! So, the halogenation of alkanes not just augments earthly life but accompanies human quests to the cosmological horizons.
A Closer Look at Free Radical Halogenation of Alkanes
Drilling down into the specifics of organic chemistry, we find that the free radical halogenation of alkanes is a crucial reaction mechanism. This mechanism is responsible for the replacement of hydrogen atoms in an alkane by a halogen, leading to a halogenated compound. Underscoring the chemical reactions, the principal players in this process are the reactive compounds termed free radicals.
Explore Free Radical Halogenation of Alkanes
So, what exactly is free radical halogenation of alkanes? The answer lies in understanding the key factors in play: free radicals, alkanes, and halogens.
A Free Radical is a species with an unpaired electron, making it very reactive. These arise when covalent bonds in molecules split homolytically, causing each resulting atom to retain one of the bonded pair's electrons.
In free radical halogenation, these radicals are generated from halogens either by heat or light. For instance, a chlorine molecule (\(Cl_{2}\)) can dissociate into two chlorine free radicals in the presence of UV-light:
\[ Cl_{2} \xrightarrow{UV} 2Cl^{.} \]
The dot represents the unpaired electron of the chlorine radical.
Next, Alkanes are the most basic type of hydrocarbons, made up solely of hydrogen and carbon atoms. They are characterised by single bonds between carbon atoms, making them saturated hydrocarbons. Examples include methane, ethane, propane, and butane.
Finally, Halogenation is a process involving the introduction of halogens to a substance. The halogens (Group VII elements on the periodic table) include fluorine, chlorine, bromine, iodine, and astatine.
The essence of the free radical halogenation of alkanes is a stepwise process known as a chain reaction. It comprises initiation, propagation, and termination steps. The halogenation process creates a haloalkane, where one or more hydrogen atoms attached to the carbon in an alkane is replaced by a halogen atom.
Take the halogenation of methane with chlorine, for example. This occurs in the presence of UV light and proceeds in three main steps: - Initiation: The chlorine molecule splits into two highly reactive chlorine free radicals in the presence of UV-light. \(Cl_{2} \xrightarrow{UV} 2Cl^{.}\) - Propagation: These radicals then attack a methane molecule, replacing a hydrogen atom, and creating a methyl radical and a molecule of hydrogen chloride. \(Cl^{.} + CH_{4} -> CH_{3}^{.} + HCl\) The methyl radical reacts with another chlorine molecule to create chloromethane and a new chlorine radical. \(CH_{3}^{.} + Cl_{2} -> CH_{3}Cl + Cl^{.}\) - Termination: This step involves the union of two free radicals to form a stable molecule, ending the chain reaction. \(Cl^{.} + CH_{3}^{.} -> CH_{3}Cl\) This is a perfect example of free radical halogenation of an alkane, where a hydrogen atom in methane is replaced by a halogen (chlorine) atom.
Contextualising Free Radical Halogenation
In the grand scheme of chemistry, how does free radical halogenation of alkanes fit in, and why is this process so important?
This mechanism is instrumental for understanding the behaviour of organic compounds under conditions of light or heat, which can have significant real-world implications. For example, it's used in the industrial synthesis of many compounds, including key intermediates used in the production of a variety of products, from plastics and refrigerants to pharmaceuticals.
By understanding how these reactions occur, chemists can control processes to synthesise useful substances more effectively and safely, ensuring minimal by-products and waste. Moreover, by manipulating the conditions, chemists can turn the direction of the reaction to favour one product over another. This is particularly relevant when halogenating unsymmetrical alkanes, where different halogenated products could be formed depending on the site of halogen substitution.
Consider the halogenation of propane with bromine in the presence of light, which can produce two possible products: 1-bromopropane and 2-bromopropane. The reaction with the formula for 1-bromopropane looks as follows: \(C_{3}H_{8} + Br_{2} \xrightarrow{light} C_{3}H_{7}Br + HBr\) For 2-bromopropane, the equation would be: \(C_{3}H_{8} + Br_{2} \xrightarrow{light} CH_{3}CHBrCH_{3} + HBr\) By understanding free radical halogenation, chemists can control the conditions to favour the formation of one product over the other.
Moreover, understanding free radical halogenation also provides insights into more complex chemical processes such as polymerisation, ozone layer degradation, and combustion reactions in engines. These are all underpinned by free radical mechanisms.
In a nutshell, free radical halogenation is not just a fundamental chemical reaction, it's a significant stepping stone in umpteen real-life phenomena and industrial applications. By understanding this mechanism, you glimpse the world of organic chemistry at work, painting everyday life with its molecular interactions.
Unravelling the Mechanism of Halogenation of Alkanes
Diving deeper into the chemistry of hydrocarbons, the halogenation mechanism proves to be a remarkable way to unlock new potential from simple molecules like alkanes. This process involves replacing hydrogen atoms with a halogen, fundamentally altering the properties of the original molecule. But what is the science behind this fascinating process? Let's explore together.
Shedding Light on Halogenation of Alkanes Mechanism
Halogenation of alkanes mechanism is all about the stepwise process known as a chain reaction. Now, whilst this may sound more like a musical hit from the 80s than a chemistry term, it actually describes how the halogenation takes place in discrete, interlinking steps: Initiation, Propagation and Termination.
Initiation: This step involves breaking the halogen molecule (like \(Cl_{2}\) or \(Br_{2}\)) into two free radicals. Radicals are highly reactive as a result of their unpaired electron. This splitting often happens when the molecule absorbs energy in the form of light or heat. It's called homolytic fission.
For example, in the halogenation of methane with chlorine:
\[ Cl_{2} \xrightarrow{UV} 2Cl^{.} \]
Propagation: This stage consists of two steps. Firstly, a fast reaction where the halogen free radical collides with the alkane molecule. This results in the replacement of a hydrogen atom with the halogen and forms a new radical in the process.
In the case of chlorination of methane:
\[ Cl^{.} + CH_{4} -> CH_{3}^{.} + HCl \]
The new radical (a methyl radical in this example) then collides with another halogen molecule. This results in the formation of the halogenated alkane and another halogen free radical, allowing the propagation process to continue:
\[ CH_{3}^{.} + Cl_{2} -> CH_{3}Cl + Cl^{.} \]
Termination: This final stage involves the union of two free radicals to form a stable molecule, thereby ending the chain reaction. It's worth noting, however, that this stage often produces a minor product that can also act as a reactant in the propagation stage, potentially causing a different halogenated product.
An example of the termination stage could be:
\[ Cl^{.} + CH_{3}^{.} -> CH_{3}Cl \]
A Detailed Study on Halogenation of Alkanes Mechanism
Riding deeper into the study of halogenation, it's important to realise that the three steps aren't necessarily sequential. They can and often do happen simultaneously once the chain reaction has been initiated.
Also, you might notice that the termination step seems a bit inherently contradictory. After all, wouldn't the production of more free radicals lead to an unending chain reaction? In practice, this isn't the case due to the low concentration of free radicals. The chance of two free radicals colliding and causing termination is much higher than a radical colliding with the alkane.
It's also key to note that propagation occurs twice in the cycle, once for each of the new radicals.
Another interesting aspect of this mechanism is the noticeable effect of temperature and light on the reaction. The initiation step requires an input of energy to break the bond holding the halogen molecule together. However, this energy is released in the subsequent propagation steps which are exothermic. That's why once the reaction has started, it can continue until the reactants are exhausted.
Moving on to the nature of the halogens themselves, the different halogens have varying levels of reactivity due to their different bond energies. For instance, chlorine is a more reactive halogen than bromine due to its lower bond energy. This varies the halogenation of different alkanes by different halogens.
Finally, when dealing with unsymmetrical alkanes, the position of halogenation can vary because the hydrogen atoms are not all equivalent. This leads to the formation of different products. And by manipulating conditions like temperature and the concentration of halogen, chemists can control which product is favoured.
All in all, the halogenation of alkanes mechanism describes a seemingly simple but highly intricate dance of molecules, radicals, and atoms that can be beautifully controlled to produce a wide variety of products.
What is Regioselectivity of Halogenation?
Regioselectivity is a principle that governs the orientation of chemical reactions. In the context of halogenation, regioselectivity comes into play when there are multiple potential sites for halogenation on an alkane molecule. This might happen, for example, with an unsymmetrical alkane, where different hydrogen atoms may be replaced by halogens leading to the formation of different products.
Going Deep into Regioselectivity of Halogenation
Regioselectivity essentially deals with the preference of one direction of chemical bond making or breaking over all other possible directions. In a reaction that could potentially create two or more constitutional isomers, the term 'regioselective' is used to describe situations where one isomer is preferred over others.
The rule which helps predict the regioselectivity of electrophilic addition to an alkene atom is famously known as Markovnikov's rule. In halogenation, things get a bit more complicated as different positions on the alkane can be targeted for halogenation.
Specifically, this is because not all hydrogens on an alkane molecule are equivalent. If there’s a choice, halogenation usually occurs at the most substituted carbon (the carbon bonded to the greatest number of other carbons) because it will form the most stable radical. This is largely due to the hyperconjugation effect, a type of interaction that helps stabilize the positive charge on a carbon atom and its radical.
Consider the following example, the halogenation of 2-methylpropane:
Here, we have three options:
- \(H^{a}\) hydrogen can form a primary radical (attached to one carbon).
- \(H^{b}\) hydrogen can form a secondary radical (attached to two carbons).
- \(H^{c}\) hydrogen can form a tertiary radical (attached to three carbons).
In this case, halogenation is more likely to occur at the \(H^{c}\) position, due to the resulting tertiary radical being more stable than either the primary or secondary radicals. Note, however, that while a particular product may be favoured, the others can still form, just in lower yield.
The principle of regioselectivity is crucial in the design and execution of synthetic pathways in organic chemistry, as well influencing our understanding of many biological processes including, but not limited to, stereochemistry and enzymatic pathways.
Unmasking the Mystery: Regioselectivity of Halogenation
The concept of regioselectivity, while complex, unveils another layer of intriguing intricacy in chemical reactions. Nature, it appears, has a preference — and in the bustling dance of molecule meeting molecule, certain steps are more likely to be followed than others.
The exploration of this concept leads us to the enigmatic realm of organic chemical reactions, where minute details — the positioning of atoms in a molecule, the type of radical involved — can have profound effects on the outcome of a reaction.
In the halogenation of alkanes, regioselectivity becomes apparent when the molecule offers multiple potential locations for halogen attachment. If the hydrocarbon is unsymmetrical, where the hydrogen atoms aren't equivalent, the situation becomes more interesting — different products may form.
To comprehend this, consider chlorination of propane. It could lead to the formation of either 1-chloropropane or 2-chloropropane, depending on which hydrogen atom is replaced by the chlorine. Yet, evidence shows us that 2-chloropropane is predominantly formed — highlighting the regioselectivity of halogenation.
One must ask, why is this? This is best understood by examining the stability of the intermediate radicals — the more stable the free radical, the more likely it is to form. By this rule, tertiary radicals (carbon atoms attached to three other carbon atoms) are more stable than secondary (attached to two carbons), which are more stable than primary (attached to one carbon). This preference is typically referred to as the 1° : 2° : 3° rule.
When we apply this rule to halogenation, we can realise that nature takes the path of least resistance — favouring the most stable of options.
In conclusion, regioselectivity brings an added layer of understanding to the halogenation process. It guides the reaction, swaying from one potential outcome towards another in a fascinating display of nature's preference.
Halogenation of Alkanes - Key takeaways
- Halogenation of Alkanes: Involves the replacement of hydrogen atoms in an alkane with halogens, resulting in a halogenated compound.
- Monohalogenation and Polyhalogenation: Monohalogenation involves substituting one hydrogen atom in the alkane, while polyhalogenation initiates replacement of all hydrogen atoms with halogens.
- Applications of Halogenation of Alkanes: Used extensively across industries including pharmaceuticals, agriculture, refrigeration, aerospace, material science, analytics and environmental monitoring.
- Free Radical Halogenation of Alkanes: A reaction mechanism involving free radicals generated by halogens breaking their covalent bonds through heat or light, initiating a chain reaction leading to a haloalkane.
- Regioselectivity of Halogenation: By understanding the halogenation reaction mechanism, chemists can control conditions to favour the formation of one product over another, particularly when halogenating unsymmetrical alkanes.
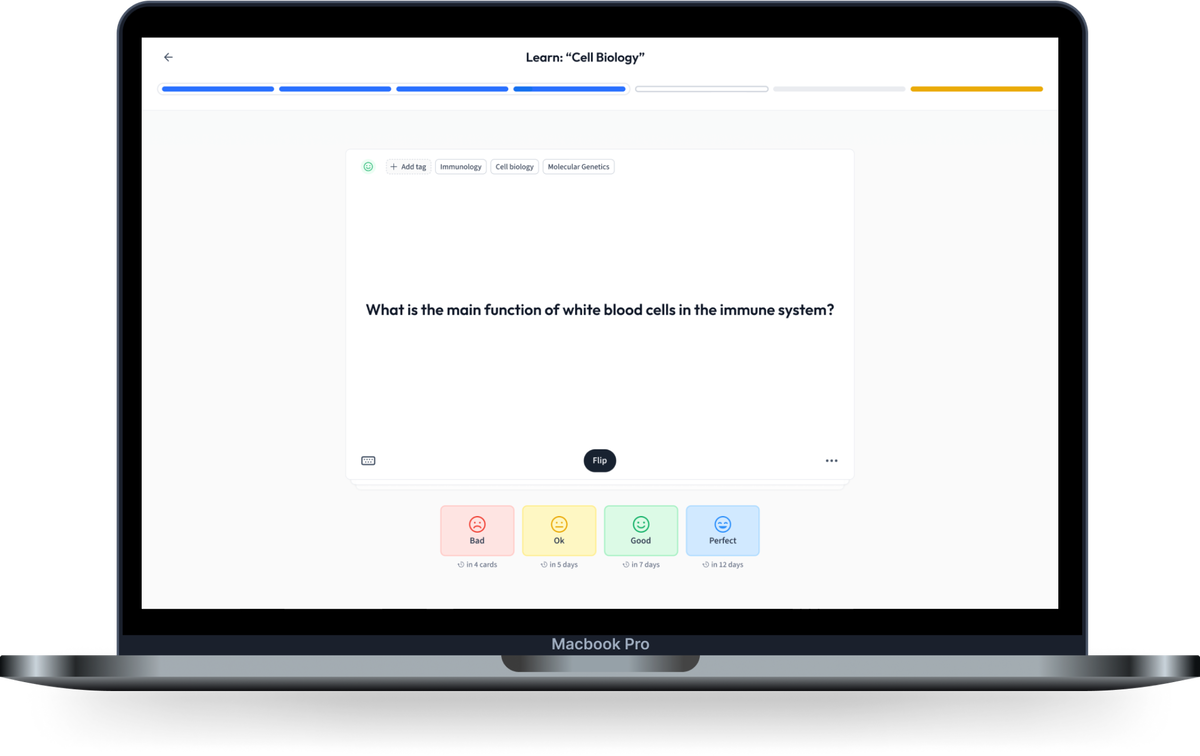
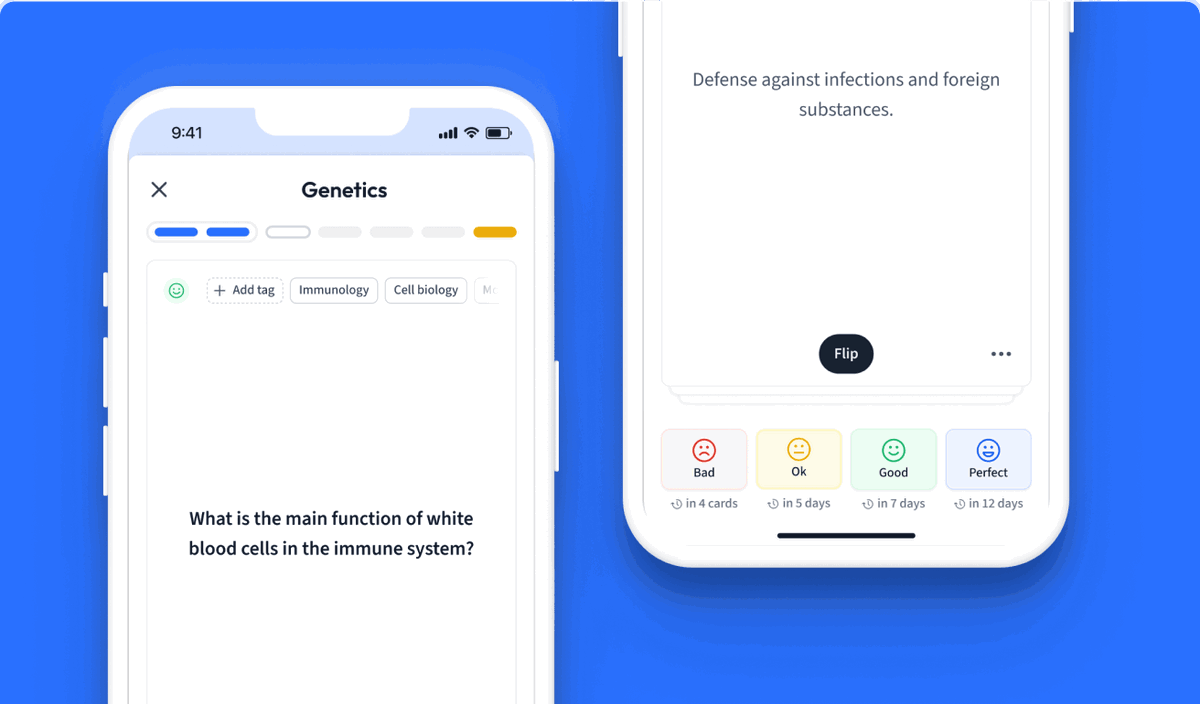
Learn with 12 Halogenation of Alkanes flashcards in the free StudySmarter app
We have 14,000 flashcards about Dynamic Landscapes.
Already have an account? Log in
Frequently Asked Questions about Halogenation of Alkanes
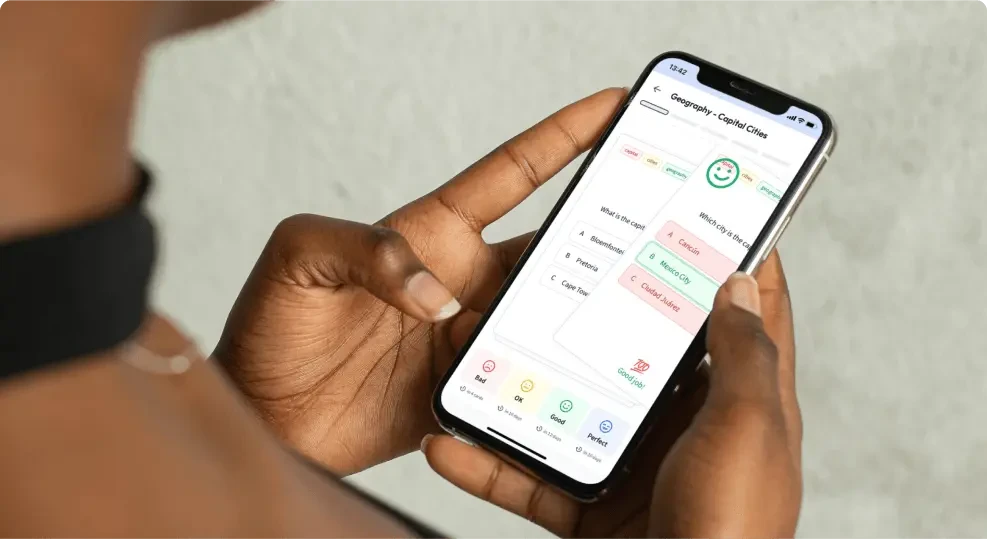
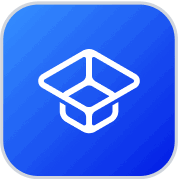
About StudySmarter
StudySmarter is a globally recognized educational technology company, offering a holistic learning platform designed for students of all ages and educational levels. Our platform provides learning support for a wide range of subjects, including STEM, Social Sciences, and Languages and also helps students to successfully master various tests and exams worldwide, such as GCSE, A Level, SAT, ACT, Abitur, and more. We offer an extensive library of learning materials, including interactive flashcards, comprehensive textbook solutions, and detailed explanations. The cutting-edge technology and tools we provide help students create their own learning materials. StudySmarter’s content is not only expert-verified but also regularly updated to ensure accuracy and relevance.
Learn more