Jump to a key chapter
Introduction to Regioselectivity
Regioselectivity, a term frequently encountered in the field of organic chemistry, plays a crucial role in determining the outcome of chemical reactions. Its impact on how molecules interact and combine in chemical reactions cannot be overstated. As such, you need to comprehend what it is and how it influences chemical reactions.
Defining Regioselectivity: A Closer Look
Regioselectivity refers to the preference of one direction of chemical bond making or breaking over all other possible directions. It manifests when a chemical reaction could potentially occur in multiple ways (producing different products), but one particular way is substantially more probable than others.
simplistic example, consider a scenario where you have two types of fruit: apples and oranges. Now, let's say you're more inclined to pick an apple than an orange. In chemistry terms, your 'fruit-selectivity' leans more towards apples. This is essentially what happens during a chemical reaction that exhibits regioselectivity.
In more technical terms, if a reaction could occur at two different functional groups in a molecule, but predominantly occurs at one of these groups, this is an illustration of regioselectivity. This preference is usually a result of various factors, including sterics, electronics, and protection/deprotection strategies.
- Ortho-, meta-, and para- director
- Markovnikov's and anti-Markovnikov's rule
The Importance of Regioselectivity in Organic Chemistry
Regioselectivity plays a critical role in organic chemistry for a variety of reasons:- Product predictability: A profound understanding of regioselectivity can help predict the major and minor products of a reaction, helping chemists to devise the appropriate reaction pathway.
- Efficiency and yield optimization: Regioselectivity aids in increasing the yield of the desired product, reducing the production of waste.
- Pharmacological implications: In pharmaceutical chemistry, even a minor modification in the molecule's structure can result in a drastic change in the drug's properties. Hence, regioselectivity is essential in designing and developing effective pharmaceuticals.
Regioselectivity vs. Chemoselectivity
When discussing selectivity in organic chemistry, two strikingly significant concepts crop up: regioselectivity and chemoselectivity. Although they may seem somewhat akin, there lie essential disparities between the two.
Understanding the Difference Between Chemoselectivity and Regioselectivity
Chemoselectivity refers to the propensity of a reagent to react preferentially with one functional group in the presence of other functional groups. Suppose you have a molecule with different functional groups, and a reagent reacts with only one of these groups selectively, that is an exhibit of chemoselectivity. It's more about the "type" of reactions occurring.
On the other hand, regioselectivity is concerned about the region (or location) within a single functional group where the reaction transpires. It's less about the type of reaction and more about the site of reaction within a certain type of functional group.
RCHO + H- -> RCH2OH RCOOR' + H- -> RCH2OOR'
Here, the reagent (H-) is chemoselective because it opts to react with the carbonyl group over other potential functional groups. Meanwhile, the reaction is regioselective because the hydride (H-) is added to the carbon of the carbonyl group rather than the oxygen.
Regioselective and Chemoselective Reactions: A Comparative Overview
Delving deeper into the comparison of regioselective and chemoselective reactions, it's key to consider examples that illustrate the principles in action.
If we take hydroboration-oxidation reaction of an alkene as an example:
RCH=CH2 + BH3 -> RCH2–CH2–BH2
In this reaction, borane (BH3) adds across the carbon-carbon double bond in an anti-Markovnikov manner—a perfect example of regioselectivity. The hydrogen and boron add to the different carbons of the alkene and not to the same carbon (which would represent a non-regioselective process).
RCHO + Nu- -> RCH(Nu)OH
In this reaction, the nucleophile (Nu-) might potentially add to either the alkene or the carbonyl group. However, because the carbonyl group is generally more electrophilic, the nucleophile adds to the carbonyl carbon selectively, illustrating chemoselectivity.
Major Chemical Reactions Showing Regioselectivity
Understanding the role of regioselectivity in chemical reactions is key for anyone studying or working in the field of organic chemistry. Below we'll delve deeper into this pivotal concept, exploring how it operates in some major chemical reactions such as Birch reduction, electrophilic aromatic substitution, Heck reaction, and hydroboration.
Birch Reduction Regioselectivity: A Detailed Analysis
The Birch reduction, named after the Australian chemist Arthur Birch, is a powerful organic reaction involving the reduction of aromatic rings in the presence of alkali metals, such as lithium, and liquid ammonia. In the context of regioselectivity, the Birch reduction is well known for its unique pattern.
In a Birch Reduction, a typical aromatic ring like benzene, upon reaction with lithium in liquid ammonia, is reduced to a non-aromatic diene, as shown below:
C6H6 + 2 Li + 2 NH3 -> C6H4 + 2 LiNH2 + H2
Importantly, the Birch reduction demonstrates stark regioselectivity. When a mono-substituted benzene undergoes Birch reduction, the incoming electrons will avoid the carbon atom connected to the substituent group (if possible) leading to its unique 1,4-cyclohexadiene product.
Regioselectivity in Birch reduction, essentially, is governed by two rules. First, electron-donating groups (EDGs) direct incoming electrons to the ortho and para positions, with para being slightly preferred. Second, electron-withdrawing groups (EWGs) steer incoming electrons away, directing them to the meta position.
For instance, if toluene (methylbenzene) undergoes Birch reduction, the two hydrogens are added to the carbon atoms ortho to the methyl (CH3) group – a clear consequence of the regioselectivity in Birch reduction.
Regioselectivity of Electrophilic Aromatic Substitution: An Examination
In the world of organic chemistry, the electrophilic aromatic substitution (EAS) holds a significant place. It involves the substitution of an atom (most commonly a hydrogen) attached to an aromatic system by an electrophile. Despite its apparent simplicity, EAS demonstrates exciting regioselectivity, primarily dictated by the type of substituents already present on the aromatic ring.
When you delve deeper, it becomes apparent that EAS regioselectivity is influenced by two types of substituents:
- Those that donate electron density into the ring (called activating groups), encouraging ortho/para substitution.
- Those that pull electron density out of the ring (deactivating groups), promoting meta substitution.
Electron-releasing groups, such as –OH and –NH2, are called 'ortho/para directors' because they steer the incoming electrophile to the carbon atoms ortho or para to them. Electron-withdrawing groups, like –NO2 and –COOH, are 'meta directors' and guide the new electrophile to the carbon meta to the substituent.
Suppose, nitration of toluene, a typical EAS, yields more of the ortho and para products in comparison to the meta product, displaying how the substituent (–CH3 in this case), an ortho/para director, influences the outcome through regioselectivity.
Heck Reaction Regioselectivity: A Comprehensive Look
The Heck reaction (or Mizoroki–Heck reaction) facilitates the creation of a new carbon-carbon bond between an alkene and an alkyl halide in the presence of a base and a palladium catalyst. Regioselectivity in the Heck reaction is intensely interesting due to its dependence on the nature of the alkene and the ligand attached to the palladium.
In a conventional Heck reaction, the palladium catalyst initially forms a complex with the alkene, and then migrates to the alkyl group, tying them together. This migration step is where regioselectivity comes into play.
For certain alkenes, the substituents dictate the orientation of the product. Two rules help predict the product:
1. When an alkene has differently substituted carbons, the new group is more likely to end up at the less substituted end (the "Heck rule"). 2. When the palladium complex bears bulky phosphine ligands, the new group typically adds to the more substituted end (an exception to the Heck rule).
Hydroboration Regioselectivity: An In-Depth Study
Hydroboration–oxidation reaction is a two-step organic reaction that transforms an alkene into an alcohol. The hydroboration step is intriguing as it showcases anti-Markovnikov regioselectivity – an unusual behaviour in the context of alkene addition reactions.
In hydroboration, borane (BH3) or a related boron compound adds across the carbon-carbon double bond of an alkene. Here's the scripted equation for it:R2C=CR2 + BH3 -> R2CH–CH2–BHR2The boron atom is added to the less substituted carbon (the carbon with more hydrogen atoms), and the hydrogen to the more substituted carbon—an example of 'regiochemical reversal' or anti-Markovnikov selectivity. The regioselectivity of hydroboration can be rationalised by considering the transition state of the reaction. In the transition state, the boron atom forms a partial bond with the less hindered carbon, leading to the observed regioselectivity. Alishing hydroboration's regioselectivity ultimately furnishes an effective strategy for converting alkenes into anti-Markovnikov alcohols—a widely useful functionality in organic chemistry.
The Role of Regioselectivity in Diels Alder Reaction
Diels–Alder reaction, named after its discoverers, Otto Diels and Kurt Alder, is a cornerstone in organic chemistry. Regioselectivity plays a crucial role in Diels-Alder reaction due to the diversity of dienes and dienophiles that can be employed and the different ways they can connect during the reaction.
Diels Alder Regioselectivity: Key Considerations
The Diels–Alder reaction allows the creation of six-membered rings by a concerted, cyclic reaction between a conjugated diene and an alkene or alkyne (known as the dienophile). The process is known for its high regioselectivity, which is governed by the electronic and steric properties of the reactants.
Regioselectivity in Diels-Alder reactions reveals itself when either the diene or the dienophile bear more than one substituent:
1. There are two types of dienes–s-cis and s-trans, referring to the relative positions of the two double bonds. 2. On the dienophile side, we can have electron-withdrawing substituents (ferrocene) and electron-donating substituents (anisole).
In forming the new six-membered ring, the substituents on the diene and dienophile can orient themselves in two ways, referred to as "endo" and "exo".
The 'endo rule' posits that the major product of a Diels-Alder reaction will be the one in which the substituents on the dienophile are pointed towards the electron cloud of the diene in the transition state—closer to the new forming bond and hence shielded. The 'exo product', on the other hand, is where these substituents are pointed away from the diene.
However, the endo preference doesn't dictate the course of all Diels-Alder reactions, particularly when sterics come into play. Substituent size can influence the outcome of a Diels-Alder reaction - large substituent groups on the diene or dienophile can lead to a preference for the exo product. In general, sterically less crowded transition states are favoured.
It's worth noting that while the endo product is often the kinetic product (it forms faster due to overlap of π systems in the transition state), it may not necessarily be the thermodynamic product (the product that is more stable). This is an interesting point in the study of regioselectivity in the Diels-Alder reaction.
To illustrate regioselectivity in Diels–Alder reaction, consider an example of a reaction between cyclopentadiene and maleic anhydride. This dienophile has two possible reactive ends, but the reaction occurs with high regioselectivity to deliver the product where the anhydride resides in the endo orientation.
C5H6 + C4H2O3-> C9H8O3
The resulting product showcases the "endo rule" as the newly formed six-membered ring has the bulky anhydride group oriented towards the electron cloud of the diene.
Understanding regioselectivity in Diels–Alder reactions is an essential part of mastering the reaction. Consideration of electronic effects, steric factors, and transition state geometries all contribute to foreseeing reaction outcomes, furthering the fundamental understanding of organic synthesis as a whole.
Practical Examples of Regioselectivity
Turning our attention from chemical reactions exclusively found in laboratories, let's highlight everyday situations where regioselectivity plays out, often unnoticed. Such examples are instrumental in demystifying the abstract nature of regioselectivity for you.
Regioselectivity Examples in Everyday Chemical Reactions
It might surprise you, but examples of regioselectivity can be found as close as your kitchen, the garden, or even inside your body. Atmospheric conditions, plant metabolism, and biological processes all mirror the regioselective principles akin to those seen in chemical laboratories.
Here are some everyday chemical reactions where regioselectivity is a critical determinant:
- Oxidation of Fats and Oils: In fats and oils, oxidation on unsaturated fatty acids preferentially occurs at the carbon atoms immediately next to double bonds, primarily due to the higher reactivity of these carbons. This regioselectivity eventually leads to rancidity, deteriorating the quality of the food.
- Photosynthesis: Crucial to life on earth, photosynthesis follows a strict regioselectivity in its light-dependent reactions. More specifically, the addition of water at the carbon atom in the reaction centre is strictly controlled; a principle of regioselectivity.
- Enzyme Activity: Inside the body, enzymes are the ultimate agents of regioselective control. They precisely catalyse biochemical reactions, picking only a specific site for reaction on a particular molecule, based on its structure and orientation.
Briefly, regioselectivity in enzymes emerges from their active sites, a cavity built up by amino acid residues, whose arrangement sieves out all but the intended reactant.
For instance, cytochrome P450, a superfamily of enzymes, is responsible for metabolising a plethora of endogenous compounds and drugs. Its regioselectivity, dictated by the structure of its active site, plays a vital role in detoxifying harmful substances in the body and activating drugs.
Recognising Regioselectivity: Real-Life Cases and Scenarios
The importance of regioselectivity permeates the practical world, from the industry down to everyday life. Recognising its effects aids in making sense of how things work in a range of scenarios, including:
- Pharmaceuticals: Regioselectivity is of critical importance in medicine, where even a slight deviation can render a drug ineffective or cause harmful side effects. For example, the anticoagulant drug warfarin—and its regioisomers— has different effects based on its regiochemistry.
- Agriculture: In farming, regioselectivity is exhibited in the synthesis and action of herbicides. Glyphosate, a commonly used herbicide, relies on its regioselective action on plant enzymes to aid in weed control.
- Brewing Beer: In beer brewing, the enzyme isomerases act regioselectively on hops to transform alpha-acids into iso-alpha-acids that contribute to the beer's bitter taste.
Recognising regioselectivity equips us to appreciate and manipulate chemical behaviour for desired outcomes. While these examples provide a brief glimpse into the myriad ways regioselectivity can manifest, they should help in understanding the extent to which regioselectivity is inextricably woven into the fabric of our everyday existence.
Regioselectivity - Key takeaways
- Regioselectivity: This concept in chemistry refers to the preference of chemical reactions to occur at one position over another within a molecule. It's pivotal in various reactions like Birch reduction, electrophilic aromatic substitution, Heck reaction, and hydroboration.
- Difference between Regioselectivity and Chemoselectivity: Chemoselectivity is about the reagent's preference for one functional group in the presence of others, whereas regioselectivity is about where the reaction occurs within a functional group.
- Birch Reduction Regioselectivity: In this reaction, regioselectivity is governed by two rules. Electronic-donating groups direct incoming electrons to the ortho and para positions, and electronic-withdrawing groups steer the electrons away to the meta position.
- Heck Reaction Regioselectivity: It relies on both the nature of the alkene and the ligand attached to the palladium. The Heck rule states that when an alkene has differently substituted carbons, the new group is more likely to end up at the less substituted end.
- Regioselectivity in Diels-Alder reaction: Regioselectivity is governed by the electronic and steric properties of the reactants. It also follows the 'endo rule', wherein the major product of a Diels-Alder reaction will be the one where the substituents on the dienophile are pointed towards the diene's electron cloud in the transition state.
Learn faster with the 15 flashcards about Regioselectivity
Sign up for free to gain access to all our flashcards.
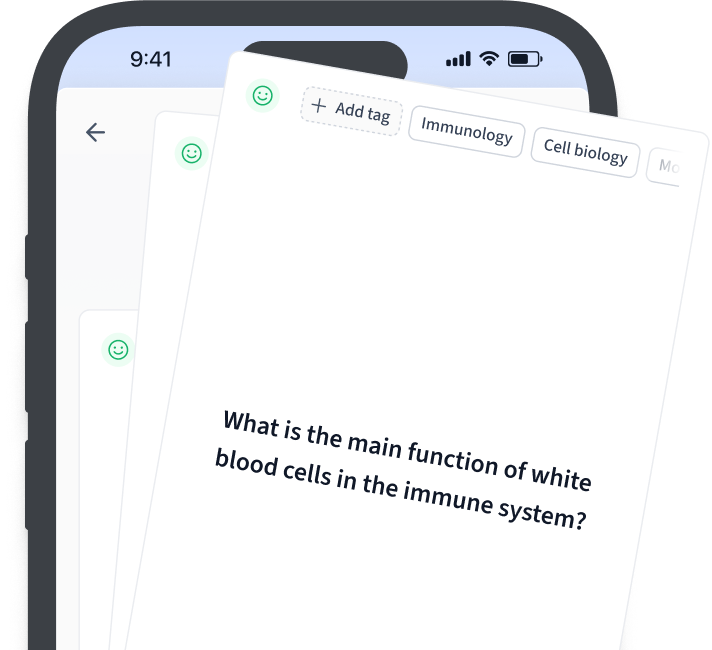
Frequently Asked Questions about Regioselectivity
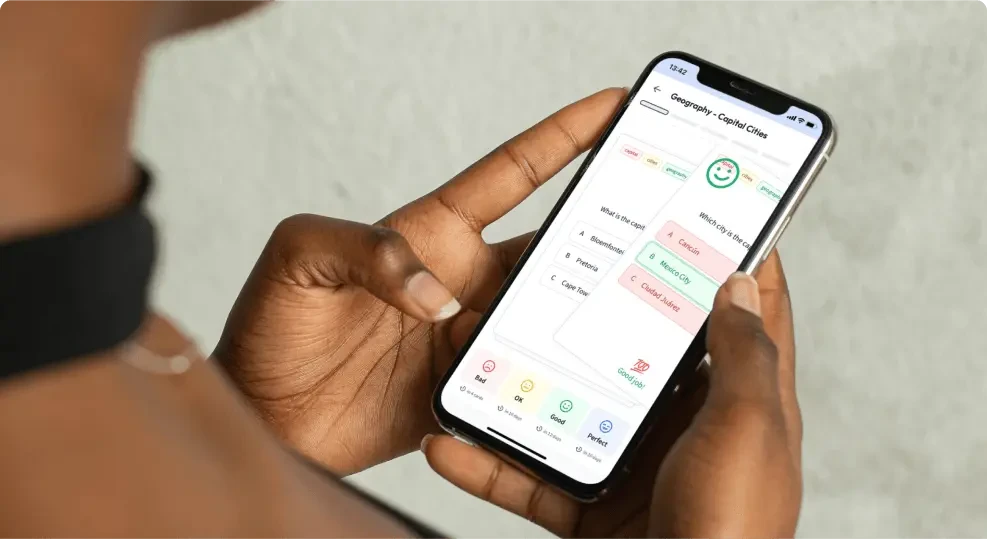
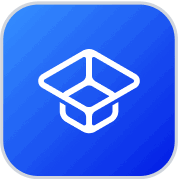
About StudySmarter
StudySmarter is a globally recognized educational technology company, offering a holistic learning platform designed for students of all ages and educational levels. Our platform provides learning support for a wide range of subjects, including STEM, Social Sciences, and Languages and also helps students to successfully master various tests and exams worldwide, such as GCSE, A Level, SAT, ACT, Abitur, and more. We offer an extensive library of learning materials, including interactive flashcards, comprehensive textbook solutions, and detailed explanations. The cutting-edge technology and tools we provide help students create their own learning materials. StudySmarter’s content is not only expert-verified but also regularly updated to ensure accuracy and relevance.
Learn more