Jump to a key chapter
This difference in energy gets absorbed or released by the reaction. Energetics is what we call the study of energy flow in a chemical process. What determines whether a reaction will release or absorb energy? Keep reading to find out!
- In this article, we will consider the energetics of chemical reactions.
- You will discover the difference between enthalpy and heat.
- You'll find out what enthalpy change, bond enthalpy, calorimetry, and Hess’ Law are.
- You will learn about the energetics of nuclear reactions.
- Finally, we will consider energetic efficiency and quality.
What is energetics in chemistry?
Energetics is part of a broader field of chemistry called thermodynamics or thermochemistry. It is all about the energy changes that happen in a chemical reaction, while thermodynamics deals with how these energy changes affect the properties of a chemical system
Energetics is what we call the study of the flow of energy in chemical reactions.
The laws of thermodynamics help us to understand how energy behaves. There are four laws of thermodynamics but we will only consider the first law here:
Energy cannot be created or destroyed, it only converts from one form to another.
Energy exists in different forms that convert from one to the other. You can learn more about the different types of energy in Thermodynamics. In chemical energetics, however, we only care about two forms of energy:
- Chemical energy, stored in bonds between atoms.
- Heat energy, released or absorbed when bonds are broken or made.
The chemical energy stored in the bonds between atoms often converts to heat energy that gets released when a chemical process takes place. A reaction will also absorb heat energy from the surroundings to beak the bonds in the compounds. The overall balance in the absorption and evolution of heat energy in a chemical reaction tells us whether the chemical process is endothermic or exothermic.
What are endothermic and exothermic reactions?
Breaking bonds requires energy in the form of heat. This means that heat energy gets absorbed or taken in from the surroundings when bonds break. On the flip side, making bonds releases heat energy. When more bonds are broken than new bonds formed in a chemical reaction, there is an overall absorption of energy. We call these types of reactions endothermic. On the other hand, when there are more bonds formed than old bonds broken, there is an overall release of energy. We call these types of reactions exothermic.
To clarify, endothermic reactions absorb energy but exothermic reactions release energy. This is all to do with the ratio of bonds broken to bonds formed. Bond breaking is an endothermic process while bond making is an exothermic process. You will learn a little more about the balance between energy release and evolution when we look at enthalpy diagrams later.
Fig. 1 - Energetics of bond-making and bond-breaking
In energetics, you will learn how heat energy transfers in and out of a chemical reaction. More importantly, you will discover enthalpy.
What is enthalpy? What is so special about it and how is enthalpy different from heat? Let us explore further.
What is enthalpy?
Enthalpy (H), or heat content, is the amount of thermal energy (or heat) stored in a system. We measure it in kilojoules per mole (kJ mol-1).
In energetics, we call the reaction mixture the system and everything outside of the system, like the laboratory where you experiment, the surroundings.
When a reaction absorbs energy, the total enthalpy of the system increases. When it releases energy, the total enthalpy decreases. We cannot directly measure the total enthalpy in a system, but we can measure the changes in enthalpy that take place in a chemical reaction.
Enthalpy change (∆H) is the amount of heat energy that is either absorbed or evolved in a chemical reaction under constant pressure.
We use the Greek letter delta '∆' to mean 'change in'. For example, ∆H is change in enthalpy, ∆T is change in temperature, and ∆S is change in entropy.
Enthalpy is something we call a state function variable. State functions are pathway independent, which means that the value of the variable remains the same, regardless of how we arrive at it.
For example, you have three routes you can take on your way home from school. All three paths measure different distances but take you the same amount of time to travel. In this instance, the time it takes you to get home is pathway independent.
State functions allows us to calculate enthalpy change. If we know an alternative route for a reaction, we can calculate the change in enthalpy. Since ∆H is pathway independent, its value will be the same for a reaction, as long as we start with the same reactants and end up with the same products.
How is enthalpy different from heat?
You are probably still curious about how enthalpy differs from heat. Most of the time, when we say ‘enthalpy’ we also mean ‘heat’. In most cases, that is okay, since under a constant pressure and volume, q = H. However, enthalpy and heat are not exactly interchangeable.
Let us begin with heat. Heat is a form of energy. We say it is the energy that flows between a system and its surroundings because of a temperature difference. For example, we know that if we put an ice cube right next to a cup of warm water, heat will flow from the cup of water to the ice.
Heat (q or Q) is the energy that flows between a system and its surroundings due to a temperature difference. Since heat is energy, we measure it in joules.
As we have seen from the example of the ice cube and cup of warm water, heat is energy in motion. Heat always moves from a hot system to a colder one.
Enthalpy is the amount of thermal energy in a system. Heat is what we call the transfer of thermal energy. Enthalpy is an essential part of a system since it depends on the number of molecules, chemical composition, and structure. Meanwhile, heat is energy that crosses a system's boundaries. Heat comes and goes- it is not an essential part of a system.
When heat leaves or enters a system, it results in a change in enthalpy. At a constant pressure heat flow directly relates to enthalpy. Fortunately, we perform most experiments under constant pressure, so we can calculate the change in enthalpy if we know the value of q. You can learn more about this in Calorimetry.
Enthalpy diagrams
We use enthalpy diagrams (also called energy diagrams) to illustrate how enthalpy changes in a chemical reaction.
- We draw the reactants and products at different levels that correspond to their energy.
- We use arrows to show the processes of bond breaking and bond making.
- When bonds break, energy is absorbed. We show this by drawing an upwards arrow.
- When bonds get made, energy is released. We show this by drawing a downwards arrow.
Enthalpy is a specific measure of energy. Here, an enthalpy change equals an energy change.
The energy balance between bond-making and bond-breaking tells us whether a reaction is endothermic or exothermic. You can see how this would work in the enthalpy diagram below. Energy diagrams are valuable because they make it easy to tell whether a reaction is endothermic or exothermic.
Fig. 2 - How to draw an enthalpy diagram
To illustrate this further, have a look at another enthalpy diagram below. It shows the combustion of methane (CH4 + 2O2 → CO2 + 2H2O). We can see from the energy diagram that the energy released in the formation of new bonds is greater than the energy absorbed to break bonds. That makes the combustion of methane an exothermic reaction.
Fig. 3 - Enthalpy diagram- the combustion of methane
You can learn more about enthalpy diagrams in Enthalpy Changes. Now, let us talk about bond enthalpy.
What is bond enthalpy?
In the energy diagram above, the energy absorbed to break each C-H bond and the O-O bond is known as bond enthalpy, or bond energy.
Bond enthalpy (E) is the amount of energy required to break one mole of a specific covalent bond in the gas phase.
The value of energy that evolves when a bond gets made is the same value that gets absorbed when a bond breaks. We can use bond enthalpies to calculate the change in enthalpy of a reaction. When doing calculations involving bond enthalpies, we use an average of all of the bond enthalpies of the same bond in different environments. We call this mean bond enthalpy. You can read more about it in Bond Enthalpy.
Calorimetry
We have mentioned before that if we know the value of q (heat flow), we can calculate the enthalpy change in a reaction.
Calorimetry is a method to measure the enthalpy changes that occur during a chemical reaction.
Simply seal the reacting chemicals inside the container with a thermometer to record any temperature change. We can use this change in temperature to calculate the enthalpy change of the reaction by using the following equation:
q = mcΔT
- q is the energy transferred, measured in joules (J).
- m is the mass of the water in grams (g).
- c is the specific heat capacity (J g-1 ºC-1).
- ΔT is the temperature change measured in Kelvin (K) or degree Celsius (ºC).
Fig. 4 - A simple calorimeter
Specific heat capacity (c) is the energy required to increase the temperature of 1g of a given liquid by 1 °C. For example, water has a specific heat capacity of 4.18 Jg-1 ºC-1. You can learn more about specific heat in Thermal Physics.
You can learn how to conduct a calorimetry experiment and use the results to calculate enthalpy change in Calorimetry. Sometimes it is not possible to use calorimetry to calculate enthalpy changes. When we have this problem we use Hess' Law.
What is Hess’ law?
Hess’ law states that the total enthalpy change for a reaction is independent of the route of the chemical reaction.
We discussed earlier that change in enthalpy is a state function; in other words, enthalpy change is pathway independent. If we know an alternate route for a reaction, we can figure out the enthalpy change. This principle is the application of Hess' Law.
In other words, let us say you have a chemical reaction from reactants A to products B. You could go directly from A to B, or you could go through an intermediate, C. According to Hess' Law, the enthalpy change in reaction A → B is equal to the sum of enthalpy changes in reaction A → C and C → B! The image below helps to illustrate this.
Fig. 5 - Hess' law
We can apply energetics to physical processes as well. Let us now explore the energetics of nuclear reactions and energetic efficiency.
Energetics of nuclear reactions
In nuclear reactions, we notice a visible change in mass when energy changes occur.
Have you ever come across Albert Einstein's equation E = mc2? It explains the relationship between mass and energy change in a reaction. We call it the mass-energy equivalence. What does this have to do with energetics? Every time a change in energy happens, a change in mass also occurs. These changes are linked together by the constant c2 (the speed of light squared).
In Albert Einstein's mass-energy equivalence, 'm' is the net change in mass in kilograms, and 'c' is a constant (the speed of light) in metres per second. The two standard units for expressing nuclear energy are joules (J) and megaelectronvolts (MeV).
The energy changes that happen in chemical processes are insignificant when compared to the amount of energy released by nuclear reactions. Since the energy changes in nuclear reactions are so large, we can observe a visible mass change. This change in mass allows us to calculate the energy changes in nuclear reactions. We do this using the relationship between an atomic mass unit (AMU) and MeV. Scientists have figured out that 1 AMU equals 1.4924 x 10-10 joules, which is the same as 931.5 MeV!
1 amu = 1.4924 x 10-10 j = 931.5 MeV
AMU is how we refer to the mass of an atom. You can learn more in Amount of Substance.
When a nucleus forms, mass is lost and energy gets released as nuclear binding energy. Nuclear binding energy is simply the energy needed to bind protons and neutrons together to make the nucleus. The energy loss is so immense that it shows up as a loss in mass. Just imagine, energy that has mass!
This loss in mass accounts for something known as mass defect. Scientists observed that the sum of the masses of individual protons and neutrons is more than the mass of the nucleus as a whole. In other words, mass defect is where the mass of the individual subatomic particles is more than that of the nucleus.
If you would like to know more, visit Binding Energy.
Energetic efficiency
Energy cannot be created or destroyed, but it converts to other forms of energy like movement, heat, electricity, and sound. What we would really like energy to do, is work. Work can be anything from moving a car, to lighting a room, or baking a cake.
It is not always easy to get energy to do the work we would like for it to do. For example, we would like a cake mixer to spin around, so the batter can be evenly mixed. Most of the electrical energy we supply to the mixer gets turned into kinetic energy that spins the mixer. However, if you have ever used a cake mixer, you know that some of that electrical energy gets converted to sound energy.
Sound energy is not very useful for mixing batter, so we call it wasted energy. Energy efficiency tells us how much of the energy supplied to a system or reaction gets converted to the type of work energy we want it to do.
Energy efficiency tells us the percentage ratio of the energy output to the energy input in a system.
Chemists would like their reactions to be efficient since efficient processes are cheaper, use up fewer resources, produce less waste, and are more sustainable. As an example, the metathesis method is a way to make organic synthesis more efficient. In metathesis, we use an energy-efficient catalyst to make reactions with a high atom economy proceed at standard temperature and pressure. Find out more about metathesis in Organic Synthesis.
Energy Quality
Energy quality measures how easily we can convert one form of energy to another. In most cases, we would like energy to do work. For example, we need the electrical energy supplied to a light bulb to convert to light energy to see in a dark space. However, some of this energy gets lost in the form of heat.
High-quality energy light bulbs like LED bulbs convert electrical energy more efficiently, so that most of the energy transfers to light energy. On the other hand, old-fashioned filament bulbs do a poor job of converting electricity to light. Most of the energy supplied to a filament bulb gets dispersed as heat energy! Low-quality energy cannot be easily used to do work. Usually, this is because the energy is too disordered, or disperses quickly (see Entropy for more information). High-quality energy can easily be converted to do work and is more efficient.
Energetic quality is only a perception of energy. Simply put, the kind of energy you think is high-quality depends on what you want it to do. Not all scientists agree on what types of energy are high quality. Some say that high-quality energy can be transformed into other kinds of energy without much loss, while others say that high-quality energy can be used to do high-quality tasks and low-quality work.
Generally, electricity is seen as high-quality energy because of its efficiency and ability to convert to other kinds of energy. Some scientists see heat energy from burning fuel as low-quality energy, so they think it can only do simple things like boil water.
Energetics - Key takeaways
Energy is either absorbed or released in chemical reactions.
Endothermic reactions absorb energy when the ratio of bonds broken is higher than the bonds made.
Exothermic reactions release energy when the ratio of bonds made is greater than the ratio of bonds broken.
Bond enthalpy is the amount of energy required to break one mole of a covalent bond in the gas phase.
Bond energy is affected by the other atoms in the molecule and thus it can vary from compound to compound. Because of this we use mean bond enthalpies.
Enthalpy change (∆H) is the amount of heat energy absorbed or released in a chemical reaction under constant pressure.
For endothermic reactions, ΔH is positive, and for exothermic reactions ΔH is negative.
Calorimetry is a method to measure the enthalpy changes that occur during a chemical reaction, by measuring the change in temperature.
You can find the enthalpy change from calorimetry by using the equation: q = mcΔT.
Hess' law states that the total enthalpy change for a reaction is independent of the route taken.
References
- Image of a simple calorimeter: https://chem.libretexts.org/Courses/Lumen_Learning/Book%3A_General_College_Chemistry_I_(Lumen)/11%3A_9-_Thermochemistry/11.03%3A_Calorimetry distrubuted under CC BY 4.0(Link: https://creativecommons.org/licenses/by/4.0/)
Learn faster with the 10 flashcards about Energetics
Sign up for free to gain access to all our flashcards.
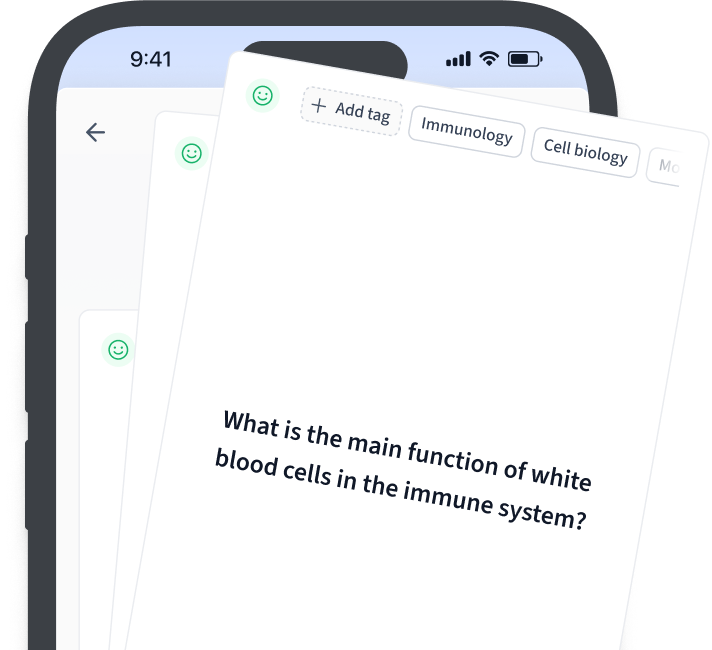
Frequently Asked Questions about Energetics
What is energetics in chemistry?
In chemistry, energetics is what we call the study of the flow of energy in and out of a chemical reaction.
What topics are included in energetics?
In A-Level chemistry, energetics includes:
- Enthalpy changes
- Hess’ Law
- Calorimetry
- Bond enthalpy
Is energetics part of a broader field within chemistry?
Yes, it is part of thermodynamics, or thermochemistry.
What is calorimetry?
Calorimetry is a method to measure the enthalpy changes that occur during a chemical reaction, by measuring the change in temperature.
What happens to energy in a chemical reaction?
Energy is either absorbed or released in a chemical reaction.
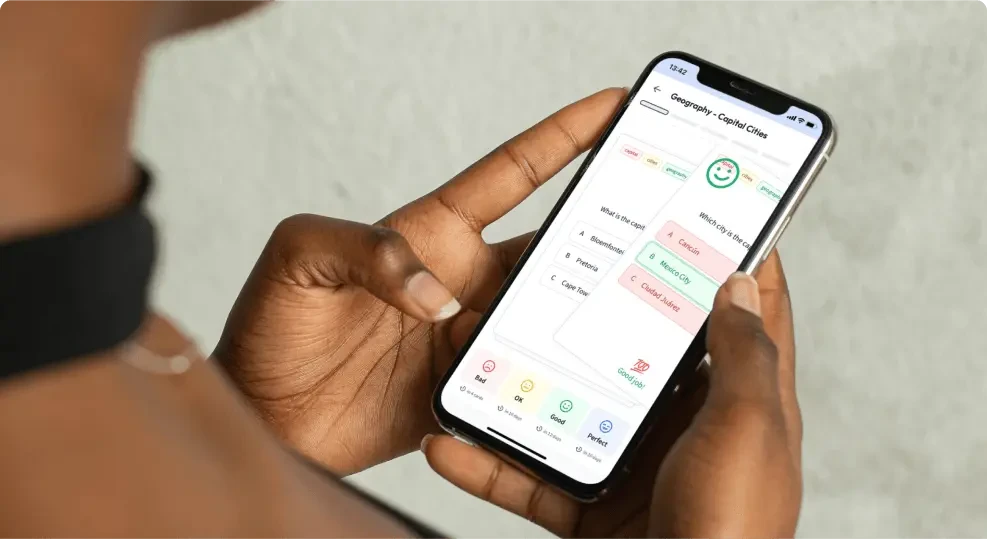
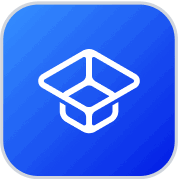
About StudySmarter
StudySmarter is a globally recognized educational technology company, offering a holistic learning platform designed for students of all ages and educational levels. Our platform provides learning support for a wide range of subjects, including STEM, Social Sciences, and Languages and also helps students to successfully master various tests and exams worldwide, such as GCSE, A Level, SAT, ACT, Abitur, and more. We offer an extensive library of learning materials, including interactive flashcards, comprehensive textbook solutions, and detailed explanations. The cutting-edge technology and tools we provide help students create their own learning materials. StudySmarter’s content is not only expert-verified but also regularly updated to ensure accuracy and relevance.
Learn more