Jump to a key chapter
Understanding Solubility Equilibria
Solubility equilibria is a crucial concept in chemistry that applies to the dissolution of substances and the formation of solutions. Understanding this principle will empower you with the ability to predict how substances interact in various environments, which is essential for fields ranging from pharmaceuticals to environmental science.
What is Solubility Equilibria?
When a solute dissolves in a solvent, an equilibrium is established between the undissolved solute and the ions or molecules in solution. This dynamic state of balance, known as solubility equilibria, describes the point at which the rate of solute dissolving equals the rate of the solute coming out of the solution, or precipitating. It is a special case of chemical equilibrium focused exclusively on the process of dissolution and precipitation.At solubility equilibrium, the concentration of the solute in the solution remains constant under a given set of conditions, such as temperature and pressure. This concentration is known as the solubility of the substance. The solubility is often expressed in terms of molarity (moles per litre) or grams per litre. The relationship between the dissolved ions and the undissolved solute can be represented by a solubility product constant (\( K_{sp} \)), which is specific to each substance at a fixed temperature.
Basic Concepts of Solubility Equilibria
To delve deeper into solubility equilibria, you must familiarise yourself with several key terms and concepts: Solubility: It is the maximum amount of a substance (solute) that can be dissolved in a solvent at a given temperature and pressure to form a saturated solution. Saturated Solution: A solution that contains the maximum concentration of solute that can dissolve at a particular temperature and pressure. Unsaturated Solution: A solution that contains less solute than the maximum amount that can dissolve under the existing conditions. Supersaturated Solution: A solution that contains more dissolved solute than would be in equilibrium with the undissolved substance; these solutions are unstable and the excess solute can precipitate out. Solubility Product Constant ( \( K_{sp} \\)): A mathematical expression that relates the concentrations of the ions in a saturated solution of the solute. For a generic salt AB dissolving as \( A^{n+} \\)+ \( B^{m-} \\), the solubility product constant would be defined as \( K_{sp} = [A^{n+}]^n[B^{m-}]^m \\). Common Ion Effect: The phenomenon where the solubility of a salt decreases in the presence of another salt in solution that shares a common ion.
Ionic Product: This is similar to the solubility product, but it differs in that it represents the product of the ion concentrations of a solution that's not necessarily saturated. When the ionic product is greater than the solubility product, precipitation will occur.
Consider \( CaF_{2} \\), which dissolves in water according to the equation: \( CaF_{2(s)} \leftrightarrow Ca^{2+}_{(aq)} + 2F^{-}_{(aq)} \\). The solubility product constant ( \( K_{sp} \\)) expression for this process would be \( K_{sp} = [Ca^{2+}][F^{-}]^2 \\).
Solubility Equilibria and the Phase Rule
The Phase Rule, formulated by Josiah Willard Gibbs, is a general principle in thermodynamics that provides a systematic framework for understanding the number of degrees of freedom in a closed system at equilibrium. The rule is expressed as: \( F = C - P + 2 \\), where \( F \\) is the number of degrees of freedom, \( C \\) is the number of components, and \( P \\) is the number of phases. Within the context of solubility equilibria, the Phase Rule aids in elucidating the constraints on a system’s variables that will influence the solubility of a substance. Components: In solubility equilibria, components usually refer to the solute and solvent. For example, in a system where \( NaCl \\) is being dissolved in water, there are two components. Phases: These are distinct forms of matter, such as the solid solute, the liquid solvent, and the aqueous solution containing the dissolved ions. Degrees of Freedom: This concept details the number of variables, such as temperature and concentration, which can be changed independently without altering the phase equilibrium of the system. Applying the Phase Rule to solubility equilibria allows you to predict the effect of changing external conditions on a system. For example, adding more solute or altering the temperature can shift the equilibrium, thus changing the solubility.
Le Chatelier's Principle also applies to solubility equilibria. When a system at equilibrium undergoes a change in concentration, temperature, or pressure, the system adjusts to counteract the imposed change and a new equilibrium is established.
Solubility Equilibria of Ionic Compounds
Solubility equilibria refer to the dynamic balance that occurs when an ionic compound dissolves in a solvent. At equilibrium, the rate of the solid ionic compound dissolving to form ions is equal to the rate of the ions coming together to reform the solid. This equilibrium is fundamental in understanding the solubility of various substances, which in turn is vital in predicting how these substances will behave in different solutions. The extent of dissolution and the concentration of ions in a saturated solution can be quantitatively described by the solubility product constant ( \( K_{sp} \ )).
Solubility Product Constant (Ksp)
The solubility product constant, often abbreviated as \( K_{sp} \ ), is a mathematical representation of the solubility equilibrium for sparingly soluble ionic compounds. When a substance reaches its solubility limit in a solution, any additional substance will fail to dissolve and will remain as a solid. This saturated solution is at equilibrium, and the product of the concentrations of the dissolved ions raised to the power of their stoichiometric coefficients equals the \( K_{sp} \ ). The expressions for \( K_{sp} \ ) depend on the specific ionic compound under consideration. In water, for example, a generic salt \( AB \ ) dissociates into A and B ions. The \( K_{sp} \ ) would then be written as \( K_{sp} = [A^m+]^n[B^n-]^m \ ). It is important to keep in mind that \( K_{sp} \ ) values are temperature sensitive and can change substantially with temperature shifts.
- The units of \( K_{sp} \ ) are determined based on the ionic charges and are not necessarily consistent across different compounds.
- \( K_{sp} \ ) does not give direct information about the actual concentrations of ions in a solution, but it allows for the calculation of the saturation concentration of the ionic compound.
- The larger the \( K_{sp} \ ), the more soluble the compound is in the solvent.
Saturation Point: The point in a solution where the maximum amount of solute has been dissolved, and any additional solute will not dissolve in the solvent under the given conditions. The concentrations of the dissolved ions at this point reflect the solubility of the ionic compound.
Let us consider \( CaF_{2} \ ), which dissociates according to the equation: \( CaF_{2(s)} \ ) \( \leftrightarrow \ ) \( Ca^{2+}_{(aq)} + 2F^{-}_{(aq)} \ ). The \( K_{sp} \ ) for \( CaF_{2} \ ) at a certain temperature might be, for instance, \( 3.45 \times 10^{-11} \ ). This translates to the expression \( K_{sp} = [Ca^{2+}][F^{-}]^2 = 3.45 \times 10^{-11} \ ). The brackets denote the molarity, or concentration, of each ion in moles per litre.
Common Ion Effect on Solubility Equilibria
The common ion effect is a phenomenon that occurs when a solute is dissolved in a solution that already contains one of the ions present in the solute. This has a significant impact on solubility equilibria because the presence of the common ion suppresses further dissolution of the solute. This effect can be explained by Le Chatelier’s Principle, which states that if a change occurs in any of the conditions of a system in equilibrium, the system will adjust itself to counteract the change. For example, if sodium chloride ( \( NaCl \ )) is added to a solution that already contains sodium ions ( \( Na^+ \ )) from another source, the additional \( Na^+ \ ) ions will shift the equilibrium position of the dissolution reaction, effectively reducing the solubility of the \( NaCl \ ). The already-present \( Na^+ \ ) ions contribute to the ionic product of \( NaCl \ ), but since \( NaCl \ ) is a strong electrolyte and thus fully dissociates, the effect is most pronounced with sparingly soluble ionic compounds.
Compound | Without Common Ion | With Common Ion |
CaF2 | Higher solubility | Lower solubility due to common ion F- |
AgCl | Higher solubility | Lower solubility due to common ion Cl- |
- Adding a salt that shares an ion with the dissolved substance will decrease the solubility of that substance.
- The effect is applicable to all types of reactions involving ions, including precipitation, acid-base, and complex formation reactions.
- Understanding the common ion effect is essential for controlling precipitations and separations in both analytical and industrial chemistry applications.
The magnitude of the common ion effect is influenced by the concentration of the common ion present in the solution. A higher concentration will have a greater impact on reducing the solubility of the original salt.
Acid Base Equilibria and Solubility Equilibria
The interplay between acid-base equilibria and solubility equilibria significantly impacts the solubility of substances. Acid-base reactions can alter the pH of a solution, which influences the solubility of various compounds, particularly those that can react with either protons (H+) or hydroxide ions (OH-). Understanding these relationships is essential in areas such as pharmaceutical drug design, environmental chemistry, and industrial processes where solubility plays a critical role.
The Role of pH in Solubility Equilibria
pH, the measure of acidity or basicity of a solution, is a critical factor in the solubility equilibria of compounds, especially for salts containing acidic or basic ions. Some substances are more soluble in acidic solutions, while others are more soluble in basic conditions due to the formation or consumption of H+ or OH- ions in solution. For compounds that contain anions from weak acids, such as carbonates (CO32-) or phosphates (PO43-), solubility increases as the pH is lowered. The addition of H+ ions to the solution causes a shift in the equilibrium by reacting with the anions to form the undissociated weak acid, which is typically less soluble. Conversely, the solubility of compounds containing cations from weak bases, such as aluminium hydroxide (Al(OH)3), increases in a basic environment. The solubility of a compound in relation to pH can be better understood by writing out the relevant chemical equilibrium reactions. Taking the dissolution of calcium fluoride (CaF2) as an example, in an acidic solution, fluoride ions (F-) react with H+ to form hydrogen fluoride (HF), a weak acid. This reaction removes F- from the solution, shifting the equilibrium to dissolve more CaF2 into the solution. The equation representing this process is as follows: \[ CaF_{2(s)} + 2H^{+}_{(aq)} \rightleftharpoons Ca^{2+}_{(aq)} + 2HF_{(aq)} \] As a result, the solubility product expression for CaF2 becomes dependent on both the concentration of calcium ions and the concentration of H+ in the solution. The general expression for the effect of pH on the solubility product is: \[ K_{sp}' = K_{sp} \times \left(\frac{1}{[H^{+}]^n}\right) \] where \( K_{sp}' \) represents the apparent solubility product in the presence of a certain concentration of H+, \( K_{sp} \) is the intrinsic solubility product constant of the compound, and \( n \) is the stoichiometric coefficient of H+ in the reaction. This analysis of solubility in relation to pH is particularly important when considering the bioavailability of medications in different environments of the human body, which vary in pH, or the mobility of pollutants in natural waters.
Solubility-PH Profile: A graph or chart that shows the solubility of a compound as a function of the pH of the solution. It is particularly useful for assessing the dissolution behaviour of pharmaceutical compounds under various pH conditions.
Remember that a high pH corresponds to a basic solution, whereas a low pH indicates an acidic solution. The pH scale typically ranges from 0 to 14.
The Influence of Acid-Base Reactions
Acid-base reactions are a subset of chemical reactions where an acid releases protons (H+) that are accepted by a base. The classic definition was given by Brønsted and Lowry, who defined acids as proton donors and bases as proton acceptors. This theory is particularly useful in explaining changes in solubility equilibria because some compounds can undergo acid-base reactions within the solution, affecting their solubility. The influence of these reactions on solubility equilibria becomes evident when dealing with salts derived from weak acids or bases. An acidic pH can often lead to the protonation of anions, resulting in the formation of neutral, less soluble molecules or ions that are less able to remain dissociated in solution. The reverse is true for cations of weak bases; they tend to form neutral molecules in the presence of OH-, which can precipitate out of solution. This can be strategically used to control solubility and promote selective precipitation in qualitative analysis and separation techniques. Considering hydroxides, such as Fe(OH)3, which is sparingly soluble, its solubility will be influenced by the pH of the solution. In a basic environment with excess OH- ions, the equilibrium is shifted to form more of the insoluble hydroxide. \[ Fe^{3+}_{(aq)} + 3OH^{-}_{(aq)} \rightleftharpoons Fe(OH)_{3(s)} \] In contrast, in acidic solutions, the H+ ions will react with the OH- ions, forming water and shifting the equilibrium to dissolve more Fe(OH)3. This relationship illustrates how acid-base reactions, and therefore pH, directly influence solubility outcomes. It is the manipulation of such equilibria that underpins titration methods in analytical chemistry and allows for the tailoring of solubility in pharmaceutical formulation. Moreover, complex formation reactions, an extension of acid-base chemistry, also affect the solubility of compounds. Formation of a soluble complex/ion with a ligand can significantly increase the solubility of an otherwise insoluble compound by changing both the chemical species present and the dynamics of the equilibrium, as seen with chelating agents in metal extractions.
Factors Influencing Solubility Equilibria
A solid understanding of the factors that affect solubility equilibria is essential for predicting how substances will dissolve in various media. Solubility equilibria, which describe the balance between undissolved solids and dissolved ions, are influenced by environmental conditions such as temperature and pressure. Comprehending these influential factors enables one to manipulate conditions to achieve desired solubility outcomes, which is crucial in fields like chemistry, pharmacology, and environmental science.
Temperature and Its Effect on Solubility Equilibria
Temperature is one of the main factors that can significantly alter the solubility equilibria of a substance. As temperature increases, the kinetic energy of the molecules within a solution increases. This generally leads to an increase in the solubility of solids and liquids, as more solute particles are dissipated into the solvent; however, for gases, solubility tends to decrease with a rise in temperature. Looking closely at the dissolution of solids, raising the temperature typically disrupts the lattice structure of the solid, making it easier for the solvent to solvate and disperse the solute molecules or ions. This endothermic process absorbs heat, following the principle of Le Chatelier which predicts that the system will shift towards dissolving more solute when temperature is increased. Conversely, if the dissolution is exothermic, sometimes observed with certain ionic salts, an increase in temperature can actually reduce solubility as the system will release heat and favour the formation of the undissolved solid.It's important to note that the temperature effect is substance-specific. Each solute-solvent combination will have its own unique solubility-temperature relationship, often represented graphically in a solubility curve. These curves demonstrate that while the relationship is generally linear for most substances, some can display nonlinear solubility increases or even decreases at higher temperatures.
Solute | Effect of Increasing Temperature |
Common Salts (NaCl, KCl) | Usually a moderate increase in solubility |
Gases (O2, CO2) | Decrease in solubility |
Compounds with highly ionic bonds (e.g., CaSO4) | Can decrease if dissolution is exothermic |
- Temperature changes can also affect the solubility of compounds through changes in the solution’s pH and ionic strength.
- The saturation point for a given solute also varies with temperature, meaning that a solution can become unsaturated or supersaturated solely due to temperature change without any change in the amount of solute present.
Le Chatelier’s Principle: A fundamental concept in chemistry which states that if a dynamic equilibrium is disturbed by changing the conditions, the position of equilibrium moves to counteract the change.
For instance, calcium chloride (CaCl2) is an example of a salt whose solubility increases with temperature. At 20°C, the solubility might be around 74.5 g per 100 ml of water, which increases to 159 g at 100°C. The process can be described by the reaction: \[ CaCl_{2(s)} \rightleftharpoons Ca^{2+}_{(aq)} + 2Cl^{-}_{(aq)} \] An increase in temperature shifts the equilibrium to the right, improving solubility, as indicated by Le Chatelier's Principle.
When designing experiments or processes requiring precise solubility control, always consider temperatures provided in solubility data, as assumptions based on room temperature may not hold true at higher or lower temperatures.
The Impact of Pressure on Solubility
While pressure has a pronounced effect on the solubility of gases, it has little impact on the solubility of liquids and solids. This phenomenon is described by Henry's Law, which states that the solubility of a gas in a liquid is directly proportional to the pressure of the gas above the liquid. As pressure increases, more gas molecules are 'forced' into the solution, increasing solubility. Once dissolved, the gas continues to exert pressure, achieving equilibrium between the gas molecules in the liquid and those in the gas phase. The \( K_{H} \) or Henry's Law constant is unique for each gas-solvent combination and is temperature-dependent. Unlike the solubility product constant for ionic compounds ( \( K_{sp} \)), Henry's Law constants give the solubility in terms of pressure.
- In the context of solubility equilibria, the practical applications of pressure effects are mostly seen in industries dealing with carbonated beverages and in the ocean where increased pressure at greater depths affects the solubility of atmospheric gases in water.
- It's also significant in medicinal chemistry and environmental engineering for delivering gases to the bloodstream or for understanding the release of gases from natural bodies of water due to changes in atmospheric pressure.
Solute Type | Impact of Pressure on Solubility |
Gases | Direct proportionality to pressure |
Liquids/Solids | Minimal impact, except in special cases |
Henry’s Law: A gas law that states that at a constant temperature, the amount of gas that dissolves in a volume of liquid is directly proportional to the partial pressure of that gas in contact with the liquid, given by: \[ C = kP \] where \( C \) is the solubility (concentration) of the gas, \( k \) is the Henry's Law constant, and \( P \) is the partial pressure of the gas.
Consider carbonated water, where the concentration of dissolved carbon dioxide ( \( CO_{2} \)) is directly related to the pressure of \( CO_{2} \) in the gas phase. At 1 atmosphere of pressure, the concentration of \( CO_{2} \) in the water might be 0.034 mol/L, but at 2 atmospheres, this could double, assuming temperature remains constant: \[ C_{1} = kP_{1} \\ C_{2} = kP_{2} \] If \( P_{2} = 2P_{1} \), then \( C_{2} = 2C_{1} \), displaying direct proportionality.
A thought-provoking application of pressure influence on solubility can be found in deep-sea ecosystems. At such depths, the pressure can be enormous, greatly affecting the solubility of gases in the water. This increased solubility can influence the distribution of marine life, as some organisms have adapted to high concentrations of dissolved gases. In the case of oxygen, which is crucial for aerobic respiration, organisms living at extreme depths must adapt to these altered conditions. Similarly, the higher solubility of gases like nitrogen at depth must be accounted for in the design of diving equipment to prevent decompression sickness, also known as 'the bends'. The careful calculation and application of pressure and solubility data are essential for ensuring the safety of divers and the proper functioning of submersible vehicles in such high-pressure environments.
Solubility Equilibria Examples and Applications
Solubility equilibria play a pivotal role in various sectors such as pharmaceuticals, environmental science, and chemical manufacturing. This balance between dissolved ions and undissolved solids in a saturated solution often dictates the behaviour and availability of substances in numerous contexts. By understanding and applying the principles of solubility equilibria, one can predict and manipulate the solubility of compounds to meet specific needs. For example, the solubility product constant (\( K_{sp} \)) provides insight into the potential saturation levels in pharmaceutical preparations or in removing contaminants from water sources.
Real-world Examples of Solubility Equilibria
Solubility equilibria are not an abstract concept but instead, find practical application in many daily scenarios. Medications, for example, rely on solubility equilibria to ensure drugs are adequately absorbed into the bloodstream at intended rates. In environmental chemistry, the dissolution of minerals in water bodies affects the availability of nutrients and the removal of pollutants. In the food industry, solubility equilibria determine the shelf-life and stability of products like soft drinks, where the dissolve of carbon dioxide is closely monitored.Some specific instances of solubility equilibria in action include:
- The treatment of hard water, where calcium and magnesium ions are removed through precipitation with washing soda, is based on the manipulation of solubility equilibria.
- In the brewing of coffee, where the desirable flavors are solubilized while unwanted compounds remain undissolved.
- The development of photographic film, which relies on the controlled precipitation of silver halide crystals.
Real-World Scenario | Role of Solubility Equilibria |
Water Treatment | Precipitation of heavy metal ions |
Medication Formulation | Optimization of drug bioavailability |
Consider solubility equilibria beyond just the laboratory; it's the reason behind the crystal-clear appearance of your tap water and even the fizz in your favorite carbonated drinks.
Investigating further into environmental applications, solubility equilibria are central to understanding bioavailability of trace metals in aquatic systems. Trace metals such as iron, copper, and zinc are essential to biological processes but are often present in forms that are not readily available to organisms. The solubility equilibria of these metals, often tied to the presence of chelating agents and the pH of the water, can govern their uptake by organisms. Moreover, the remediation of contaminated soils through processes like phytoremediation hinges on changing the solubility equilibria to make pollutants more or less bioavailable. In the realm of art, conservation scientists need to take into account solubility equilibria when deciding on the appropriate methods to prevent deterioration or when applying restoration techniques to cultural artefacts.
Solubility and Simultaneous Equilibria in Everyday Chemicals
The subject of solubility equilibria extends to simultaneous equilibria when a compound can participate in more than one equilibrium process. A prevalent example is calcium carbonate found in eggshells and seashells. It is involved in the equilibrium involving its dissolution to form calcium and carbonate ions, and a simultaneous equilibrium where carbonate ions are in dynamic balance with carbonic acid and carbon dioxide in water. \[ CaCO_{3_{(s)}} \rightleftharpoons Ca^{2+}_{(aq)} + CO_{3^{2-}}_{(aq)} \] \[ CO_{3^{2-}}_{(aq)} + H_{2}O \rightleftharpoons HCO_{3^{-}}_{(aq)} + OH^{-}_{(aq)} \] Such concurrent solubility equilibria are also crucial in the formation of buffer solutions, where a weak acid and its conjugate base, or a weak base and its conjugate acid, maintain the pH of a solution. Considering cooking, the process of tenderizing meat with marinades often involves acidic components that can shift the solubility equilibria related to protein denaturation and collagen breakdown.
Substance | Solubility Equilibria Applications |
Baking Soda ( \( NaHCO_{3} \)) | Provides CO2 upon heating for leavening in baking |
Table Salt ( \( NaCl \)) | Maintains ion balance in the body, crucial for fluid regulation |
- Simultaneous equilibria come into play when antacids, which contain bases like calcium carbonate, neutralize stomach acid through multiple equilibria involving the dissociation of the antacid and the acid-base neutralization.
- In the ecosystem of a freshwater lake, solubility and simultaneous equilibria influence the concentration of nutrients and minerals, which are essential for aquatic life.
Solubility Equilibria - Key takeaways
- Definition of Solubility Equilibria: A dynamic state where the rate of solute dissolving equals the rate of solute precipitating, with solubility product constant (\( K_{sp} \\) relating dissolved ions and undissolved solute.
- Concepts of Saturated, Unsaturated, and Supersaturated Solutions: Respectively, these are solutions that contain the maximum, less than the maximum, and more than the maximum solubility limit of the solute at given conditions.
- Acid-Base Equilibria's Impact: The pH of a solution can significantly influence the solubility of compounds, particularly those with weak acidic or basic ions, affecting compounds’ solubility.
- Factors Affecting Solubility Equilibria: Temperature, which generally increases solubility for solids and liquids and decreases for gases, and pressure, which significantly impacts the solubility of gases (Henry's Law).
- Real-World Solubility Equilibria Applications: These include the optimization of drug bioavailability, water treatment processes, and the stabilization of carbonated beverages.
Learn faster with the 26 flashcards about Solubility Equilibria
Sign up for free to gain access to all our flashcards.
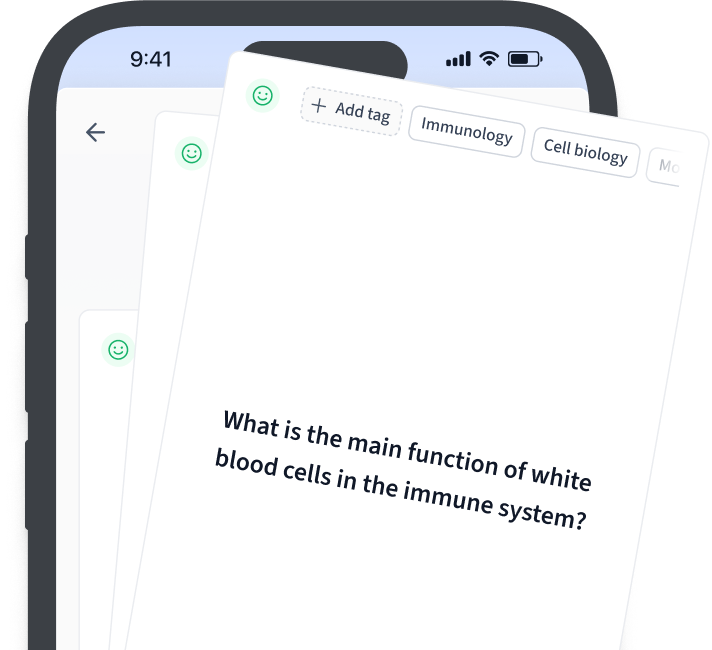
Frequently Asked Questions about Solubility Equilibria
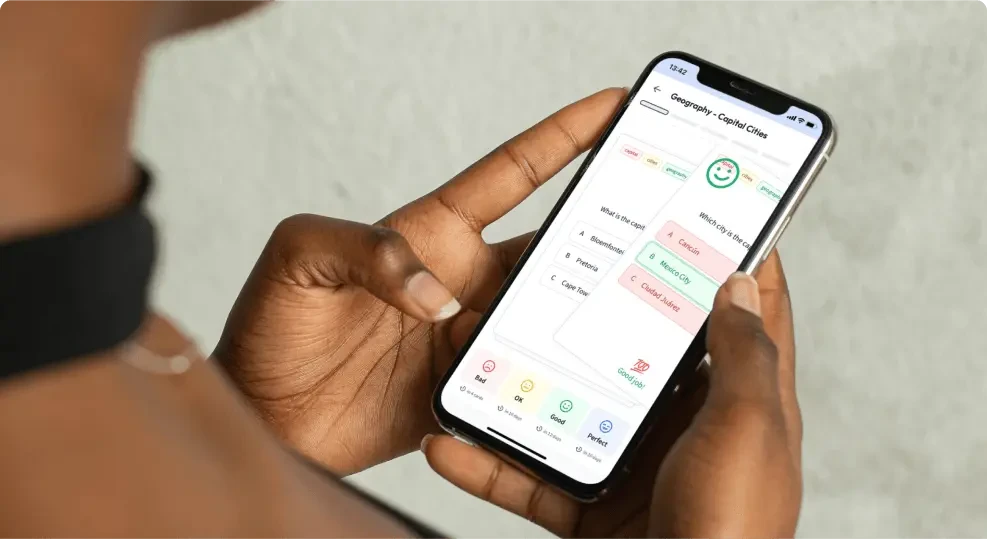
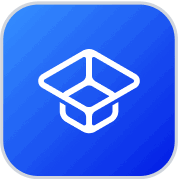
About StudySmarter
StudySmarter is a globally recognized educational technology company, offering a holistic learning platform designed for students of all ages and educational levels. Our platform provides learning support for a wide range of subjects, including STEM, Social Sciences, and Languages and also helps students to successfully master various tests and exams worldwide, such as GCSE, A Level, SAT, ACT, Abitur, and more. We offer an extensive library of learning materials, including interactive flashcards, comprehensive textbook solutions, and detailed explanations. The cutting-edge technology and tools we provide help students create their own learning materials. StudySmarter’s content is not only expert-verified but also regularly updated to ensure accuracy and relevance.
Learn more