Jump to a key chapter
What is Synthon? - Define Synthon
Have you ever heard of the term 'synthon'? It's a concept that can revolutionise your understanding of organic chemistry! In simple terms, a synthon can be described as an idealised form of a molecule's fragment. But there's more to it, so let's delve into the basics of synthons and why they matter in organic chemistry.
The Basics of Synthons
At its core, a synthon isn't something you can buy from a chemical supplier. Instead, it’s a conceptual tool used by chemists to help visualise the breaking and forming of bonds in the process of a chemical reaction.
"Synthon" is derived from the Greek words "syn", meaning 'together' and "onthos", meaning 'being'. It was coined by the Nobel laureate E.J. Corey and stands for 'synthetic units' that are used for the transformations in retrosynthesis.
Synthons help to simplify complex organic structures and easily understand the reaction mechanisms. They are particularly important in retrosynthesis, which is the process of breaking down complex molecules into simpler ones for easier synthesis.
For example, in the reaction of an acid chloride to a ketone, the acid chloride can be considered as two synthons - an acyl cation (\( \text{R-CO}^+ \)) and a chloride anion (\( \text{Cl}^- \)).
Understanding Synthons through their unique characteristics
A synthon showcases several unique characteristics which are crucial in understanding its behaviour in a chemical reaction.
They are highly reactive species, more reactive than the actual reagents normally used in synthesis.
Synthons are usually represented as ions, cations for electrophilic centres and anions for nucleophilic centres.
They help in the planning of a synthetic route in retrosynthetic analysis.
They are drawn as a fragment of the target molecule where modifications are required to reach the desired compound.
Defining Synthon: Crucial in Organic Chemistry
Understanding the concept of synthons can be a game-changer in studying organic chemistry, especially for those planning to delve deeper into the exciting world of molecular synthesis.
Property | Explanation/Example |
Synthetic Value | Synthons represent the methodology in retrosynthetic analysis, transforming complex molecules into simple ones. |
Reactivity | Being highly reactive species, synthons assist in the reaction process. |
Structure | Synthons showcase how the molecular structure can be broken down into fragments, simplifying the route of synthesis. |
Interestingly, while synthons are not actual entities, they are closely related to synthetic equivalents, which are real compounds having the same reactivity as the synthon. Synthetic equivalents are crucial as they bridge the gap between theory and reality in organic synthesis.
Now, consider for a moment how vital synthons are in the world of organic chemistry. These theoretical constructs play an essential role in retrosynthetic analysis and understand various reaction mechanisms. They may not exist in the physical world, but they are definitely real in the world of chemistry!
Retrosynthesis Synthons in Organic Chemistry
Delving deeper into synthetic chemistry, let's focus our attention on how retrosynthesis and synthons play a crucial role in organic chemistry. These two concepts are interconnected and provide chemists a structured approach for designing synthetic pathways for complex chemical compounds.
Importance of Retrosynthesis and Synthons
Retrosynthesis, coupled with the concept of synthons, forms a cornerstone of organic chemistry. Breaking down a complicated chemical structure into simpler, synthesizable molecules significantly simplifies the process of organic synthesis. Retrosynthesis is a technique by which a chemist plans the synthesis of complex organic molecules by decomposing a target molecule (TM) into simpler starting materials.
- Understanding the relationship between the target molecule and its smaller, cheaper, and readily accessible fragments is at the heart of retrosynthesis. These fragments are represented as synthons.
- Conceptually synthesizing a molecule in reverse means thinking backwards from the TM in order to discover the best synthetic pathway. Synthons play a crucial role in retrosynthetic analysis by providing a logical method to dissect complex molecules.
- Retrosynthetic planning and the idea of synthons go hand in hand. Much like a jigsaw puzzle, synthons can be rearranged to form the target molecule. The transformation of these synthons into the target molecule creates an effective road map for synthesis.
Practical Examples: Synthon technique in Chemistry
To further demonstrate the practical application of synthons and retrosynthesis, let's go through a few examples:
Let's consider the synthesis of an important compound in organic chemistry: phenol. Phenol can be broken down into two synthons: a negatively charged phenoxide (-O^-) and a positively charged hydrogen ion (H^+). This can be represented as \[ \text{Phenol} \rightarrow \text{Phenoxide} (O^-) + \text{Proton} (H^+) \]. The synthetic equivalents can be sodium phenoxide and water (because water can provide the proton).
Another example could be the synthesis of an alcohol. Here, an alcohol can be broken down into a carbonyl compound (\(R-CHO\)) and \(H_2\): \[ \text{Alcohol} \rightarrow \text{Carbonyl} (RCHO) + \text{hydrogen} (H2) \]. The synthetic equivalent here could be a primary alcohol and a reducing agent.
Retrosynthesis Synthons: The Backbone of Organic Chemistry
Throughout the exploration of the concepts of retrosynthesis and synthons, one thing remains consistent - their high importance within the organic chemistry universe. With the ability to simplify complexities, synthons and retrosynthesis prove to be the backbone or support structure of organic chemistry for a number of reasons:
Property | Explanation |
Predictive Capacity | Synthons can predict the desired changes in a molecular structure to reach a target molecule. This guides the planning of a synthetic route in retrosynthetic analysis. |
Breakdown of Complex Molecules | Through retrosynthesis, complex molecules can be broken down into simpler ones, thereby identifying the synthetic equivalents. This simplifies the process of organic synthesis and the understanding of various reaction mechanisms. |
Offers Strategic Approach to Synthesis | Retrosynthesis offers a strategic approach in synthesizing complex organic molecules, making it more efficient and controllable. |
That said, retrosynthesis and synthons cannot be overlooked when studying organic chemistry. They offer to simplify the process of understanding and planning complex synthetic transformations, turning the intimidating into a fascinating exploration of molecules and their transformations.
Examples of Synthon in Chemistry
For more in-depth understanding of synthons in chemistry, examples serve as effective tools. By examining more closely how synthons work in real-life organic chemistry scenarios, it becomes easier to gain a comprehensive view of the synthesis processes and also understand the extent of their application in the field of chemistry. The following sections highlight some notable examples of synthons in chemistry and showcase how they are utilised in practical scenarios.
Popular Examples of Synthon
The use of synthons is particularly evident in the planning stage of organic synthesis. Understanding synthons can help chemists visualise the various ways a molecule can be formed, aiding in the creation of a successful pathway for its synthesis. Below are a few illustrations of synthons.
Consider the compound ethanol. In a retrosynthetic analysis, ethanol can be considered as composed of a negatively charged ethoxide ion (\( CH_3CH_2O^- \)) and a positively charged proton (\( H^+ \)). From an organic chemistry perspective, these would be your synthons. To find the synthetic equivalents, we would look for actual chemical species that can behave similarly in a synthetic reaction. In this case, ethoxide could be represented by sodium ethoxide (\( CH_3CH_2ONa \)), and proton can be provided by an acid like \( HCl \).
Another example would be in the retrosynthesis of the molecule 2-butanol. The target molecule can be divided into a secondary alkyl carbanion synthon (\( CH_3CH^-CH_2CH_3 \)) and a proton synthon (\( H^+ \)). Synthetic equivalents for these synthons could be 2-bromobutane and water or any other base, which would create 2-butanol when combined.
These examples might seem simple, but they lay the foundation behind more complex synthetic reactions. Synthons guide the strategy towards the synthesis of very complex structures, simplifying the approach and making it more efficient.
How are Synthons used in Organic Chemistry?
Synthons are primarily used in organic chemistry to design synthetic routes towards the formation of complex organic molecules. By understanding the functional groups of the target molecule and how they react, chemists can identify possible bond disconnections, thus revealing potential synthons.
Here's a structured approach on how synthons are used in organic chemistry:
- Identify the target molecule and its functional groups
- Consider possible disconnections that will break the molecule into simpler structures
- Conceptualize the resulting fragments as synthons
- Determine the real chemical entities or the synthetic equivalents for these synthons
- Consider potential conditions under which these synthons or synthetic equivalents can be combined to form the target molecule
Real-life cases: Synthon Applications
In real-life chemical scenarios, the application of synthons goes much beyond the classroom. They are an integral part of the development and discovery of new medicines, the synthesis of complex natural products, and the design of new materials.
Here's a brief look at the real-life applications of synthons:
- Synthesis of Medicines: Many pharmaceutical drugs are produced by complex organic synthesis processes which extensively use the concept of synthons.
- Natural Product Synthesis: Natural products often have complex structures that present many challenges for chemists. Synthons are extremely useful in these complex syntheses. For instance, the synthesis of several complex antibiotics relies heavily on the use of synthons.
- Development of New Materials: The production processes of many modern materials, for example, light-emitting diodes (LEDs), polymers, and advanced functional materials that are used in the manufacture of electronic devices, utilise the concept of synthons in their synthesis.
The practical applications of synthons are wide-ranging and crucial in diverse areas of scientific research and industry. Understanding the concept of synthons provides valuable insights into the intricate processes of organic synthesis, paving the way towards the exciting world of molecular engineering.
Synthon Technique in Chemistry: A Comprehensive Review
Within the tapestry of organic chemistry, the synthon technique plays a complex and vital role, contributing greatly to the development of new molecular constructions. This technique, as a cornerstone in retrosynthesis planning, largely influences how chemists design strategies to create complex organic molecules from simpler starting materials. Understanding the synthon technique is crucial for chemists looking to devise efficient, streamlined approaches to molecular synthesis.
Mechanisms involved in Synthon Technique
Delving deeper into the workings of the synthon technique requires a strong grasp of its foundational mechanisms. At its core, the synthon technique is all about rearranging chemical structures, dissecting an intricate molecule into relatively simpler objects known as synthons. These synthons outline the route for the synthesis of the complex molecule of interest. The most fundamental mechanisms of the synthon technique revolve around two key components: retrosynthetic analysis and disconnections.
The retrosynthetic analysis is a planning mechanism that involves a thought experiment. It invites chemists to consider the synthesis of a complex organic molecule from the finish line to the starting point. As a methodical process for devising the optimum synthetic plan for an organic compound, it involves the identification of the bond (or bonds) in the target molecule that can be dissected to yield simpler, readily accessible fragments—an exercise leading to possible synthons.
The retrosynthetic process is largely influenced by the rules of reactivity, chemoselectivity, and stereoselectivity—parameters that assure the feasibility and success of the transformation. The chemist essentially works backward from the TM to simple, commercially available starting materials through sequential disconnections.
Disconnections play a crucial role in retrosynthetic analysis. This theoretical concept involves envisaging the breaking of a bond within the target molecule, leading to the formation of two (or more) synthons. Bond disconnections are guided by two important principles: functional group interconversions and use of synthetic equivalents. These principles aid chemists in departing from the retrosynthetic world (synthons) into the synthetic world (real molecules).
How does the Synthon technique work?
A closer exploration of the synthon technique offers a more practical understanding of its operation. The synthon technique functions through a sequence of ordered disconnections. Each disconnection is a possible retrosynthetic step. While working with the synthon technique, chemists begin by defining the target structure or target molecule. The process follows these steps:
- Identify the bond (or bonds) in the target molecule that can be broken into simple parts.
- Visualise and label synthons resulting from these breaks, highlighting the charges and possible reactive partners for each synthon.
- Find synthetic equivalents or 'reagents', actual chemical species that mirror the synthons' behaviour.
- Planning the chemical routes that can assemble these reagents to create the target molecule.
It must be noted that the negative charge on a synthon indicates the need for a synthetic equivalent that can act as a nucleophile. Similarly, a positive charge on the synthon indicates a requirement for a synthetic equivalent that can act as an electrophile.
Exploring the Impact of Synthon Technique in Chemistry
The impact of the synthon technique in the field of chemistry is profound. It has revolutionised the approach to synthetic organic chemistry, pushing the limits of chemical innovation. The synthon technique offers several advantageous outcomes:
- Strategic Synthesis Planning: Using synthons simplify the envisioning of complex molecule assembly. This adds a strategic edge to synthesis planning, making it more coordinated, efficient and effective.
- Overcoming Synthetic Challenges: Synthons help to address synthetic challenges by offering systematic and logical pathways for molecular assembly.
- Enhanced Understanding of Reactions: Synthons also play a significant role in understanding reaction mechanisms by visually representing how parts of a molecule react.
The synthon technique has left a significant footprint on various sub-domains of chemistry. In medicinal chemistry, it is used to drive the synthesis of new pharmaceuticals. Similarly, in material science, the synthon approach simplifies the building of complex materials with defined properties.
Overall, the benefits and influence of the synthon technique extend far beyond its academic importance. It is a crucial aspect that enables chemists to design, formulate, and synthesise complex molecules with high efficiency and selectivity, thereby playing a vital part in advancing the field of chemistry.
Meaning of Synthon in Chemistry: Exploring its Significance
In the fascinating realm of organic chemistry, the concept of a 'synthon' holds immense significance. Coined by the widely renowned chemist E.J. Corey, the term synthon refers to a structural unit within a molecule that is capable of forming a bond with other molecules or molecular fragments during a synthetic process. Essentially, synthons provide a powerful thinking tool that allows chemists to deconstruct complex molecules into simpler, manageable components in retrosynthetic analysis, a part of the synthetic planning process. Synthons, however, are theoretical and don't necessarily exist per se; they represent how chemists envisage the breakdown of a complex molecule into simpler parts. It is their synthetic equivalents—real chemical species—that are used in laboratory processes.
Why is the Concept of Synthon Important?
Understanding the concept of synthons is of vital importance in contemporary synthetic chemistry for several reasons. They provide a theoretical framework that chemists use to design synthetic pathways for complex organic molecules—a critical step in the advancement of medicine, material science, and many other scientific fields. Essentially, synthons are the linchpins that hold the strategic framework of retrosynthetic analysis together.
The primary function of a synthon is to simplify the conceptual process of bond disconnection during retrosynthetic analysis. When a chemist looks at a complex molecule, they can mentally dissect the molecule into smaller synthons, thereby revealing potential routes for the synthesis of the said molecule. This disconnection into synthons helps streamline the synthesis process, bringing clarity to the synthetic strategy. To illustrate this, a ketone molecule, when broken down into its synthons, can be represented as \(R_1CO^-+R_2H^+\).
Once synthons are identified, chemists can then look for real, existing chemicals, or 'synthetic equivalents', which exhibit similar reactivity. Such equivalents can be utilised in actual laboratory processes to synthesise the complex molecule. Therefore, the concept of synthons essentially links theoretical disconnections to practical synthetic methods.
<_>Deciphering Synthon: A closer look
Delving deeper into the anatomy of a synthon, one can observe that synthons often bear charges since they arise from theoretical bond disconnections of a neutral molecule. In reality, when a chemist performs a disconnection, it's not a physical disconnection; instead, it reflects the strategic disconnection of a bond using electron-pushing diagrams.
Here's an in-depth breakdown of the structure of a synthon:
- Nucleophilic Synthons: A synthon with a negative charge implies that a nucleophile is necessary in the synthetic process. For example, in a retrosynthetic analysis of an alcohol molecule (R-OH), when the hydroxyl group is disconnected, it generates a nucleophilic oxygen synthon (\(R-O^-\)) and a hydrogen cation (\(H^+\)).
- Electrophilic Synthons: A synthon with a positive charge indicates the need for an electrophile. For example, a carbonyl molecule (R-CO-R') can be disconnected to generate a carbocation synthon (\(R^+ \)) and a carbanion synthon (\(R'^-\)).
While conceptualising synthons, one must remember that synthons don't really exist; they are imaginary constructs that are useful for planning a synthetic route. The real entities utilised in synthesis are their corresponding synthetic equivalents, derived from appropriate starting materials.
The Implication of Synthon in Contemporary Chemistry
In today's scientific landscape, the concept of synthons has vital implications. It fosters an innovative thought process in organic synthesis that dramatically enhances the scope of synthetic chemistry. It allows chemists to conquer the complexity of complicated molecular structures and has made it possible to synthesise a virtually infinite variety of organic structures, thus opening up new horizons in the field of chemistry.
Here are some notable applications of synthons in contemporary chemistry:
- Medicine Synthesis: The synthesis of most drugs involves complex organic chemistry. Understanding synthons allows pharmaceutical chemists to strategise and develop new, effective drug molecules.
- Natural Product Synthesis: Nature produces myriad complex organic molecules, and scientists often need to recreate these in the lab. The concept of synthons is a powerful tool in their toolbox, helping to design ways to create these complex structures.
- Material Development: Synthons are also instrumental in the development of new materials. By understanding how synthons work, chemists are able to devise ways of constructing complicated materials, such as polymers and electronic materials. `
From a broader perspective, synthons represent a paradigm shift in how chemists think about molecular creation. They have ushered in a new era of strategic, thoughtful, and logical synthetic organic chemistry that reaches far beyond the lab, into pharmaceutical factories, material science labs, and many other fields.
Synthons - Key takeaways
- Synthons are theoretical structural units within a molecule that are capable of forming a bond with other molecules or molecular fragments during a synthetic process.
- Retrosynthesis is a technique in organic chemistry that involves deconstructing a target molecule into simpler, synthesizable molecules. This helps in planning the synthesis of complex organic molecules.
- Synthons are key components in the retrosynthesis process. Synthons can predict the desired changes in a molecular structure for achieving a target molecule and guide the planning of a synthetic route in retrosynthetic analysis.
- Synthons are used in practical applications such as the synthesis of medicines, natural product synthesis, and the development of new materials. Understanding synthons can provide valuable insights into organic synthesis and molecular engineering.
- The synthon technique is about rearranging chemical structures, dissecting a complex molecule into simpler objects known as synthons. This approach plays a complex and vital role in organic chemistry, influencing how chemists devise strategies for creating complex organic molecules from simpler starting materials.
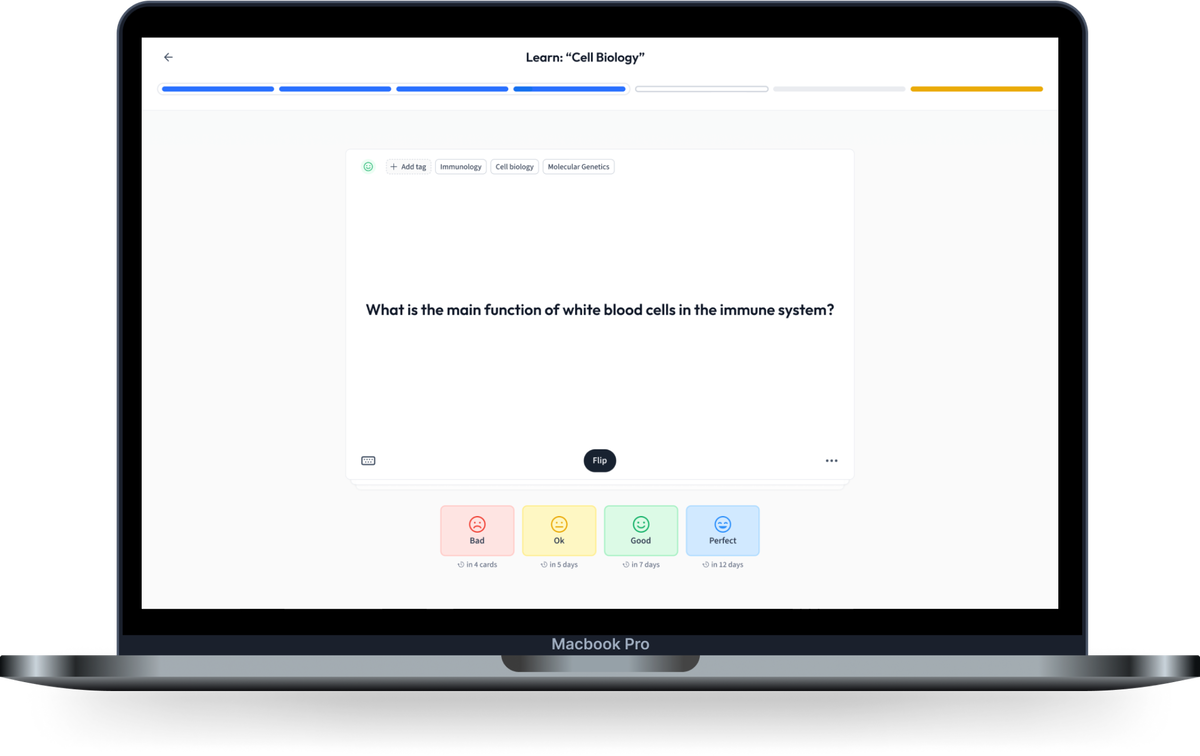
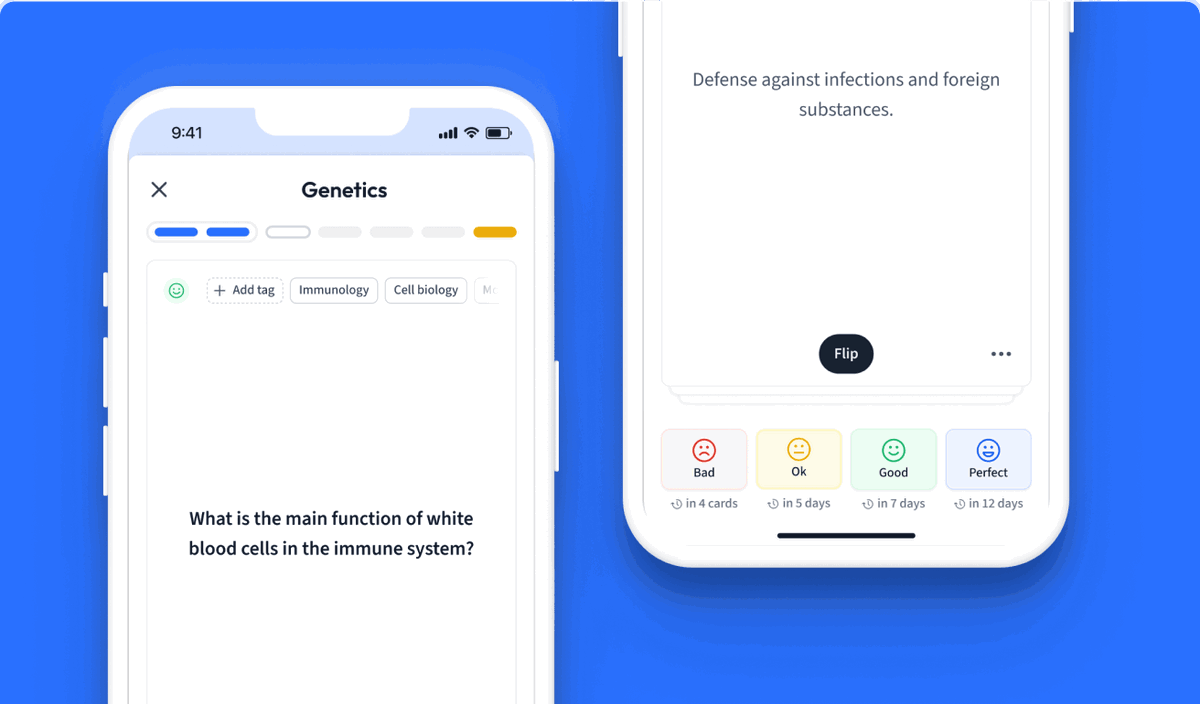
Learn with 15 Synthons flashcards in the free StudySmarter app
Already have an account? Log in
Frequently Asked Questions about Synthons
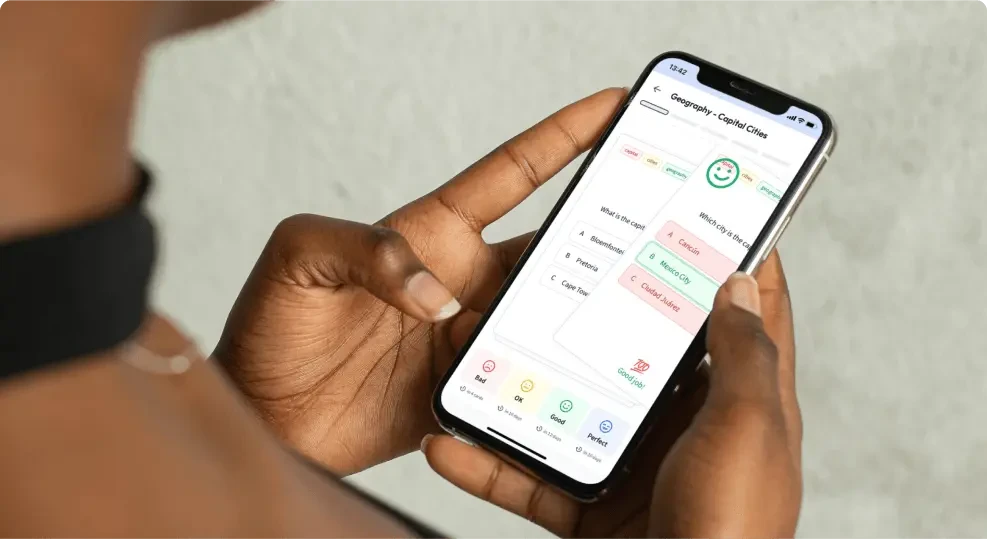
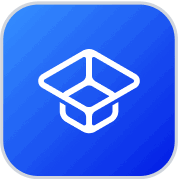
About StudySmarter
StudySmarter is a globally recognized educational technology company, offering a holistic learning platform designed for students of all ages and educational levels. Our platform provides learning support for a wide range of subjects, including STEM, Social Sciences, and Languages and also helps students to successfully master various tests and exams worldwide, such as GCSE, A Level, SAT, ACT, Abitur, and more. We offer an extensive library of learning materials, including interactive flashcards, comprehensive textbook solutions, and detailed explanations. The cutting-edge technology and tools we provide help students create their own learning materials. StudySmarter’s content is not only expert-verified but also regularly updated to ensure accuracy and relevance.
Learn more