Jump to a key chapter
- In this article, you will learn all about enthalpy changes.
- We'll start by defining enthalpy and enthalpy change then discover how enthalpy change relates to endothermic andexothermic reactions.
- We'll then learn how to interpret enthalpy diagrams.
- After that, we'll look at standard enthalpy changes, including the standard enthalpies of reaction, formation, combustion, and neutralisation.
- Finally, we'll explore how you calculate enthalpy change using calorimetry experiments, the formula q = mc∆T, and Hess' law.
Enthalpy change definition
All chemical systems contain energy. We refer to this internal energy as enthalpy.
Enthalpy (H) is a measure of the energy within a system. It is the sum of its internal energy and the product of its pressure and volume. Enthalpy has the units J mol-1 or kJ mol-1.
However, we can't measure enthalpy directly. This is because some of its components aren't easily quantified or accessible. However, we can measure the change in enthalpy.
Enthalpy change (∆H) is the amount of heat energy transferred during a chemical reaction at constant pressure. We also measure enthalpy change in J mol-1 or kJ mol-1.
Whenever a chemical reaction occurs at constant pressure, heat energy is absorbed or released, and thus an enthalpy change takes place. This transfer of heat energy changes the total enthalpy within a system. We have different terms for reactions depending on whether they absorb or release energy; let's look at them now.
Enthalpy changes: Endothermic and exothermic reactions
As we've learned, chemical reactions that take place at a constant pressure result in a change in enthalpy. This means that there is a transfer of heat energy to or from the surroundings. Overall, heat energy is either absorbed or released.
- When a reaction absorbs energy, its total enthalpy increases. This is a positive enthalpy change and is known as an endothermic reaction. Overall, the temperature of the surroundings decreases.
- When a reaction releases energy, its total enthalpy decreases. This is a negative enthalpy change and is known as an exothermic reaction. Overall, the temperature of the surroundings increases.
Here are some everyday examples of endothermic and exothermic reactions:
- Photosynthesis is an endothermic reaction, in which plants use energy from the sun to make essential sugars.
- These sugars are then broken down by (almost all) living organisms in respiration, an exothermic reaction that releases energy in the form of ATP. ATP acts as a molecular currency for all sorts of processes - it is used to build new tissues and power our cells.
- Whenever you bake something, be it a cake, batch of cookies, or a fresh loaf of bread, you often form a golden crust on the outside of the tasty treat. This crust is caused by an endothermic reaction between amino acids and reducing sugars and is known as the Maillard reaction. The Maillard reaction is responsible for all browning of food: the crispy skin of a roast chicken, the caramelised top of a creme brulee, and the black griddle marks on your seared steak.
- Combustion of fuels, such as coal or wood, releases heat energy and so is an exothermic reaction. The heat released during combustion can not only be used directly to heat our homes but also be turned into electrical energy to power our lights, televisions, and power stations.
- It is widely accepted that our universe started almost 14 billion years ago with a massive expansion known as the Big Bang. Initially, this event produced just hydrogen, helium, and trace amounts of lithium and beryllium. All the other elements that we know today were created in endothermic nuclear fusion reactions, that combined lighter elements to make heavier ones.
Enthalpy changes: Enthalpy diagrams
It is easy to see the difference between exothermic and endothermic reactions by illustrating them using reaction profiles or enthalpy diagrams. These diagrams show the relative enthalpy levels of the reactants and products in exothermic and endothermic reactions and thus tell you about the reaction's overall enthalpy change.
Fig. 1 - Enthalpy diagrams for exothermic and endothermic reactions
The y-axis shows enthalpy, while the x-axis shows the extent of the reaction. The dotted lines show the enthalpy levels of the reactants and products. The difference between the levels is the enthalpy change (∆H) as the reactants turn into the products. What differences can you see between endothermic and exothermic reactions?
In endothermic reactions, the products have a higher enthalpy than the reactants.
In exothermic reactions, the products have a lower enthalpy than the reactants.
Endothermic reactions have a positive ∆H, while exothermic reactions have a negative ∆H.
Reaction pathways
Reaction profiles show you a reaction's overall enthalpy change. We see the relative enthalpy of the reactants and the relative enthalpy of the products, but nothing in between. However, what if we want to look at the relative enthalpy of a system at different points within the reaction? To do this, we can instead draw a reaction pathway. You can think of this as a more detailed type of reaction profile. It doesn't just show you the enthalpy at the start and end of a reaction, but throughout the reaction's process as well. For example, you can see how enthalpy changes as bonds break in the reactants and form in the products.
You draw a reaction pathway on similar axes to the ones we used in the enthalpy diagrams above. However, instead of straight upwards or downward arrows, we use a curved line to show the enthalpy change as the reaction progresses.
Here is an example of a reaction pathway.
Fig. 2 - Exothermic reaction profile
You can see that the products have a lower enthalpy than the reactants. This means that the reaction profile shows an exothermic reaction. What else do you notice?
- The enthalpy in the reaction pathway increases as the bonds in the reactants break.
- The enthalpy then peaks. At this peak, where the curve is at its highest point, the amount of enthalpy in the reaction is at its maximum. This is known as the transition state. It represents the point in the reaction where the new bonds are half-made and the old bonds are half-broken.
- Enthalpy decreases as new form bonds to make the products.
- The amount of energy required to break bonds and reach the transition state is less than the amount of energy released when forming new bonds.
The amount of energy between the level of the reactants and the transition state is called the activation energy.
Activation energy is the minimum amount of energy required for a successful reaction.
Simply put, activation energy is the smallest quantity of energy required to get the reactants to the transition state. Without this energy, the reaction can't occur.
Here's an example of an endothermic reaction profile.
Fig. 3 - Endothermic reaction profile
In some ways, an endothermic reaction pathway looks like the opposite of an exothermic reaction pathway. Here, the products have a higher enthalpy than the reactants. But note the similarities between the two.
- The enthalpy in the reaction pathway increases as the bonds in the reactants break.
- The enthalpy then peaks at the transition state.
- Enthalpy decreases as new form bonds to make the products.
- The amount of energy required to break bonds and reach the transition state is greater than the amount of energy released when forming new bonds.
We still require activation energy to get the reaction going and reach the transition state. Without this activation energy, the reaction won't take place. Once the reaction reaches the transition state, it then decreases in enthalpy as new bonds form to make the products.
Standard enthalpy changes
A particular reaction's enthalpy change should always be the same if you carry it out under the same conditions. This means that we can create a database of accurate enthalpy changes for specific reactions. To ensure continuity, we carry out the reactions under the same temperature, pressure, and concentration, to obtain standard enthalpy changes.
Standard enthalpy changes are enthalpy changes measured under standard conditions with the reactants and products in their standard states. We represent them using the symbol ∆Hº.
What are standard conditions? Well, they are defined as:
A temperature of 298 K (or 25 ºC).
A pressure of 100kPa (or 1 bar).
In the case of solutions, a concentration of 1.00 mol dm-3.
What about standard states? It is simple: A species' standard state is simply its physical state under standard conditions. For example, under the standard conditions of 298 K and 100 kPa, oxygen (O2) is a gas. This means that its standard state is gaseous.
Some exam boards define the pressure component of standard conditions as 1 atm. Check with your specification to find out which definition you need to know.
Types of standard enthalpy change
Of course, you can measure enthalpy changes under any conditions, not just standard ones. However, using standard enthalpy changes makes it a lot easier to compare the enthalpy changes of different reactions and carry out calculations involving enthalpy.
For your exams, you need to know about a few different types of standard enthalpy changes. Then, in the article Hess’ Law, you'll learn how you can use these standard enthalpy changes in more complicated enthalpy calculations. The enthalpy changes we will consider here are:
Standard enthalpy change of reaction.
Standard enthalpy change of combustion.
Standard enthalpy change of formation.
Standard enthalpy change of neutralisation.
We often omit the word change from all of these terms, to make them a little shorter. So, instead of saying standard enthalpy change of reaction, you could simply just say standard enthalpy of reaction.
Standard enthalpy change of reaction
The standard enthalpy change of reaction (∆rHº) is the enthalpy change when reactants form products in quantities given in the balanced chemical equation, under standard conditions and with all species in their standard states.
Standard enthalpy change of reaction is a generic term for any enthalpy change that accompanies a chemical equation. You'll often see it written as just ∆Hº - the subscript r is implied. The rest of the standard enthalpies that we'll explore today are all standard enthalpy changes of reaction for specific types of reactions, such as combustion and neutralisation reactions.
Be aware that standard enthalpy change of reaction depends on the molar coefficients in the chemical reaction. If you double all of the reaction coefficients, you double the value of the standard enthalpy change of reaction.
One mole of CaO reacts with one mole of water under standard conditions. The system's enthalpy decreases by 63.7 kJ mol-1.
- Write an equation for this reaction, giving the standard enthalpy change of reaction.
- Write an equation for the similar reaction between three moles of CaO and three moles of water under standard conditions. Once again, give the standard enthalpy change of reaction.
Because this reaction takes place under standard conditions, we know that it is a standard enthalpy change. Here, enthalpy decreases, and so the standard enthalpy change of reaction is negative. Here's our answer:
$$CaO(s)+H_2O(l)\rightarrow Ca(OH)_2(s)\qquad \Delta^{\circ }H=-63.7\space kJ\space mol^{-1}$$
To find an equation for the reaction in part b, we simply multiply our first equation by three. To find the standard enthalpy of reaction for this second reaction, we merely multiply the first standard enthalpy of reaction by three, too.
$$3CaO(s)+3H_2O(l)\rightarrow 3Ca(OH)_2(s)\qquad \Delta^{\circ }H=-191.1\space kJ\space mol^{-1}$$
Standard enthalpy change of formation
The standard enthalpy change of formation (∆fH°) is the enthalpy change when one mole of a species is formed from its elements, under standard conditions and with all species in their standard states.
There are few important things to note about standard enthalpies of formation:
- As we mentioned, ∆fHº values are for the formation of just one mole of a species. This might mean that you have to use fractional molar coefficients for the other species involved in the balanced equation. That is totally OK!
- We say that the ∆fHº value for any element in its standard state is 0.0 kJ mol-1. We then measure ∆fHº values for various compounds from that baseline.
Producing two moles of CaCO3 increases the system's enthalpy by 105.0 kJ mol-1. Write an equation showing the standard enthalpy change of formation of CaCO3.
Well, to form two moles of CaCO3 under standard conditions and with all elements in their standard states, we need two moles of Ca, two moles of C, and three moles of O2. The question tells us that this reaction has an enthalpy change of +105.0 kJ mol-1:
$$2Ca(s)+2C(s)+3O_2(g)\rightarrow 2CaCO_3(s)\qquad \Delta^{\circ }H=+105.0\space kJ\space mol^{-1}$$
However, by definition, standard enthalpy changes of formation only produce one mole of a species. In the equation above, we produced two. Therefore, we need to halve both the balanced reaction equation and the reaction's enthalpy change to find an equation and value for the standard enthalpy change of formation of CaCO3:
$$Ca(s)+C(s)+\frac{3}{2}O_2(g)\rightarrow CaCO_3(s)\qquad \Delta^{\circ }H=+52.5\space kJ\space mol^{-1}$$
Scientists have calculated the standard enthalpies of formation (∆fH°) for many compounds. You can find them on an enthalpy table in your exam; they'll come in handy in later enthalpy change calculations.
Standard enthalpy change of combustion
The standard enthalpy change of combustion (∆cH°) is the enthalpy change when one mole of a substance burns completely in oxygen under standard conditions with all species in their standard states.
Note that all combustion reactions are exothermic. This means that you'll never find a positive standard enthalpy of combustion - they should all be negative!
Most hydrocarbons, such as methane (shown in the example below), have ∆cHº values that are high in magnitude. This means that they release a lot of energy when they are burnt, and thus they make good fuels. You can see a clear trend in ∆cHº values within organic hydrocarbon families. For example, the standard enthalpy of combustion of alkanes increases in magnitude by about 650 kJ mol-1 as you add an extra -CH2 group to the carbon chain.
The equation for the standard enthalpy of combustion of methane is shown below:
$$CH_4(g)+2O_2(g)\rightarrow CO_2(g)+H_2O(l)\qquad \Delta _cH^{\circ }=-890\space kJ\space mol^{-1}$$
Standard enthalpy change of neutralisation
The standard enthalpy change of neutralisation (∆nH°) is the enthalpy change when an acid solution and an alkali solution react under standard conditions to form one mole of water.
Like standard enthalpies of combustion, standard enthalpies of neutralisation are exothermic, and so are always negative. You should also note that these values are for the production of one mole of water. This means that you might need to include fractional molar coefficients in your answer. However, be aware that the acid and alkali are not in standard states, but in solution - their state is (aq).
Here's the standard enthalpy of neutralisation for the reaction between HCl(aq) and NaOH(aq):
$$HCl(aq)+NaOH(aq)\rightarrow H_2O(l)+NaCl(aq)\qquad \Delta _nH^{\circ}=-57.1\space kJ\space mol^{-1}$$
Under standard conditions, all reactions between a strong acid and a strong base have similar ∆nH° values. This is usually between -57 and -58 kJ mol-1. Strong acid-strong base reactions essentially boil down to a reaction between hydrogen ions and hydroxide ions, which always has the same enthalpy change. The other ions that make up the acid or base are just spectator ions and so don't really get involved.
However, weak acid-weak base reactions have varying ∆nH° values that are always slightly less exothermic than those of their stronger relatives. This is because weak acids and bases don't dissociate fully in solution. The overall enthalpy of neutralisation needs to take other enthalpy changes into consideration, such as those involved in ionising the acid or base. Check out Acids and Bases for an introduction to this topic, where you'll learn plenty more about both strong and weak acids and bases.
Enthalpy change: Measuring and formula
We know what enthalpy changes are. But how do we find them?
Well, there are two ways of working out the enthalpy change of a reaction. They're both associated with a certain method and specific formula.
- We can determine enthalpy change experimentally using calorimetry.
- We can calculate enthalpy change theoretically using Hess's law.
To start, let's consider calorimetry.
Calorimetry
Calorimetry is a technique used to measure the heat transfer in a chemical reaction. It is a way of calculating enthalpy change.
Calorimetry, as defined above, is the first way of measuring enthalpy change. It is simple in principle:
- We carry out a chemical reaction and record the temperature change of the solution or a surrounding medium, which is typically water.
- We use the temperature change, mass, and specific heat capacity of the solution or surrounding medium to work out the amount of heat energy released or absorbed during the chemical reaction.
- We then convert the heat energy transferred into enthalpy change.
The calorimetry experiment
We can use calorimetry to approximate many different types of enthalpy changes for many different chemical reactions. The calorimetry experiment itself varies, depending on the type of reaction taking place. You might practice some of these in class. For example, you could burn ethanol underneath a beaker of water and measure the water's temperature change. This will help you find a value for ethanol's enthalpy of combustion. You could also react 1.00 mol dm-3 H2SO4 with 1.00 mol dm-3 NaOH and measure the temperature change of the solution itself. This will help you find a value for the enthalpy of neutralisation.
Fig. 4 - A simple calorimeter which can be used to find enthalpies of neutralisation
However, enthalpy changes calculated using calorimetry are only approximations. Even the best calorimetry experiments include multiple sources of error, many of which are hard to minimise. Whatever your method, it is important to remember the limitations of calorimetry and the measures you should take to make your results as reproducible, reliable, and accurate as possible:
- Enthalpy changes found using calorimetry are often not very accurate. This is mostly due to measurement errors, incomplete reactions, and more often than not, loss of heat to the surroundings.
- We can minimise heat loss by insulating the surrounding medium, using a draft shield (for combustion experiments), and adding a lid to the system. You can see the Styrofoam cup in the diagram above, used to insulate the reaction mixture.
Explore calorimetry experiments in more detail in the article Calorimetry. This article dives into the different calorimetry methods and includes further information about their limitations.
Determining enthalpy change
Once you've carried out your calorimetry experiment, you use your data to calculate the heat energy transferred during the reaction with one simple formula. This formula relates the transfer of heat energy to the change in temperature, mass, and specific heat capacity of the solution or surrounding medium warmed:
$$q=mc\Delta T$$
Here:
- q is the energy transferred, in joules (J).
- m is the surrounding medium's mass, in grams (g).
- c is the surrounding medium's specific heat capacity, in joules per Kelvin or joules per degrees Celsius (J K-1 or J ºC-1).
- ΔT is the surrounding medium's temperature change, in Kelvin (K) or degrees Celsius (ºC).
We can measure the temperature change in either Kelvin or ºC. That is because a change of 1 Kelvin is the same as a change of 1 ºC.
We then calculate enthalpy change. Its units (kJ mol-1) give us a clue as to how we find this value from the amount of heat energy transferred.
- You need to first change the units of your value of heat energy transfered from joules (J) into kilojoules (kJ).
- You then write a balanced equation for the reaction in order to determine the type of enthalpy change you want to find out.
- After that, you use various bits of information, such as the number of moles of each species that reacted and the balanced reaction equation, to work out the enthalpy change.
- Finally, you must deduce whether the reaction was endothermic and exothermic, and thus whether the enthalpy change is positive or negative. If the reaction was exothermic, you add a minus sign to the enthalpy change accordingly.
Remember that endothermic reactions take in heat energy. This is a positive enthalpy change and causes the temperature of the surroundings to decrease. Exothermic reactions, on the other hand, release heat energy. This is a negative enthalpy change and causes the temperature of the surroundings to increase.
Have a go at calculating enthalpy change with the help of the example below.
0.068 moles of potassium iodide dissolve in 48g of water, resulting in a temperature decrease of 14.8ºC. Calculate the enthalpy change of reaction for this dissolution in kJ mol-1. Water has a specific heat capacity of 4.48 J g-1 K-1.
First, we use the equation \(q=mc\Delta T\) to calculate the amount of heat energy transferred during the reaction. Here, m = 48, c = 4.48, and ΔT = 14.8:
$$\begin{align} q&=48\times 4.48\times 14.8\\ q&=3182.6\space J \end{align} $$
Let's change this into kJ by dividing by 1000:
$$3182.6\div 1000=3.1826\space kJ$$
We now write a balanced equation for this reaction:
$$KI(s)\rightarrow K^+(aq)+I^-(aq)$$
We know that enthalpy changes of reaction are measured according to the quantities given in the balanced chemical equation. Here, the equation features just one mole of KI, and so we need to work out the enthalpy change per mole of KI. We dissolved just 0.068 moles of KI, which resulted in a transfer of 3.1826 kJ of heat energy. Therefore, dissolving 1 mole of KI would result in a transfer of \(3.1826\div 0.068\) kJ of energy:
$$3.1826\div 0.068=46.8\space kJ$$
This reaction was endothermic - the temperature of the surroundings decreased. This means that the enthalpy change of reaction is positive. Hence, our final answer is +46.8 kJ mol-1.
Calorimetry calculations going in one ear and out the other? You can find further examples of using calorimetry to calculate enthalpy change in Measuring Enthalpy Changes.
Hess's law
For some reactions, calculating enthalpy change using calorimetry can be a little tricky. Perhaps the quantities of reactants that you are using are simply too small to accurately measure. You might not even have all of the reactants - you could just want to find the enthalpy change hypothetically. Fortunately, you don't have to carry out calorimetry experiments each time you want to know a new enthalpy change. You can instead calculate a theoretical value using enthalpy changes that have already been scientifically determined, by taking advantage of Hess's law.
Hess's law states that the enthalpy change of a chemical reaction is always the same, regardless of the route taken from reactants to products.
For example, what if we want to create product B from reactant A? We can do this in one step:
$$A\rightarrow B\qquad \Delta H_1$$
However, we can also do this in multiple steps:$$\begin{align} A&\rightarrow X\qquad \Delta H_2\\ X&\rightarrow Y\qquad \Delta H_3\\ Y&\rightarrow B\qquad \Delta H_4\end{align}$$
In both cases, we started with the same reactant (A) and ended with the same product (B). The only difference was the route we took to get from one to the other - the first route took us directly from A to B, whilst the second took us via some intermediates. Well, Hess's law tells us that the enthalpy changes of these two routes are the same. Therefore, we can find the enthalpy change of the first route by adding up all of the enthalpy changes in the second route:
$$\Delta H_1=\Delta H_2+\Delta H_3+\Delta H_4$$
Head over to Hess' Law for a full explanation of this idea, including worked examples for many different types of enthalpy change.
Enthalpy changes - Key takeaways
- Enthalpy (H) is a measure of the amount of thermal energy (heat) in a chemical system. It is measured in J mol-1 or kJ mol-1.
- Enthalpy change (∆H) is the amount of heat energy transferred during a chemical reaction at constant pressure. It is also measured in J mol-1 or kJ mol-1.
- Reactions can be endothermic or exothermic.
- Endothermic reactions absorb heat energy. They have a positive enthalpy change and decrease the temperature of the surroundings.
- Exothermic reactions release energy. They have a negative enthalpy change and increase the temperature of the surroundings.
- We can use enthalpy diagrams and reaction pathways to show the enthalpy change of a reaction. These diagrams show the relative enthalpy levels of the reactants and products.
- Standard enthalpy changes (ΔH°) are enthalpy changes measured under standard conditions and with all species in their standard states.
- Standard conditions are defined as a temperature of 25ºC or 298K, a pressure of 1 bar or 100kPa, and a concentration of 1.00 mol dm-3.
- Standard state is the physical state of a species under standard conditions.
- We can calculate enthalpy changes using calorimetry experiments and Hess' law.
- Calorimetry is a technique used to measure the transfer of heat energy in a chemical reaction. We calculate heat energy transfer using the equation \(q=mc\Delta T\), then use this value to work out enthalpy change.
- Hess' lawstates that the enthalpy change of a chemical reaction is always the same, regardless of the route taken from reactants to products. We use scientifically-verified enthalpy changes that we do know in order to work out enthalpy changes that we don't know.
Learn faster with the 2 flashcards about Enthalpy Changes
Sign up for free to gain access to all our flashcards.
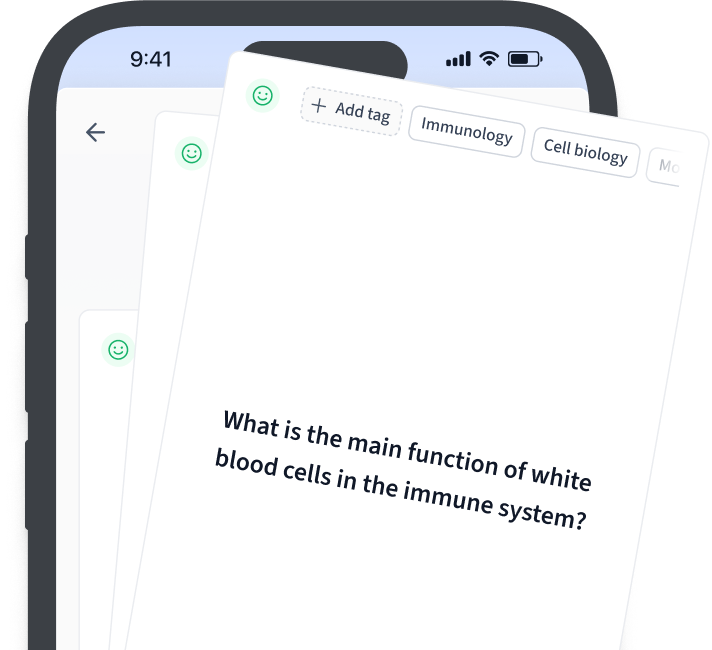
Frequently Asked Questions about Enthalpy Changes
How do you calculate standard enthalpy change?
You can calculate standard enthalpy change by conducting calorimetry under standard conditions, with all of the reactants and products in their standard states. Scientists have done much of the hard work for us by determining lots of standard enthalpy changes already, and so we don’t have to conduct a calorimetry experiment every time we want to know the enthalpy change of a reaction. We can simply look up many standard enthalpy change values in an enthalpy table.
How do you calculate the enthalpy change of combustion?
You can calculate the enthalpy change of combustion by using the enthalpies of formation of the products and reactants in the equation. This is an example of Hess' law. Here's the equation:
∆cH° = ∆fH°(reactants) - ∆fH°(products)
What is enthalpy change?
Enthalpy change (∆H) is the amount of heat energy transferred in a chemical system at constant pressure.
How do you calculate the enthalpy change of formation?
Scientists calculate standard enthalpy changes of formation using calorimetry experiments and mean bond enthalpies. You don't need to work these out yourself - you'll be given any necessary enthalpies of formation in the exam. However, you can use enthalpies of formation to calculate other enthalpy changes by applying Hess' law. You'll learn about this more in the article with the same name, but here's the equation if you are curious:
∆rH° = ∆fH°(products) - ∆fH°(reactants)
How do you calculate enthalpy change?
You can use calorimetry and the equation q = mc∆T to calculate enthalpy change. You can also use Hess' law.
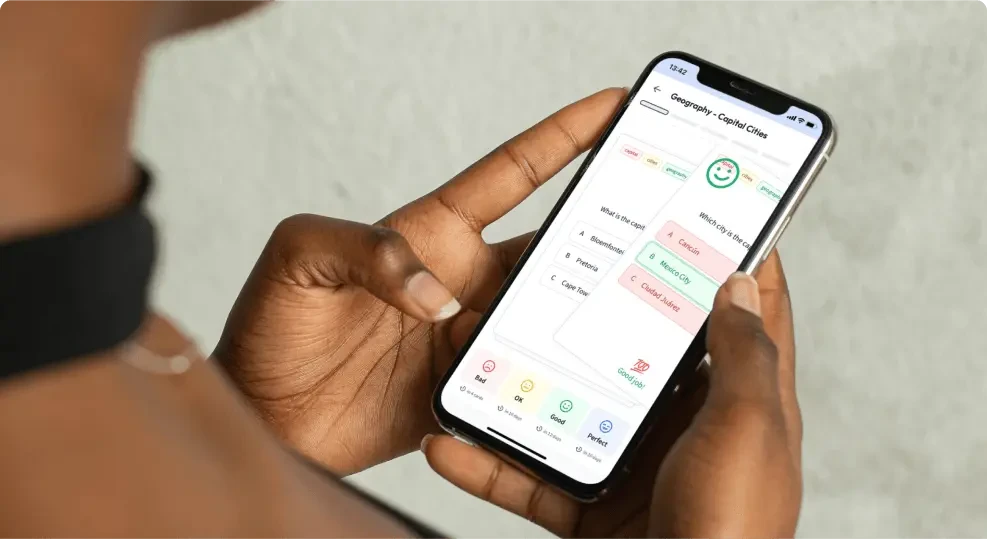
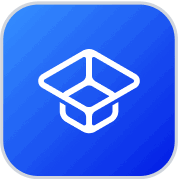
About StudySmarter
StudySmarter is a globally recognized educational technology company, offering a holistic learning platform designed for students of all ages and educational levels. Our platform provides learning support for a wide range of subjects, including STEM, Social Sciences, and Languages and also helps students to successfully master various tests and exams worldwide, such as GCSE, A Level, SAT, ACT, Abitur, and more. We offer an extensive library of learning materials, including interactive flashcards, comprehensive textbook solutions, and detailed explanations. The cutting-edge technology and tools we provide help students create their own learning materials. StudySmarter’s content is not only expert-verified but also regularly updated to ensure accuracy and relevance.
Learn more