Jump to a key chapter
Understanding Zwitterion and Its Features
Firstly, let's dive into the world of chemistry. You'll find that one of the most unique things about this science is the diversity it reveals in the molecular world. The term 'zwitterion' is one such example. It refers to a molecule that carries both positive and negative charges.A zwitterion, also known as an internally compensated ion, possesses both positive and negative charges. However, despite having two different charges, the net charge of the molecule remains zero.
The Concept of Zwitterion
The word 'zwitterion' originates from the German language where 'zwitter' means 'hybrid'. This accurately describes the nature of zwitterions as they exhibit both positive and negative charges in the same molecule.
Zwitterion Characteristics
Zwitterions have several unique characteristics which set them apart from other compounds. Here are some of them:- Their ability to simultaneously carry both positive and negative charges.
- The presence of stable internal salts.
- Their behaviour in polar solvents such as water.
- Their high melting points due to the strong ionic bonds they form.
- Their ability to act as buffers in certain solutions.
Zwitterion Example in Everyday Life
To illustrate the importance of zwitterions in everyday life, let's consider the case of amino acids. These small molecules serve as the building blocks for proteins, which are essential for nearly every biological process.Take glycine, the simplest amino acid. At neutral pH, it exists as a zwitterion with a deprotonated carboxyl group and a protonated amino group, as shown in the equation below.
The Relationship Between Zwitterion and Amino Acid
Zwitterions and amino acids share a profound relationship. To be precise, the zwitterionic form of amino acids gives these molecules their unique properties and behaviours. It greatly influences their role in biological systems, especially protein synthesis.Amino Acid Zwitterion: What It Means
To understand the concept of an amino acid zwitterion, envision an amino acid's structure. It typically includes a central carbon atom (the alpha carbon) connected to four different groups - a hydrogen atom, an amino group, a carboxyl group, and a side chain. The last one varies to give each amino acid its uniqueness, totalling 20 with distinct characteristics. Now, consider this amino acid in a neutral pH environment - something magical happens. The carboxyl group, initially a weak acid, donates a proton (H+) and becomes negatively charged. Simultaneously, the basic amino group accepts a proton and becomes positively charged. Consequently, every amino acid has a characteristic zwitterionic form in which it exists as a particle with a net charge of zero. This behavior is illustrated by the following equation using general terms for carboxyl and amino groups. \[ \text{{-COOH}} + \text{{-NH2}} \rightarrow \text{{-COO-}} + \text{{-NH3+}} \] Remember that the zwitterionic state is highly dependent on pH. The molecule would lose or gain protons based on the environmental acidity or alkalinity, thus shifting from its zwitterionic status.Alanine Zwitterion: A Closer Look
Alanine, a non-polar, hydrophobic amino acid, serves as a good illustration of this pH-dependent behavior. Its structure involves a methyl group as the side chain. At a very low pH (highly acidic environment), both the carboxyl and amino groups become protonated, rendering the molecule a positive charge. On the other hand, at a very high pH (highly alkaline environment), both groups lose their protons, and the alanine molecule carries a negative net charge. However, at a neutral pH around 6.02 (alanine's isoelectric point), alanine exists as a zwitterion. The following equation shows the zwitterionic form of alanine. \[ \text{{-NH3+CH3CHCOO-}} \] This zwitterionic behaviour is essential in enabling the formation of peptides - a crucial step towards building proteins.Aspartic Acid Zwitterion: Delving Deeper
Aspartic acid, a polar and negatively charged amino acid because of its acidic side chain, also demonstrates zwitterionic behaviour. This polar amino acid has a carboxyl side chain. As a result, at a specific pH (its isoelectric point, approximately 2.77), aspartic acid exists in a zwitterionic form, with both its carboxyl groups deprotonated and its amino group protonated. The formula below displays the zwitterion state of aspartic acid. \[ \text{{-NH3+CHCH2COO-COO-}} \] Bear in mind that in an environment with extreme pH conditions, the aspartic acid will alter its state as per the acidity or alkalinity. Laying the foundation with these examples, the concept of zwitterions cements your understanding of the properties and behaviour of fundamental biological building blocks - amino acids. Precisely, it underlines how each molecule can engage with its environment, affecting interactions and reactions crucial to life.Why are Amino Acids Zwitterions?
A fascinating aspect of amino acids, the building blocks of proteins, is that they exist as zwitterions. This peculiar situation arises from the presence of two functional groups in amino acids: the carboxyl and amino groups.The Biochemistry behind Amino Acids as Zwitterions
To comprehend why amino acids exist as zwitterions, take into account the nature of the carboxyl (\(-COOH\)) and amino (\(-NH2\)) groups. Both of these entities are weak acids and bases, respectively. Particularly under specific environment conditions (pH), they can exchange protons, a phenomenon known as proton transfer. The carboxyl group, being slightly acidic, tends to lose a proton (H+), which results in a negatively charged carboxylate ion (\(-COO-\)). Conversely, the amino group, being slightly basic, tends to accept a proton, transforming into a positively charged ammonium ion (\(-NH3+\)). This simultaneous loss and gain of protons temporarily create a molecule with a net charge of zero, despite carrying both positive and negative charges. This state of the molecule is what you refer to as a zwitterion, and it primarily occurs at a region referred to as the pKa. Specifically, the pKa of a molecule is the pH at which half the molecules are deprotonated, and half remain protonated. For most amino acids, this value is typically around physiological pH, although there are variations depending on the amino acid in question and its chemical environment.Why Alanine and Aspartic Acid Exist as Zwitterions
Taking specific examples, both alanine and aspartic acid can exist as zwitterions, but this status depends indeed on pH conditions. In alanine, under very acidic conditions, the molecule will exist entirely in its cationic state, with both the carboxyl and amino groups protonated. Raise the pH, and you'll reach a point where the carboxyl group gives up a proton, and alanine adopts its zwitterionic state. Raise the pH further, and you'll pass the pKa of the amino group. Now, the alanine molecule exists entirely in its anionic form with both groups deprotonated. Aspartic acid has an additional carboxyl group in its side chain, which adds another layer of complexity to matters. At very low pH, all groups are protonated. Increase the pH, and first, the -COOH in the side chain loses a proton. Aspartic acid is still positively charged at this pH. Raise the pH further, and the central -COOH group gives up a proton. Now we're at the zwitterionic state. However, keep raising the pH, and eventually the -NH3+ gives up a proton, remaining anionic. In both cases, the specific conditions dictate which form the molecule adopts, reinforcing the importance of understanding this dynamic equilibrium.The Role of Zwitterions in Amino Acid Properties and Reactions
The zwitterionic state of amino acids plays various roles, particularly in biochemical reactions. Because they carry both positive and negative charges, zwitterions tend to be highly polar and thus readily soluble in water, a fundamental property for amino acids' physiological roles. Another critical behaviour of zwitterions is their isoelectric point (pI) which is the pH where the compound exists almost entirely in zwitterionic form. At this pH, the compound carries no net electric charge providing an environment that minimises solubility and promotes crystallisation. Moreover, zwitterions can act as buffers, resisting changes in pH upon addition of an acid or base. While most molecules would readily accept or donate a proton altering the pH, zwitterions can both accept and donate protons allowing the solution to maintain a relatively constant pH. Furthermore, the zwitterionic property impacts peptide bond formation, the linkage responsible for connecting amino acids in protein structures. The negatively charged carboxyl group (\(-COO-\)) of one amino acid reacts with the positively charged amino group (\(-NH3+\)) of another amino acid forming a peptide bond via dehydration synthesis, a process in which water is released. The fundamental understanding of zwitterions enables you to indeed appreciate their crucial roles in biochemistry and molecular biology, highlighting the intertwined complexity between structure and function in biological macromolecules.Exploring Other Zwitterionic Compounds
While amino acids are perhaps the most recognized zwitterions, you can find such ion forms in several other compounds. These other zwitterions underline the pervasive influence of this distinct molecular state in chemistry, with wide-ranging implications not only in biochemistry but also in organic and inorganic chemistry.Beyond Amino Acids: Other Zwitterion Examples
Let's extend your knowledge horizon beyond the realm of amino acids and delve into some other examples of zwitterions that comprise of many notable and industrially significant compounds:Glycine: Although it is recognised as the simplest and the only achiral amino acid, glycine owes its highlight here due to the ease to comprehend structural simplicity. In a neutral pH solution, glycine exists in its zwitterionic state, characterised as \(-NH3+CH2COO-\).
Sulfamic Acid: Sulfamic acid or amidosulfonic acid is a compound carrying a sulfonic group (-SO3H). However, at room temperature, the sulfonic group can lose a proton while the amino group can gain a proton. This is represented as \(NH3+SO3-\), which describes the zwitterionic state of sulfamic acid.
CHAPS: 3-[(3-Cholamidopropyl)dimethylammonio]-1-propanesulfonate, commercially known as CHAPS, is a zwitterionic detergent used in laboratories to solubilise proteins. The sulfonate group carries a negative charge while the quaternary ammonium carries a positive charge making it a zwitterion.
Buffer Compounds: Many buffering agents, e.g., PIPES and HEPES, designed to resist changes in pH are zwitterionic. They can absorb or release protons, strengthening their buffering capability, thus making them effective even in narrow pH ranges.
Betaines: Betaines, such as trimethylglycine, are another class of compounds that exist as zwitterions. Betaines are characterised by \(R3N+COO-\) functional groups. They function as osmolytes, protecting cells against dehydration, also used as surfactants in the cosmetics industry.
The Role of Zwitterions in Biochemistry and Organic Chemistry
The existence and functional relevance of zwitterions isn't limited only to the field of biochemistry. They also hold a significant role in various chemical reactions and industrial applications within organic chemistry. Let's now explore those in detail,Acid-Base Reactions: A core aspect of zwitterions' role in chemistry comes from their being the products of acid-base reactions, where the relocation of a proton from an acidic group to a basic group within the molecule creates an internal salt bearing both positive and negative charges.
Solubility and Crystallisation: The presence of both positive and negative charges on zwitterions allows them to readily interact with polar solvents like water, thus influencing their solubility. They only display minimum solubility at their isoelectric point (pi), which favours the crystallisation of these molecules.
Chemical Synthesis: Zwitterions often participate in chemical reactions, specifically cycloadditions and dipolarophile with other species. This process helps synthesise new compounds. Notably, 1,3-Dipolar cycloadditions are cornerstone reactions in the synthesis of heterocyclic compounds.
Catalysis: Some zwitterions function as potent catalysts in organic reactions. For instance, they can catalyse the stereospecific addition of nitromethane to unsaturated carbonyl compounds. Betaines, a type of zwitterions, have also found use in automotive coolant formulations due to their high thermal stability and as effective corrosion inhibitors.
Unlocking the Mystery of Zwitterion
In the vast world of chemistry, a fascinating array of molecules, each with unique characteristics, is present. Among these, zwitterions stand out due to their intriguing dual-charged nature. As the term suggests, from the German 'Zwiter', meaning 'hybrid', zwitterions are essentially hybrid ions. They contain both negatively charged and positively charged functional groups, with the overall molecular charge being zero.The Meaning of Zwitterion in Organic Chemistry
Zwitterions occur when a molecule with both a basic and an acidic group transfers a proton within the molecule from the acid to the base. The most common representative examples of zwitterions are amino acids, which you often think of as the primary building blocks of proteins.In amino acids, a molecule has a basic amino (\(-NH2\)) group and an acidic carboxyl (\(-COOH\)) group. Under appropriate conditions (usually neutral pH), a proton transfer occurs from the carboxyl group to the amino group, resulting in the amino group gaining a positive charge (\(-NH3+\)) and the carboxyl group carrying a negative charge (\(-COO-\)).
For example, the zwitterionic form of the simplest amino acid, glycine, is represented as \(-NH3+CH2COO-\).
However, zwitterions are not limited to amino acids. Other examples include certain biological buffers like HEPES and PIPES, and you can find these in sulfonic acids and betaines as well. Other even more complex compounds can exist as zwitterions, such as sulfamic acid, with its structure represented as \(NH3+SO3-\).
Betaines are another class of zwitterionic compounds generally defined by the presence of \(R3N+COO-\) functional groups. Betaines also include a group of substances in living organisms that protect cells against dehydration.Sometimes, molecules may not have designated acidic and basic groups but can still form structures akin to zwitterions.
How the Knowledge of Zwitterions Contributes to the Study of Biochemistry and Organic Chemistry
Understanding the concept of zwitterions is fundamental to various aspects of biochemistry and organic chemistry. The presence of zwitterionic amino acids plays a pivotal role in protein structures and bio-functions. The dual charge nature of zwitterions also permits them to behave as dipolar species, which allows warm-blooded species to resist changes in bodily pH (acid-base balance), ensuring that processes in the body occur under stable pH conditions. Zwitterions also form the bedrock of several biochemical reactions. For instance, their role in peptide bond formation is essential in the construction of protein chains. The negatively charged carboxyl group (\(-COO-\)) of one amino acid can react with the positively charged amino group (\(-NH3+\)) of another amino acid, facilitating the formation of a peptide bond, the linkage essential to connect amino acids into protein structures. Zwitterions also possess significant influence in organic chemistry. These compounds are critical in the orchestrating stereospecific organic reactions and can serve as catalysts in many chemical synthesis processes. They can participate uniquely in 1,3-dipolar cycloaddition reactions, which are valuable tools for synthesising five-membered heterocycles. The study of zwitterions advances the understanding of various chemical reactions, provides insights into the factors influencing molecule solubility, and guides the synthesis of new compounds. Zwitterions prove to be salient components in multiple commercially valuable applications, including detergents, fuel cells, and ion exchange resins. Therefore, the study of zwitterions and the knowledge of their properties and behaviours provide a valuable perspective that interlinks numerous realms within organic and biochemistry, driving the progress in both applied and academic ventures.Zwitterion - Key takeaways
- Zwitterions refer to hybrid ions, having both negative and positive charges, yet with an overall net charge of zero.
- In a neutral pH environment, amino acids exist as zwitterions, where the carboxyl group donates a proton (H+) and becomes negatively charged, while the amino group accepts a proton and becomes positively charged.
- Alanine and aspartic acid can exhibit zwitterionic behaviour. However, this behaviour depends on pH levels, with neutral pH fostering the zwitterionic state. In extreme pH conditions, these amino acids shift from their zwitterionic form.
- Zwitterions play a crucial role in biochemical reactions. They are soluble in water due to their polarity, can act as buffers resisting changes in pH and facilitate peptide bond formation in protein structures.
- Besides amino acids, zwitterions are found in other compounds, including glycine, sulfamic acid, CHAPS, buffer compounds, and betaines. This zwitterionic state plays a key role in acid-base reactions, solubility and crystallisation, chemical synthesis, catalysis, and the formulation of pH-responsive materials.
Learn faster with the 15 flashcards about Zwitterion
Sign up for free to gain access to all our flashcards.
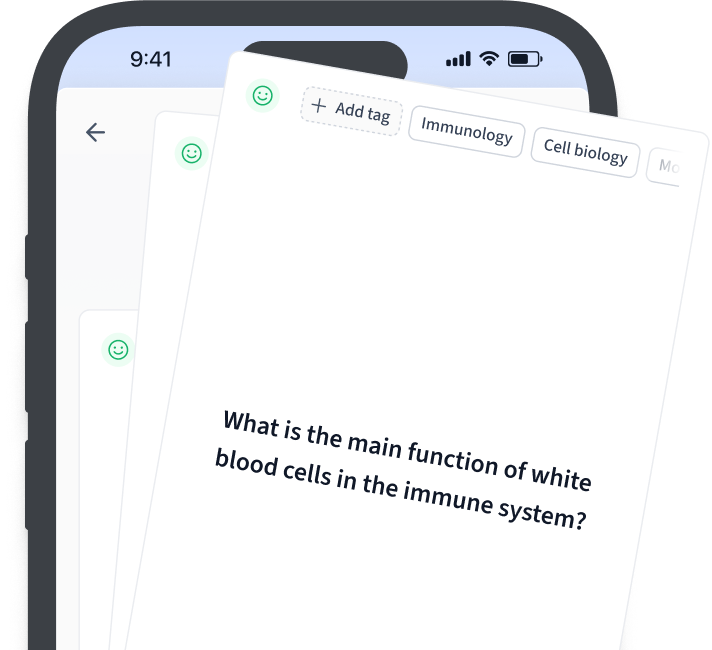
Frequently Asked Questions about Zwitterion
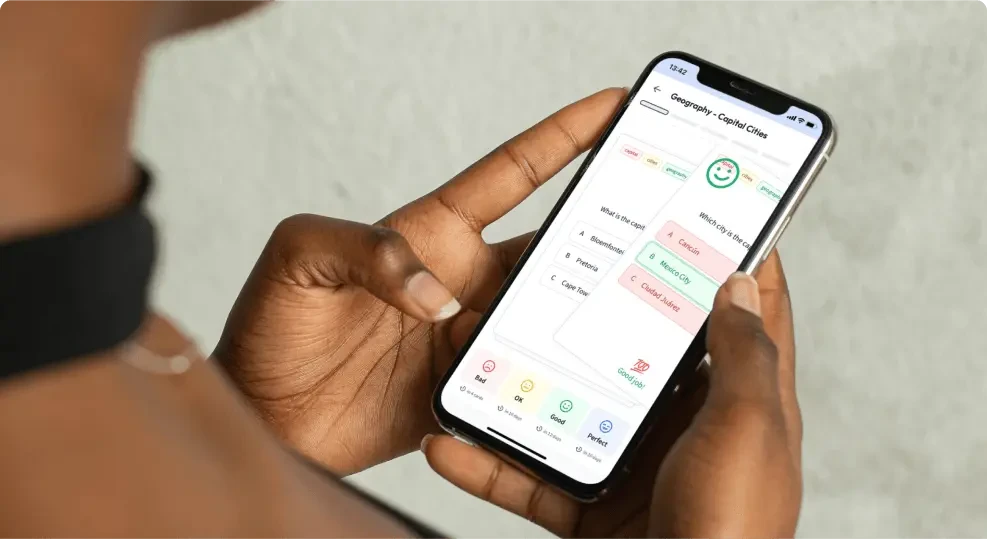
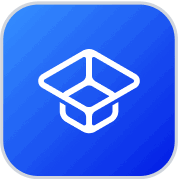
About StudySmarter
StudySmarter is a globally recognized educational technology company, offering a holistic learning platform designed for students of all ages and educational levels. Our platform provides learning support for a wide range of subjects, including STEM, Social Sciences, and Languages and also helps students to successfully master various tests and exams worldwide, such as GCSE, A Level, SAT, ACT, Abitur, and more. We offer an extensive library of learning materials, including interactive flashcards, comprehensive textbook solutions, and detailed explanations. The cutting-edge technology and tools we provide help students create their own learning materials. StudySmarter’s content is not only expert-verified but also regularly updated to ensure accuracy and relevance.
Learn more