Jump to a key chapter
Another example is formaldehyde. Correctly known as methanal, it is the most common aldehyde in industry. It has multiple uses, such as in tanning and embalming, or as a fungicide. However, we can also react it with different molecules to make a variety of more useful compounds. These include polymers, adhesives and precursors to explosives. But how do aldehydes and ketones react, and why?
- This article is about the reactions involving aldehydes and ketones.
- We will begin by looking at the polarity of the carbonyl group, C = O, and why it reacts so readily.
- We will then explore some of the common reactions of aldehydes and ketones and practice drawing their mechanisms.
- Finally, we'll end by learning how you can distinguish between aldehydes, ketones, and other organic molecules.
Why do aldehydes and ketones react?
You should remember from Aldehydes and Ketones that they both contain the carbonyl functional group , . This is a carbon atom joined to an oxygen atom by a double bond. Let's take a closer look at it.
If we compare the electronegativities of carbon and oxygen, we can see that oxygen is a lot more electronegative than carbon.
Electronegativity values of some common elements
As explored in Polarity, electronegativity is an atom's ability to attract a bonding pair of electrons towards itself. This means that the oxygen atom in the C = O bond pulls on the four bonding electrons that it shares with carbon quite strongly, tugging them closer. This makes the oxygen atom partially negatively charged and leaves the electron-deficient carbon atom partially positively charged. We can show this using the delta symbol , δ .
The carbonyl bond, shown here in methanal
The carbonyl bond is now polar. We can say that it has a permanent dipole moment. This makes it very attractive to certain molecules called nucleophiles, which we'll look at in just a second.
We can also see that aldehydes and ketones are unsaturated compounds - they contain a double bond. This means that they easily take part in addition reactions. If we put this all together, we can conclude that aldehydes and ketones often react with nucleophiles using nucleophilic addition. However, they also take part in other sorts of reactions such as oxidation and reduction, which we'll explore later.
Nucleophilic reactions of aldehydes and ketones
As mentioned above, aldehydes and ketones react with nucleophiles in nucleophilic addition reactions. Before we look at how, let's first remind ourselves of what a nucleophile is.
What is a nucleophile?
Nucleophiles are electron pair donors. They are all negatively charged or contain a partially negatively charged atom, and all have a spare pair of electrons in their outer shell.
Oppositely charged particles attract one another, so nucleophiles are attracted to positively charged areas such as the carbon atom found in the carbonyl group. Some common examples include:
- The cyanide ion, \(CN^-\)
- The hydride ion, \(H^-\)
- Water \(H_2O\)
- Ammonia, \(NH_3\)
In this article we'll focus on reactions involving the first two molecules: the cyanide and hydride ions.
Reaction with hydrogen cyanide
Aldehydes and ketones react with hydrogen cyanide and dilute hydrochloric acid to form hydroxynitriles, which are also known as cyanohydrins. This is an important reaction because hydroxynitriles contain both a hydroxyl (\(-OH\)) and a nitrile ( ) functional group, making them relatively reactive, meaning they are easily turned into other compounds. For example, we can use hydroxynitriles to make synthetic versions of amino acids.
We don't use hydrogen cyanide directly in this reaction, for hydrogen cyanide is an extremely poisonous gas. Instead we use aqueous potassium or sodium cyanide. In solution, this produces cyanide ions and hydrogen cyanide.
Let's look at the general reaction between an aldehyde and cyanide ion.
The general reaction between an aldehyde and the cyanide ion
- The negative cyanide ion is attracted to the partially positively charged carbon in the C=O bond. It forms a bond with this carbon and forces the C=O double bond to break, transferring one of the bonding pairs of electrons to the oxygen atom.
- The oxygen atom is now a negatively charged ion with a lone pair of electrons. It reacts with a positive hydrogen ion from the solution to form a hydroxyl functional group, -OH.
- The end product is a hydroxynitrile.
The reaction has the following general equation: \(RCHO + HCN \rightarrow RCH(OH)CN\)
To see how ketones react with cyanide ions, we simply replace the hydrogen atom bonded to the carbonyl group with another organic R group, as you can see in the following mechanism:
Nucleophilic addition of a ketone
Let's now apply that to a named aldehyde, ethanal.
Nucleophilic addition of ethanal using the cyanide ion
Remember to include all partial charges and lone pairs of electrons when drawing organic mechanisms.
Because the product of this reaction contains two functional groups, both the nitrile group and the hydroxyl group, we use the prefix hydroxy and the suffix nitrile to name the molecule. The carbon atom attached to the nitrogen atom is always labeled carbon. 1. Here we can see that the alcohol functional group is joined to carbon 2, and so we call this molecule 2-hydroxypropanenitrile.
2-hydroxypropanenitrile
You might have noticed that we started with ethanal, which contains a carbon chain that is two atoms long, and have ended up with a carbon chain three atoms long. This is because we reacted ethanal with the cyanide ion, which contains an additional carbon atom. Remember to include this when naming your product. For further information on nomenclature, check out Organic Compounds for more detail on naming conventions.
We can also react potassium cyanide with the ketone butanone, as shown below:
Nucleophilic addition of butanone using the cyanide ion
Look at the product. It still has a chain that is four carbon atoms long. But counting the carbon attached to the nitrogen as carbon 1, you can see that there is now both a methyl group and a hydroxyl group joined to carbon 2. We therefore name this molecule 2-hydroxy-2-methylbutanenitrile.
2-hydroxy-2-methylbutanenitrile
Isomeric products of nucleophilic addition
Let's look more closely at the previous example. If we react potassium cyanide with butanone, we can actually get two different products. This is because the cyanide ion can attack from either above or below the planar molecule. Both mechanisms are shown below for comparison.
Second mechanism for the nucleophilic addition of butanone
Second mechanism for the nucleophilic addition of butanone
Look at the two products. They are optical isomers of each other.
Optical isomers are molecules with the same structural formulas that differ in their arrangement of atoms around a chiral centre. A chiral centre is a carbon atom bonded to four different groups.
Optical isomers are also known as enantiomers. They are produced because the cyanide ion can attack from above or below the plane, as shown in the mechanisms above. The ion is equally as likely to attack from above as below, so the reaction produces a 50:50 mixture of the two isomers, known as a racemic mixture.
The two isomeric products of the reaction between butanone and the cyanide ion. They are optical isomers, known as enantiomers
For further information on isomers, check out Optical Isomerism.
Reduction of aldehydes and ketones
When you react aldehydes and ketones with a reducing agent which supplies the hydride ion , you get an alcohol. This is also a type of nucleophilic addition reaction but is more often known as reduction.
Common reducing agents include sodium tetrahydridoborate (III) in an aqueous solution. That is a bit of a mouthful to remember but it is actually just the molecule . It is also known as sodium borohydride.
Sodium borohydride
Reducing an aldehyde produces a primary alcohol and reducing a ketone produces a secondary alcohol. You might have realised that this is the opposite reaction to oxidisng alcohols, as explored in Oxidation of Alcohols. Oxidising a primary alcohol produces an aldehyde whilst oxidising a secondary alcohol produces a ketone.
Remember that a primary alcohol has zero or one R groups attached to the carbon with the hydroxyl functional group , whereas a secondary alcohol has two attached R groups.
Let's take a look at what happens when we reduce the aldehyde ethanal. It is very similar to the nucleophilic addition mechanism we looked at earlier.
The reduction of ethanal
- The hydride ion acts as a nucleophile and attacks the partially positive carbon atom in the C=O bond. The C=O bond breaks and one of the bonding pairs of electrons is transferred to the oxygen atom, forming a negative oxygen ion with a spare pair of electrons.
- The oxygen atom’s spare pair of electrons is attracted to a positive hydrogen ion from the solution and forms the hydroxyl functional group, -OH.
- The product is a primary alcohol.
We can represent this reaction using [H] to show the reducing agent:
$$ RCHO + 2[H] \rightarrow RCH_2OH $$
The general equation for the reduction of a ketone is given below. Notice how it has two R groups attached to the carbon:
$$ RCOR' + 2[H] \rightarrow RCH(OH)R' $$
Isomeric products of reduction
Reducing a ketone can produce optical isomers in the same way as earlier in the article. However, reducing aldehydes does not produce optical isomers. This is because reduction of an aldehyde forms a primary alcohol. Primary alcohols have at least two hydrogen atoms bonded to the carbon containing the -OH group. To be a chiral centre, a molecule must have four different groups bonded to a carbon atom and here this clearly isn't the case. Therefore, primary alcohols don't show optical isomerism.
A primary alcohol
Oxidation of aldehydes
One way of creating aldehydes in the lab is by heating a primary alcohol with acidified potassium dichromate (VI). This oxidises the alcohol. If you distill the product off you get an aldehyde, but if you instead use reflux, the aldehyde will react further. It is oxidised again and forms a carboxylic acid.
You don't need to know the mechanism for this reaction, but remember to use [O] to represent the oxidising agent in the equation.
For example, oxidising ethanal produces ethanoic acid.
$$ CH_3CHO + [O] \rightarrow CH_3COOH $$
Carboxylic acids are organic molecules that contain both the hydroxyl functional group, , and the carbonyl functional group, . Collectively, these two functional groups are known as the carboxyl group. Even the word itself is just a mash-up of hydroxyl and carbonyl !
Carboxylic acids are weak acids. One common example is citric acid, an organic compound with three carboxyl groups. You'll learn more about carboxylic acids in Carboxylic Acids.
Ketones don't react in this way - they can't be oxidised further because an oxidation reaction would involve breaking one of their strong CC bonds. This fact can be used to tell aldehydes and ketones apart with a number of different reagents.
Potassium dichromate (VI)
Oxidising an aldehyde with potassium dichromate (VI) turns the solution from orange to green. However, there will be no colour change with a ketone.
The reaction of acidified potassium dichromate (VI) with a ketone (left) and an aldehyde (right)
Tollens' reagent
Tollens' reagent is also known as the silver mirror test. It contains the diaminesilver (I) ion, . If you warm a ketone with Tollens' reagent, the solution will remain colourless, but adding an aldehyde will form a metallic silver mirror deposit on the sides of the test tube.
The reaction between Tollens' reagent and a ketone (left) and an aldehyde (right)
Fehling's test
Fehling's solution is made up of two other solutions called Fehling's A and Fehling's B. The solution is naturally blue. If you heat it with an aldehyde, you'll form a brick red precipitate. This tends to turn the whole solution red. However, warming with a ketone gives no colour change.
Reacting Fehling's solution with a ketone (left) and an aldehyde (right)
The following table should help summarise your knowledge of the different oxidation reactions you can use to tell aldehydes and ketones apart.
Testing for aldehydes and ketones
Distinguishing between molecules
Potassium dichromate is a much stronger oxidising agent than Tollens' reagent and Fehling's solution. It is also able to oxidise alcohols, as we mentioned earlier. This means it can help us distinguish between alcohols, aldehydes, and ketones.
Add three drops of an unknown aldehyde, ketone, or alcohol to a test tube that contains acidified potassium dichromate (VI). Warm gently in a water bath and observe any changes.
- If the solution remains orange, your unknown compound is a ketone.
- If the solution turns green, it could be either an alcohol or an aldehyde.
Test your unknown compound further by adding three drops to Tollens' reagent or Fehling's solution. In the presence of an aldehyde, Tollens' reagent will form a metallic silver mirror on the sides of the test tube and Fehling's solution will go from blue to brick red. However, these oxidising agents aren't strong enough to oxidise an alcohol so you won't see any change.
Reactions of Aldehydes and Ketones - Key takeaways
- Aldehyde and ketones all contain the carbonyl group, . This has a polar bond and is often attacked by nucleophiles in nucleophilic addition reactions.
- Aldehydes and ketones react with cyanide ions in acidic solution to form hydroxynitriles. These can show optical isomerism.
- Aldehydes and ketones are reduced by reducing agents such as to form primary and secondary alcohols respectively.
- Aldehydes can be oxidised to form carboxylic acids. However, ketones cannot as this would require breaking a strong CC bond.
- To distinguish between aldehydes and ketones we can use acidified potassium dichromate (VI), Tollens' reagent or Fehling's solution.
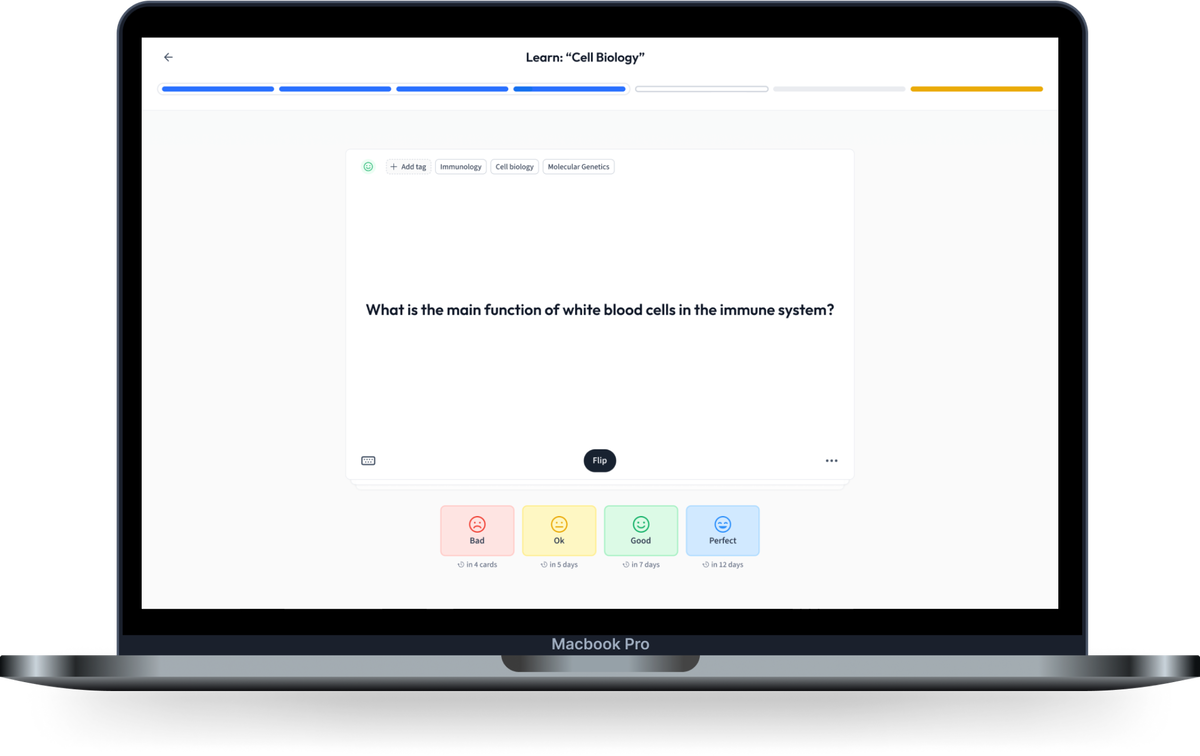
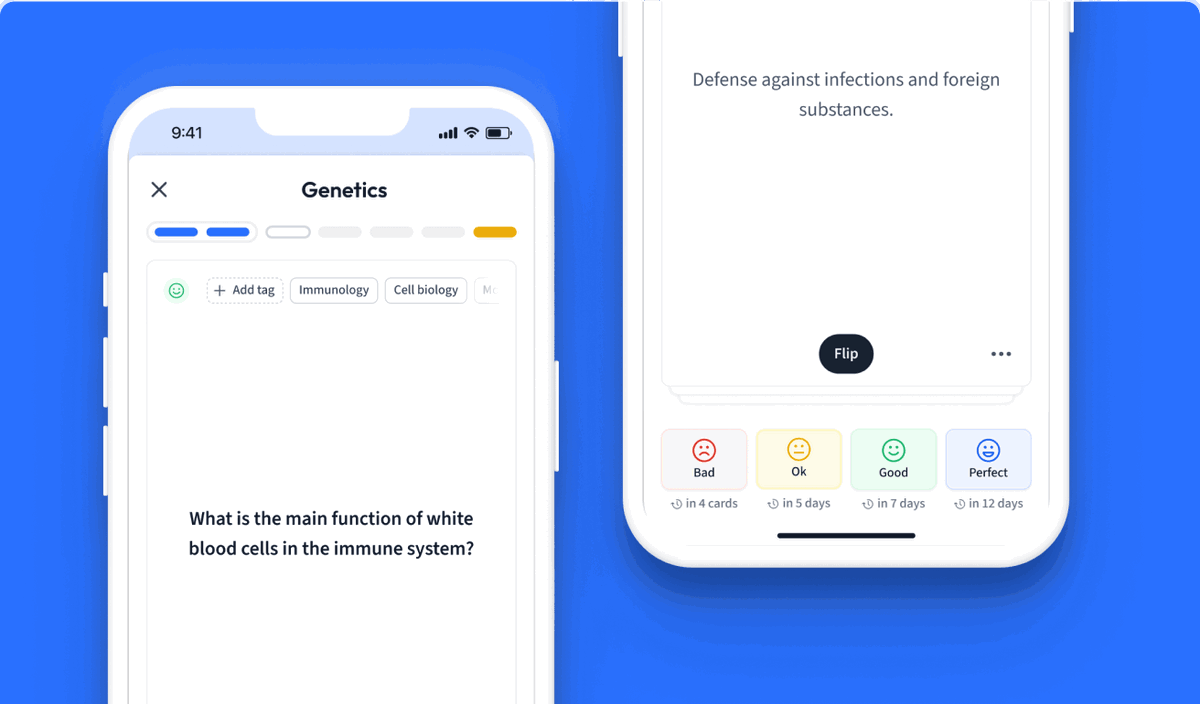
Learn with 9 Reactions of Aldehydes and Ketones flashcards in the free StudySmarter app
Already have an account? Log in
Frequently Asked Questions about Reactions of Aldehydes and Ketones
What are the reactions involving aldehydes and ketones?
Aldehydes and ketones react in a variety of reactions. For examples, they react with hydrogen cyanide to form hydroxynitriles. They can also be reduced to give primary and secondary alcohols. In addition, you can oxidise aldehydes to give carboxylic acids. However, you can't oxidise ketones.
What are the reactions of ketones?
Ketones react with hydrogen cyanide to form hydroxynitriles and can be reduced using sodium tetrahydridoborate to form secondary alcohols.
Which reaction will distinguish between ketones and aldehydes?
There are several different reactions you can use to distinguish between aldehydes and ketones. You can use potassium dichromate (VI), for example. Aldehydes turn the solution from orange to green, but ketones give no visible reaction. Aldehydes also react with Fehling's solution, forming a brick red precipitate. However, with ketones the solution remains blue. Similarly, aldehydes react with colourless Tollens' reagent to form a metallic silver deposit on the sides of the test tube but ketones produce no visible change.
What is the name for the general reaction of aldehydes and ketones?
There isn't a general name for the reactions of aldehydes and ketones. They can react in a variety of different ways. For example, they can both be reduced. This article will take a look at some of their different reactions.
How do you distinguish between aldehydes and ketones in one test?
You can distinguish between aldehydes and ketones in a few different ways. Aldehydes turn potassium dichromate (VI) from orange to green but ketones produce no visible reaction. Aldehydes will also react with blue Fehling's solution, forming a brick red precipitate, but ketones won't react at all. Likewise, aldehydes react with colourless Tollens' reagent to form a silver mirror deposit on the sides of the test tube, but ketones again produce no visible reaction.
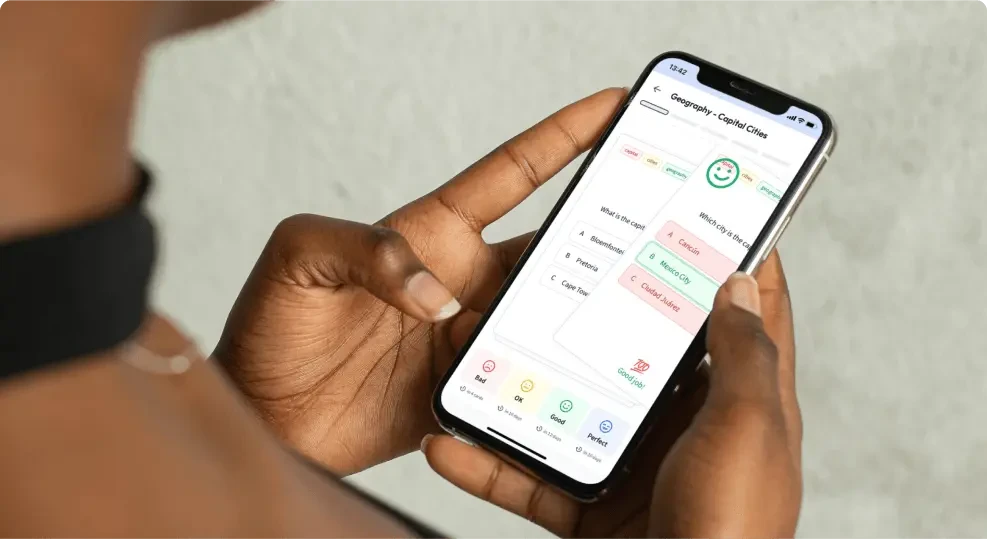
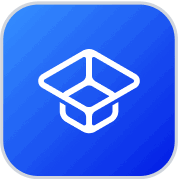
About StudySmarter
StudySmarter is a globally recognized educational technology company, offering a holistic learning platform designed for students of all ages and educational levels. Our platform provides learning support for a wide range of subjects, including STEM, Social Sciences, and Languages and also helps students to successfully master various tests and exams worldwide, such as GCSE, A Level, SAT, ACT, Abitur, and more. We offer an extensive library of learning materials, including interactive flashcards, comprehensive textbook solutions, and detailed explanations. The cutting-edge technology and tools we provide help students create their own learning materials. StudySmarter’s content is not only expert-verified but also regularly updated to ensure accuracy and relevance.
Learn more