Jump to a key chapter
Understanding Aerodynamic Forces
Aerodynamic forces play a crucial role in determining how objects move through air. From the flight of an aeroplane to the design of a car, understanding these forces is key to engineering more efficient and effective designs.
What are Aerodynamic Forces?
Aerodynamic forces are those forces that act on an object as it moves through the air. These forces are primarily the result of differences in air pressure and can be classified into four main types: lift, drag, thrust, and weight.
Lift: A force that acts perpendicular to the direction of motion, typically helping an object to rise.
Drag: A force that opposes the direction of motion, causing resistance that slows down the object.
Thrust: A force that propels an object forward in direction, often generated by engines or propellers.
Weight: The force due to gravity, acting downwards, that must be overcome by lift for an object to rise.
Consider an aeroplane: It achieves lift through its wings, drag is encountered as air resistance against its body, thrust is provided by its engines, and it must overcome its weight to ascend.
Engineers carefully balance these forces to ensure safe and efficient flight.
The Role of Aerodynamic Forces in Flight
The interplay between lift, drag, thrust, and weight is fundamental to flight. To achieve lift-off, an aircraft must generate enough lift to overcome its weight, and enough thrust to overcome drag.
- Lift is generated by the shape of the aircraft's wings, which are designed to create lower air pressure on their top surface compared to the bottom, allowing the aircraft to rise into the air.
- Drag must be minimized to preserve fuel and improve speed. This is achieved through streamlined design and smooth surfaces.
- Thrust generated by the engines must exceed drag for the aircraft to accelerate forward.
- Weight affects how much lift is needed. Heavier aircraft require more lift and, consequently, more thrust to maintain flight.
One interesting aspect of aerodynamic forces is how they are utilised differently in various flight manoeuvres. For example, during takeoff, thrust must be maximised to overcome drag and weight quickly, ensuring a rapid ascent. Conversely, during landing, drag is increased deliberately, by deploying flaps or reverse thrust, to allow the aircraft to slow down safely.
Different flight phases—takeoff, cruising, and landing—require adjustments in the balance between these forces for optimal performance and safety.
Aerodynamic Forces Acting on an Aircraft
When it comes to understanding how aircraft navigate the skies so efficiently, the concept of aerodynamic forces comes front and centre. These forces, including lift, drag, and others, determine the aircraft's ability to fly, maintain altitude, and manoeuvre through the air.
Exploring Aerodynamic Lift Force
Aerodynamic lift force is the invisible hand that elevates an aircraft into the sky. It's a vital component of flight, making it possible for planes, from small Cessnas to large commercial jets, to leave the ground and soar. But what exactly is lift, and how is it generated?Lift occurs when air moving over the wing travels faster than the air beneath it, creating a pressure difference. This phenomenon is explained by Bernoulli's principle, which states that an increase in the speed of a fluid occurs simultaneously with a decrease in pressure.
Lift: A mechanical force generated by the motion of an aircraft through the air, created by the difference in air pressure above and beneath the wings.
Looking at an aircraft wing during flight highlights how air divides, moving faster over the top surface. This difference in speed results in lower pressure above the wing and higher pressure below, causing the aircraft to be pushed upwards.
Adjusting the wing shape and angle can significantly affect the lift force, which pilots do to control ascent and descent.
Unravelling Aerodynamic Drag Force
Equally important but opposite in effect to lift is the aerodynamic drag force. While lift seeks to raise the aircraft, drag aims to do the very opposite, slowing it down by opposing the aircraft's motion through the air.There are two main types of drag: parasitic and induced. Parasitic drag, which includes form drag, skin friction, and interference drag, increases with the aircraft's speed. Induced drag, on the other hand, is related to generating lift and occurs as the result of the creation of wingtip vortices.
Drag: The resistive force acting opposite to the direction of motion of an aircraft through the air, consisting of components like form drag, skin friction, and induced drag.
When cruising at high speeds, an aircraft encounters increased resistance from the air, demonstrating parasitic drag. During takeoff and landing, wingtip vortices are more prominent, illustrating induced drag.
Streamlined design and smooth surfaces are crucial for minimising parasitic drag, enhancing overall efficiency.
The Importance of Balance Between Lift and Drag
The dance between lift and drag defines not only the flight of an aircraft but its efficiency and safety. An optimal balance ensures that the aircraft can maintain altitude, speed, and direction effectively. Too much drag relative to lift can lead to increased fuel consumption and reduced range, whereas too much lift can result in an inability to maintain stable flight.Engineers and pilots work together to manage this balance through aircraft design, operational techniques, and in-flight adjustments. For instance, altering the angle of attack and utilising flaps and slats can modify lift and drag properties mid-flight to suit specific needs.
Understanding the balance between lift and drag is further complicated by factors such as air density, temperature, and speed. Air at higher altitudes is less dense, which affects both lift and drag – requiring adjustments in engine thrust and wing configuration to maintain desired flight paths. Moreover, the challenges of balancing these forces become increasingly significant in supersonic flight, where drag rises sharply and different aerodynamic principles come into play.
Aircraft wings are often swept back to reduce drag at high speeds, a design inspired by observations of birds and natural flight.
Aerodynamic Force Equation
The Aerodynamic Force Equation is pivotal in understanding how objects move through air. By quantifying the forces acting on an aerodynamic body, this equation enables engineers and designers to predict and enhance the performance of aircraft, vehicles, and other objects.
Breaking Down the Aerodynamic Force Equation
The aerodynamic force ( extit{F}) acting on an object can be determined by the equation extit{F} = extit{0.5} * extit{ ho} * extit{V}^2 * extit{A} * extit{Cf}, where:
- extit{ ho} (rho) is the air density,
- extit{V} is the velocity of the object relative to the air,
- extit{A} is the reference area (such as wing area), and
- extit{Cf} (Cf) is the coefficient of force, which varies depending on the type of aerodynamic force being calculated (e.g., lift coefficient or drag coefficient).
Aerodynamic Force Equation: A mathematical representation used to calculate the forces of lift, drag, and overall aerodynamic force on an object as it moves through the air. It combines air density, object velocity, reference area, and a specific force coefficient.
For instance, calculating the lift force ( extit{L}) on an airplane wing involves using the lift coefficient ( extit{Cl}) in place of the coefficient of force ( extit{Cf}). With known values for air density, velocity, and wing area, one can predict how much lift an aircraft wing generates at different speeds and angles of attack.
The coefficient of force ( extit{Cf}) is crucial in these calculations, as it reflects the efficiency of the object's shape in generating lift or reducing drag.
Calculation Examples of Aerodynamic Force Equation
To illustrate the practical use of the Aerodynamic Force Equation, let's consider a few calculation examples. These not only demonstrate the applicability of the equation but also how it influences design and operational decisions.Example 1: Calculating the lift force on an aircraft wing at cruising speed. Given a cruising speed ( extit{V}), average air density at cruising altitude ( extit{ ho}), wing area ( extit{A}), and lift coefficient ( extit{Cl}), one can determine the total lift force keeping the aircraft aloft.Example 2: Estimating the drag force on a car. Using the car's frontal area as the reference area ( extit{A}), its velocity ( extit{V}), the air density ( extit{ ho}), and the drag coefficient ( extit{Cd}), the equation can help calculate the resistive force impacting the car's fuel efficiency and maximum speed.
Understanding the nuances of the Aerodynamic Force Equation sheds light on how subtle changes in design or operating conditions can significantly impact performance. For example, increasing the wing area ( extit{A}) of an aircraft can increase the lift force, but it may also increase drag, requiring a careful balance. Similarly, the air density ( extit{ ho}) decreases with altitude, affecting both lift and drag forces and thus impacting decisions related to the optimal cruising altitude for aircraft.
Aerodynamic Force Coefficient
The aerodynamic force coefficient is a fundamental concept in the study and application of aerodynamics, playing a central role in the design and analysis of vehicles and structures that interact with fluid flows, such as air.
Decoding the Aerodynamic Force Coefficient
The aerodynamic force coefficient is a dimensionless number that quantifies the aerodynamic force on an object as a function of its shape, orientation, and flow conditions. It is crucial for comparing the aerodynamic properties of different objects under varying conditions. The general formula for aerodynamic force ( extit{F}) is given by extit{F = 0.5 * extit{ ho} * V^2 * A * Cf}, where:
- extit{ ho} (rho) is the air density,
- extit{V} is the velocity of the object relative to the air,
- extit{A} is the reference area, and
- extit{Cf} is the aerodynamic force coefficient, specific to either lift ( extit{Cl}) or drag ( extit{Cd}) forces.
Aerodynamic Force Coefficient: A dimensionless number that represents the aerodynamic force acting on an object in relation to its size, shape, and the flow conditions around it.
As an example, the drag coefficient ( extit{Cd}) of a car determines how easily it cuts through the air. A lower extit{Cd} means the vehicle encounters less aerodynamic drag, improving fuel efficiency.
Aerodynamic force coefficients are extensively tested in wind tunnels where real-flow conditions can be simulated.
Application of Aerodynamic Force Coefficient in Design
In engineering, the aerodynamic force coefficient is instrumental in designing vehicles and structures to achieve optimal performance and safety. It influences how designers shape cars, aircraft, bridges, and buildings to withstand wind forces or reduce resistance. For vehicles, a lower drag coefficient ( extit{Cd}) translates into higher speed and better fuel efficiency. Engineers use this coefficient to streamline vehicle bodies, reducing air resistance. In the case of aircraft, both the lift coefficient ( extit{Cl}) and drag coefficient are crucial for achieving sufficient lift and controllable flight speeds. In the construction of bridges and skyscrapers, understanding wind load effects, guided by the force coefficients, is essential for ensuring structural stability and safety.
One particularly interesting application is in the design of sports apparel and equipment, such as cycling helmets and swimsuits. These items are engineered with surfaces that mimic biological structures known for their aerodynamic properties, aiming to reduce drag and improve performance. Moreover, the exploration of aerodynamic force coefficients has paved the way for innovations like biomimicry in design, where principles learned from the study of birds, fish, and other animals are applied to reduce aerodynamic drag and enhance energy efficiency in vehicles and aircraft.
Aerodynamic Forces - Key takeaways
- Aerodynamic Forces Definition: Forces acting on an object as it moves through air, including lift, drag, thrust, and weight, resulting primarily from differences in air pressure.
- Aerodynamic Lift Force: Generated by the shape of the aircraft's wings, it acts perpendicular to the direction of motion and is created by differences in air pressure above and beneath the wings, enabling the aircraft to rise.
Aerodynamic Drag Force: Opposes the direction of motion and consists of parasitic and induced drag, caused by air resistance against an object's surface and the creation of wingtip vortices respectively. - Aerodynamic Force Equation: Expressed as F = 0.5 * ρ * V2 * A * Cf, where ρ is air density, V is velocity, A is the reference area, and Cf is the aerodynamic force coefficient.
- Aerodynamic Force Coefficient: A dimensionless value (either lift coefficient Cl or drag coefficient Cd) that quantifies aerodynamic forces on an object, reflecting the efficiency of its shape in generating lift or reducing drag.
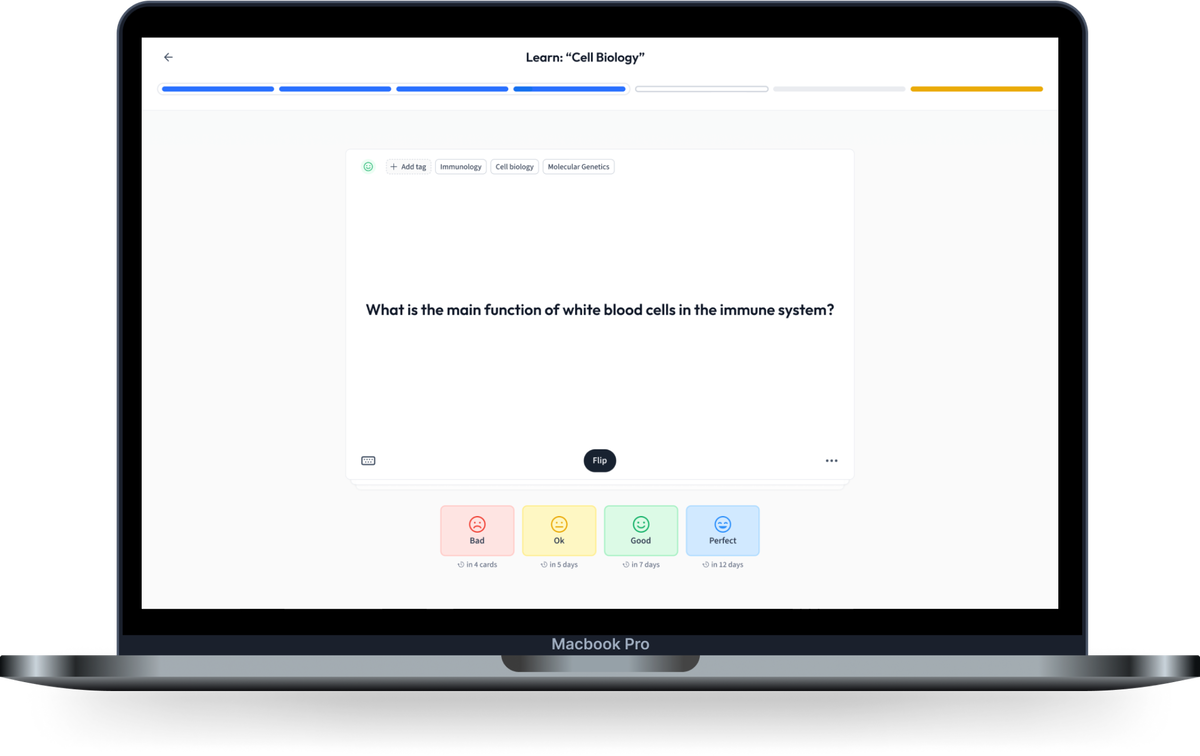
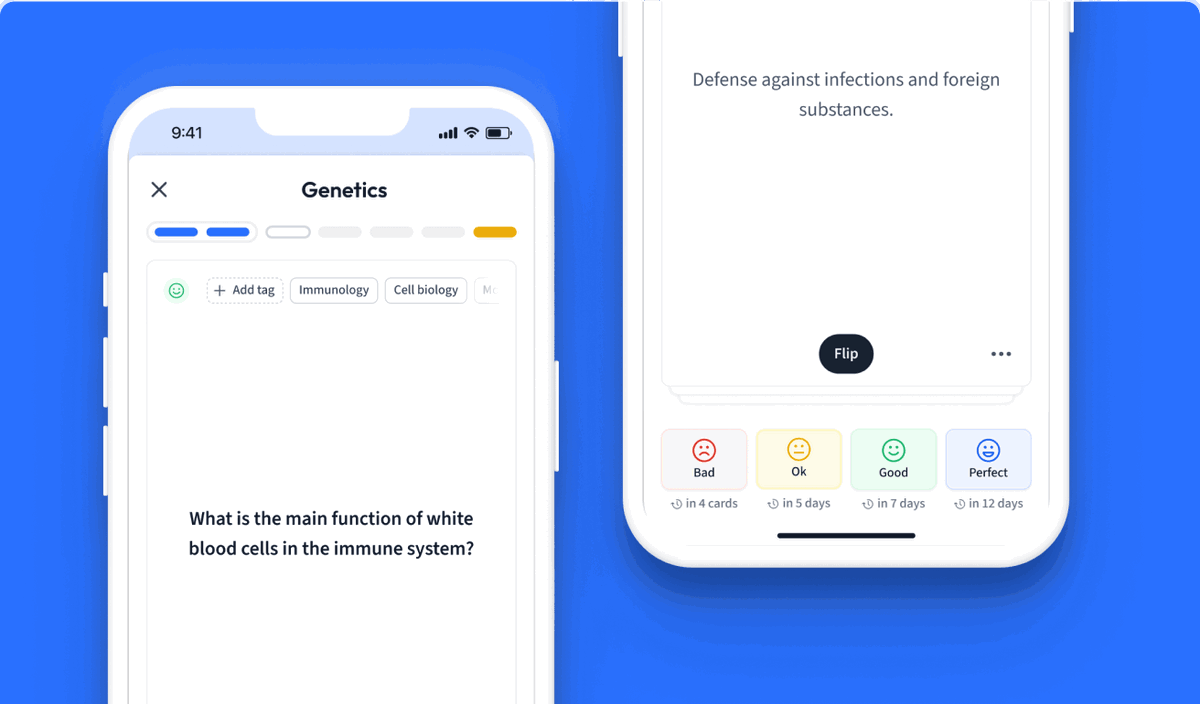
Learn with 12 Aerodynamic Forces flashcards in the free StudySmarter app
Already have an account? Log in
Frequently Asked Questions about Aerodynamic Forces
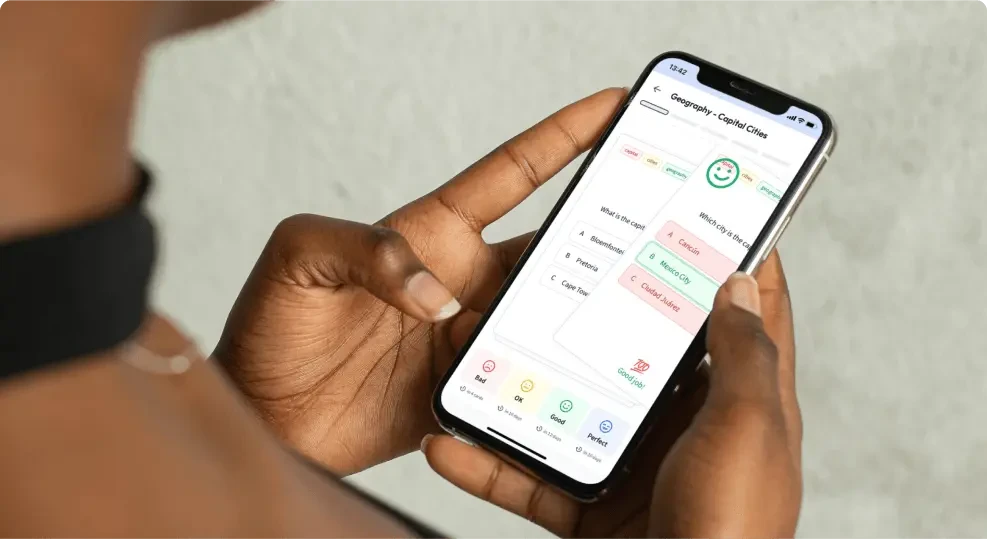
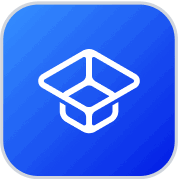
About StudySmarter
StudySmarter is a globally recognized educational technology company, offering a holistic learning platform designed for students of all ages and educational levels. Our platform provides learning support for a wide range of subjects, including STEM, Social Sciences, and Languages and also helps students to successfully master various tests and exams worldwide, such as GCSE, A Level, SAT, ACT, Abitur, and more. We offer an extensive library of learning materials, including interactive flashcards, comprehensive textbook solutions, and detailed explanations. The cutting-edge technology and tools we provide help students create their own learning materials. StudySmarter’s content is not only expert-verified but also regularly updated to ensure accuracy and relevance.
Learn more