Jump to a key chapter
Understanding the SN2 Reaction
The second-order nucleophilic substitution, or SN2 reaction, represents one of the core concepts in organic chemistry. SN2 stands for 'Substitution Nucleophilic Bimolecular' reaction. SN2 reactions are characterised by their singular step involving a concerted reaction: both the bond-breaking and bond-forming occur simultaneously.
SN2 Reaction Meaning and Basics
The term "SN2" decodes to mean 'Substitution Nucleophilic Bimolecular': 'Substitution' indicates the replacement of an atom or a group of atoms, 'Nucleophilic' refers to the nucleus-loving nature of the reactant species known as the nucleophile, and 'Bimolecular' highlights that two molecular entities are involved simultaneously in a given step of the reaction.
It's fascinating to note that the reaction rate of SN2 reactions is influenced by both the nucleophile and substrate. This is why, in terms of reaction kinetics, the rate equation is given as: rate = k[Nucleophile][Substrate], where k is the rate constant.
- The reaction takes place in a single step, with no intermediates.
- Since the reaction involves a nucleophile and substrate simultaneously, it is described as a 'concerted' reaction.
- A fundamental feature of the SN2 reaction is backside attack, which inverses the stereochemistry of the chiral center.
Detailed Overview of SN2 Reaction Mechanism
During an SN2 reaction, the nucleophile performs a "backside attack" on the substrate's carbon attached to the leaving group. This simultaneous breaking and forming of bonds produces an inversion of stereochemistry, famously referred to as the 'Walden inversion'. First, let's understand the steps in a simple tabular representation:1. Approach of Nucleophile | The negatively charged nucleophile approaches the substrate molecule from the side opposite to the leaving group. |
2. Transition State | The nucleophile begins to form a bond with the substrate leading to a pentacoordinate transition state, while the bond with the leaving group starts to break. |
3. Exit of Leaving Group | The bond between the substrate and the leaving group breaks completely, and the leaving group departs with the pair of electrons. |
How the SN2 Reaction Mechanism Works
Taking a deeper look, you will see that the landscape of the SN2 reaction is not without its peaks and valleys. Consider this example:Imagine a simple SN2 reaction where hydroxide (OH-) is the nucleophile and bromomethane (CH3Br) is the substrate, with bromine acting as the leaving group. As the hydroxide approaches the carbon from a trajectory 180 degrees opposite the bromine, the nucleophile begins to form a bond with carbon, the transition state is reached wherein carbon is momentarily connected to five things. The bond with bromine weakens and soon breaks, and bromide ion (Br-) leaves. The product is methanol (CH3OH), and the configuration at carbon is inverted.
Unveiling Examples of SN2 Reaction
A handy way to understand the SN2 reaction mechanism is to examine some practical, real-life examples.
Relatable SN2 Reaction Examples in Everyday Life
Even though it might not be immediately apparent, you're quite likely experiencing the results of an SN2 reaction on a regular basis. Brace yourself as we will dive deeper into the SN2 reactions happening around you every day.
A popular instance of an SN2 reaction is in the formation of soaps through saponification. In this process, a triglyceride (fat) reacts with an alkali base, often sodium or potassium hydroxide. The result is glycerol and salts of fatty acid, which is soap!
Triglyceride + Alkali -----> Glycerol + Soap
If you've ever done a home experiment to make soap, you've observed the SN2 reaction process in action!
Industrial SN2 Reaction Examples
Even in the industrial context, SN2 reactions find wide applications that greatly contribute to our modern life.
An important industrial process that employs SN2 reaction mechanism is the methylation of phenol to anisole. This reaction is catalysed by dimethyl sulfate, and due to the SN2 reaction mechanism, it runs smoothly under mild conditions. Anisole is subsequently used in perfumery owing to its pleasant, ether-like smell.
Phenol + Dimethyl Sulfate -----> Anisole + Sulfuric Acid
Note, the formation of Anisole via SN2 is a process that not only happens in the lab but also on a huge industrial scale!
The formation process of Paracetamol essentially involves an intermediate reaction where acid chloride (ethanoic anhydride) is treated with an amine (p-aminophenol). The nitrogen in the amine, a strong nucleophile, reacts with the carbonyl carbon in the acid chloride, displacing the chloride in an SN2 fashion.
Practical Applications of SN2 Reaction
In the realm of practical chemistry, the SN2 reaction holds a key role for a wide range of applications. Its versatility makes it invaluable in industries such as pharmaceuticals, agriculture, and environmental science. Additionally, it’s an essential part of academic and research studies in organic chemistry.
Role of SN2 Reaction in Drug Synthesis
The pharmaceutical industry relies heavily on SN2 reactions, specifically in the making of vital medicines. The SN2 mechanism is leveraged to construct complex drug molecules via breakdown or combination of simpler substances. The factor of stereochemistry inversion involved in SN2 reaction is especially crucial when synthesising drugs with chiral centres.
Consider the production of the painkiller, Paracetamol. This process entails an intermediate reaction where acid chloride (ethanoic anhydride) is treated with an amine (p-aminophenol). Here, the nitrogen in the amine, acting as a potent nucleophile, reacts with the carbonyl carbon in the acid chloride, displacing the chloride as per SN2 mechanism.Ethanoic anhydride + p-aminophenol -----> Paracetamol + Ethanoic acid
Crucially, Paracetamol not only helps relieve pain, but also reduces fever, demonstrating the impact of SN2 reaction on our day-to-day health.
Stereochemistry: The study of the spatial arrangement of atoms in molecules and their effects on the chemical behaviour of substances. In drug synthesis, the arrangement of atoms can significantly influence a drug's effectiveness and potential side effects.
Contribution of SN2 Reaction in Green Chemistry
The world of 'Green Chemistry' - a discipline aiming to reduce the environmental impact of chemical processes - capitalises extensively on the potential of SN2 reactions. One major instance lies in the production of biodiesel. During transesterification, an alcohol like methanol is introduced to a fat or oil. In the presence of a catalyst, the alcohol replaces the glycerol in the fat/oil molecule, yielding glycerine and biodiesel (alkyl esters). This process can be classed as an SN2 reaction due to the replacement of the 'leaving group' - glycerol in this case. A representation of this reaction could be:Fat/Oil + Methanol -----> Biodiesel (Alkyl esters) + GlycerineThis reaction capitalises on the abundance of fats/oils in renewable sources like plant seeds or recycled cooking oil. What's more, biodiesel produced via this process burns cleaner than fossil fuels, contributing significantly to efforts to limit global warming. SN2 reactions also play a key role in the degradation of pollutants. Certain pesticides and other harmful chemicals undergo SN2 reactions that help to mitigate their destructive impact on the environment. Hence, understanding and harnessing the power of SN2 reactions can be paramount to our pursuit of a more sustainable world.
Transesterification: A chemical reaction that replaces the alcohol in an ester compound by reacting it with another alcohol. It's an important process in the production of biodiesel.
SN1 vs SN2 Reaction Mechanism
When it comes to nucleophilic substitution reactions in organic chemistry, the two cardinal classes that confront you are the SN1 and SN2 reactions. Both mechanisms share similarities, but differences are also apparent, shaping the conditions under which they occur and the products they yield. Understanding these comparative aspects is vital for anyone hoping to gain mastery over the complexities of nucleophilic reactions.
Identifying Differences Between SN1 and SN2 Mechanisms
The differences between SN1 and SN2 reactions hinge primarily on molecularity, rate-determining steps, stereochemistry, and reaction kinetics, among other factors. To understand these, let's examine the various stages of the two mechanisms, as well as the nature of the substrates that undergo these reactions.
Molecularity: This refers to the number of molecules involved in the rate-determining step of a reaction. For SN1 reactions, the rate-determining step involves only one molecule (unimolecular) - hence SN1, whereas for SN2, two molecules interact in the rate-determining step (bimolecular) - hence SN2.
- Ionisation of the substrate, forming a carbocation and leaving group.
- Nucleophilic attack on the carbocation, forming a new bond.
Choosing Between SN1 and SN2 Reaction Mechanism: Factors to Consider
Picking between SN1 or SN2 reactions for a given substrate relies heavily on several factors. These include the type of compound, reaction conditions, the nature of the leaving group, and the use of any specific solvents.
Here's a simplified guide for determining whether a nucleophilic substitution would proceed via SN1 or SN2:- Type of Compound: SN1 reactions commonly occur in tertiary alkyl halides, where the carbocation can be stabilised by neighbouring carbon atoms. Secondary alkyl halides can undergo both SN1 and SN2, while primary alkyl halides almost exclusively follow the SN2 mechanism due to their inability to form a stable carbocation.
- Reaction Conditions: The high activation energy in SN1 reactions requires mild heating. SN2 reactions are typically carried out at room temperature.
- Leaving Group: Good leaving groups - those which can handle a negative charge well - are preferred for both SN1 and SN2. Halides and sulfonate ions are commonly employed.
- Solvent: Polar protic solvents (those capable of forming hydrogen bonds) favour SN1 because they can interact strongly with the leaving group and the carbocation. SN2 reactions usually prefer polar aprotic solvents (those incapable of forming hydrogen bonds) to improve the nucleophilicity of the incoming nucleus.
Factors Influencing SN2 Reactions
When discussing SN2 reactions, numerous factors come into play that can significantly affect the rate of reaction and the overall outcome. These include, but are not limited to, the nature of the solvent, the nucleophilicity of the reactant, the substrate structure, and the nature of the leaving group. A comprehension of these elements is pivotal for any venture into organic chemistry, especially in the applied sectors such as the pharmaceutical and environmental industries.
Impact of Solvent on SN2 Reactions
Solvents play an essential role in the realm of SN2 reactions, impacting not just the progress of the reaction, but also influencing the stereochemical aspects of the end product. The significance of solvent selection can be attributed to its direct relationship with the nucleophilicity of the reactants, thereby affecting the overall rate of the reaction.
When considering solvents, there are two broad types: polar protic and polar aprotic. The distinction comes down to their ability to form hydrogen bonds.Polar Protic Solvents: These solvents have atoms with hydrogen atoms attached directly to highly electronegative elements like \(O\) or \(N\) and can form hydrogen bonds. Examples include water (\(H_{2}O\)), methanol (\(CH_{3}OH\)), and acetic acid (\(CH_{3}COOH\)).
Polar Aprotic Solvents: These solvents have polar bonds but lack an H atom on the highly electronegative element, so they cannot form hydrogen bonds. This list includes dimethyl sulfoxide (\(C_{2}H_{6}OS\)), acetone (\(C_{3}H_{6}O\)), and ethyl acetate (\(C_{4}H_{8}O_{2}\)).
Instructions to Control the Rate of SN2 Reactions
Controlling the rate of SN2 reactions can be complex due to the various nuanced factors involved. However, with knowledge of the impacts of different factors, it becomes feasible to control these reactions with accuracy. Below are significant factors that can be manipulated to control the rate of SN2 reactions:
- Substrate: Considering the steric hindrance in the substrate is crucial. For instance, 1° alkyl halides are likely to react faster than 2° alkyl halides due to less steric hindrance.
- Nucleophile: The strength of a nucleophile impacts the reaction rate. Target the selection of nucleophiles based on factors like charge, electronegativity, size, and solvent.
- Leaving Group: Better leaving groups aid in speeding up the reaction. One should rely on leaving groups that can disperse negative charge well.
- Solvent: As already discussed, the usage of polar aprotic solvents tends to expedite SN2 type reactions.
Factor | Instruction to Increase Reaction Rate |
Substrate | Choose a substrate with less steric hindrance (e.g., 1º alkyl halide over 2º) |
Nucleophile | Opt for a stronger nucleophile (charge, electronegativity, solvent-dependency in mind) |
Leaving group | Select a good leaving group that handles negative charge well |
Solvent | Utilise polar aprotic solvents where possible |
SN2 Reaction - Key takeaways
- The SN2 Reaction Mechanism involves a nucleophile forming a bond with the substrate while the bond with the leaving group starts to break. In the transition state, the substrate is connected to five things.
- Illustrative examples of SN2 Reactions include: the formation of soaps via saponification, the conversion of glucose-1-phosphate to glucose-6-phosphate in our bodies, and the industrial production of anisole via the methylation of phenol.
- The SN2 Reaction Applications exist in areas such as: pharmaceutical industry (synthesis of medicines like Paracetamol), and 'Green Chemistry' (production of biodiesel through transesterification and degradation of pollutants).
- Comparing sn1 vs sn2 reaction mechanism: SN1 reactions proceed in two stages and via a carbocation intermediate, whereas, SN2 reactions proceed in a single concerted step without any intermediate. Some factors influencing the choice between SN1 and SN2 include the type of compound, reaction conditions, nature of the leaving group, and the type of solvent used.
- Key factors affecting SN2 Reactions include the choice of solvent, nucleophilicity of the reactant, substrate structure, and the nature of the leaving group. SN2 Reactions typically prefer polar aprotic solvents, which are incapable of forming hydrogen bonds.
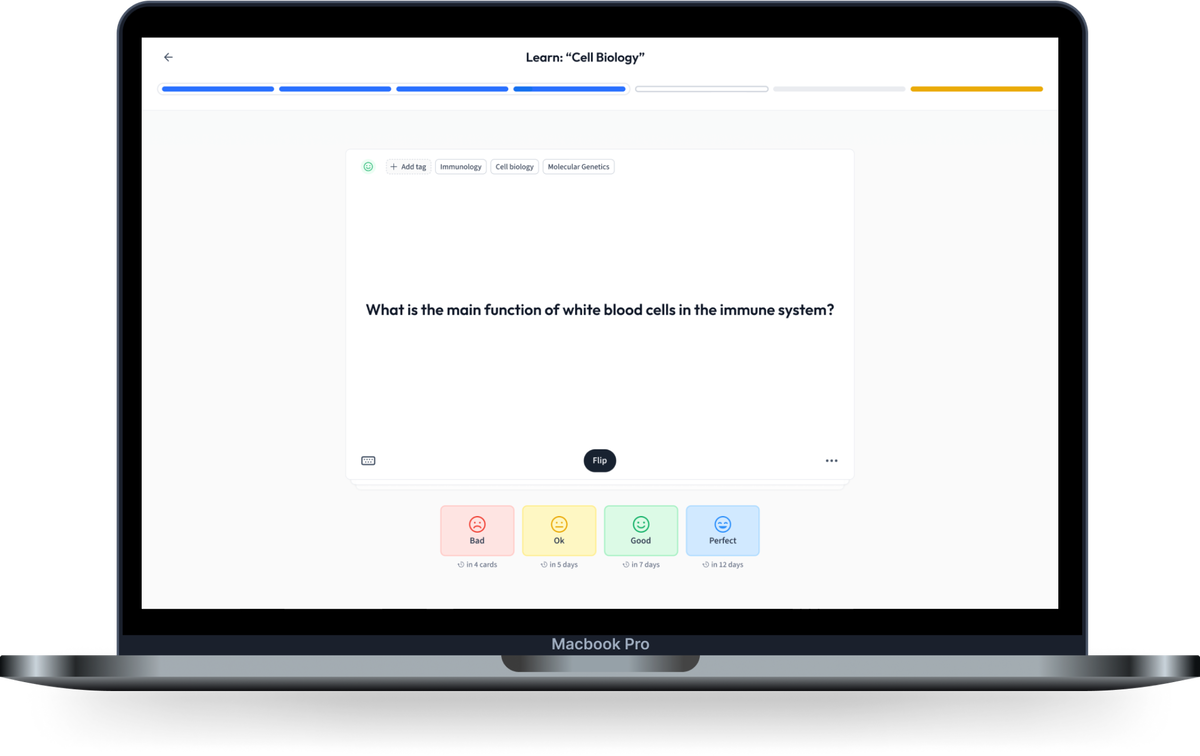
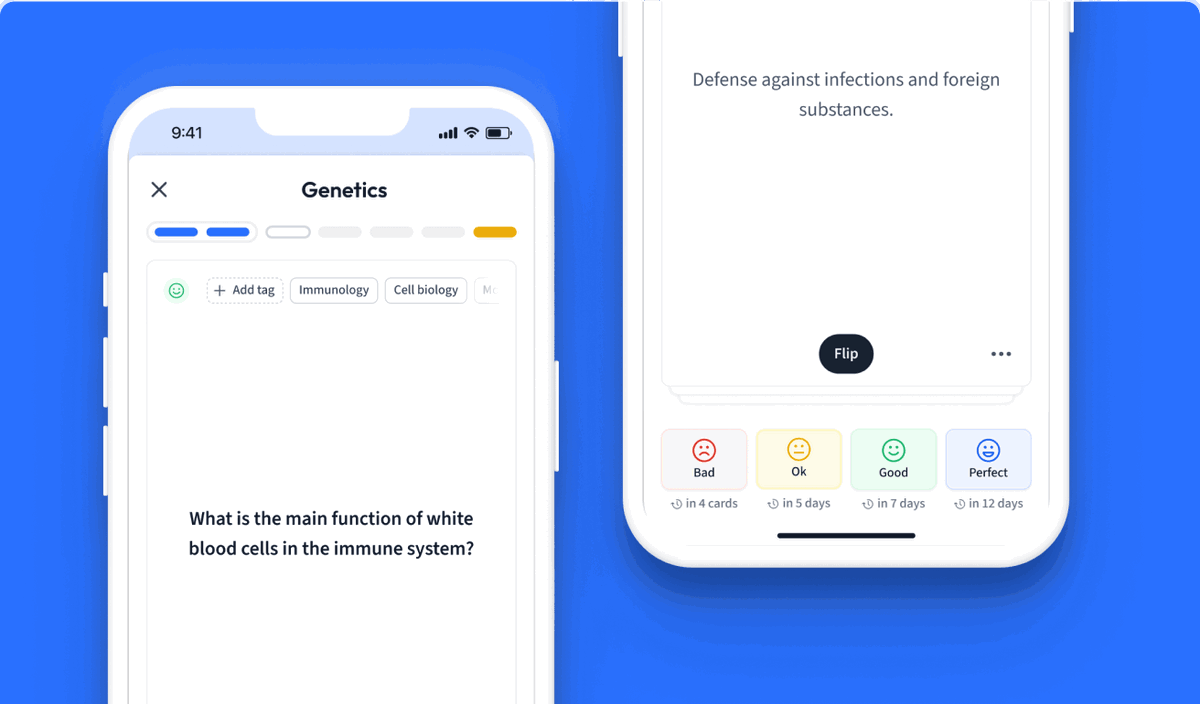
Learn with 15 SN2 Reaction flashcards in the free StudySmarter app
Already have an account? Log in
Frequently Asked Questions about SN2 Reaction
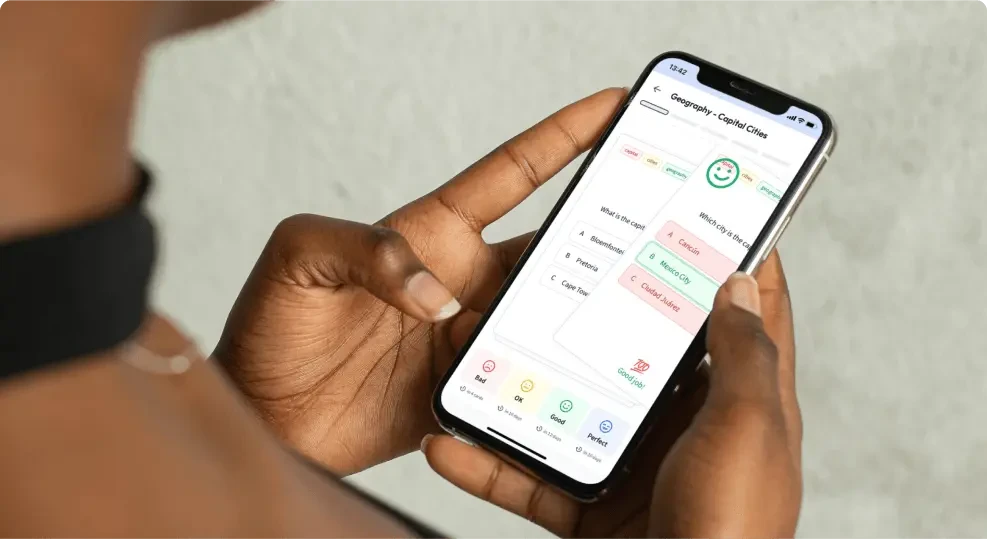
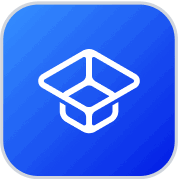
About StudySmarter
StudySmarter is a globally recognized educational technology company, offering a holistic learning platform designed for students of all ages and educational levels. Our platform provides learning support for a wide range of subjects, including STEM, Social Sciences, and Languages and also helps students to successfully master various tests and exams worldwide, such as GCSE, A Level, SAT, ACT, Abitur, and more. We offer an extensive library of learning materials, including interactive flashcards, comprehensive textbook solutions, and detailed explanations. The cutting-edge technology and tools we provide help students create their own learning materials. StudySmarter’s content is not only expert-verified but also regularly updated to ensure accuracy and relevance.
Learn more