Jump to a key chapter
What is Aeroelasticity Testing?
Aeroelasticity testing is a critical component of aerospace engineering, focusing on understanding and preventing potential issues arising from the interaction between aerodynamic forces, structural elasticity, and inertial forces. Such testing is essential to ensure the safety, performance, and durability of aircraft and spacecraft.
Understanding Aeroelasticity Testing Definition
Aeroelasticity Testing: The process of evaluating how airborne vehicles or structures respond to aerodynamic forces, elastic counter forces, and inertial loads to predict and mitigate potential aerodynamic instabilities.
This form of testing incorporates a range of simulations and physical experiments designed to identify and analyse phenomena such as flutter, buffeting, and divergence, which can compromise the integrity and control of an aircraft. By understanding these interactions, engineers can design safer and more efficient aircraft that can withstand the unpredictable nature of flying.
Example: Wind tunnel tests are a common practice within aeroelasticity testing. These tests allow engineers to observe the behaviour of scaled models of aircraft components under varying wind conditions and speeds, providing valuable data to predict full-scale performance.
The Importance of Aeroelasticity Testing in Aerospace Engineering
Aeroelasticity testing plays a pivotal role in aerospace engineering for several reasons:
Modern aerospace engineering relies heavily on computational fluid dynamics (CFD) and finite element analysis (FEA) along with physical tests to conduct aeroelasticity testing.
Aeroelasticity Flutter Testing Techniques
Aeroelasticity Flutter Testing Techniques encompass a range of experimental and computational methods to assess and mitigate flutter—a potentially destructive oscillation caused by the interaction between aerodynamic forces, structural dynamics, and control system responses. These techniques are vital for ensuring the safety and reliability of aircraft and other aerodynamic structures.
Basic Principles of Aeroelasticity Flutter Testing
The foundation of aeroelasticity flutter testing lies in understanding the interaction between aerodynamic forces, structural response, and inertial effects. This interaction can give rise to flutter, a dynamic instability that can lead to catastrophic failure if not adequately addressed. At its core, flutter testing aims to identify the conditions under which flutter occurs and to validate the effectiveness of design modifications to mitigate these risks.Key components of this process include determining the flutter margin, which is the safety buffer between operational speeds and the onset of flutter, and assessing the damping characteristics of the structure. Both of these factors are critical for ensuring that an aircraft remains safe and flutter-free throughout its operational envelope.
Flutter Margin: The difference between the airspeed at which flutter occurs and the maximum operational airspeed of the aircraft, providing a safety buffer to prevent flutter during normal operations.
Techniques used in basic flutter testing range from ground vibration testing, where the structure is excited in a controlled environment to identify its natural frequencies, to wind tunnel testing and in-flight experiments, where actual aerodynamic conditions are simulated. These tests are complemented by computational analyses using numerical methods such as Computational Fluid Dynamics (CFD) and Finite Element Analysis (FEA) to predict flutter behaviour without the need for extensive physical testing.
Example: A wind tunnel test for flutter might involve mounting a model of an aircraft wing on a flexible support inside the wind tunnel. The model is subjected to airflow at increasing speeds while sensors record the wing's response, looking for signs of flutter or other aeroelastic phenomena. This provides valuable data for designing flutter-resistant wings.
Advances in Aeroelasticity Flutter Testing Methods
With technological advancements, new methods in aeroelasticity flutter testing have emerged, significantly enhancing the ability to predict and mitigate flutter. These include the use of advanced computational models that can simulate complex fluid-structure interactions more accurately and at a fraction of the cost of traditional testing methods.Additionally, the integration of real-time data acquisition systems in flutter testing allows for the continuous monitoring of structural responses during flight tests. This real-time feedback enables immediate adjustments and vastly improves the efficiency of the flutter mitigation process, potentially saving both time and resources.
Recent Innovations: Ground-breaking techniques such as active flutter suppression systems and adaptive materials offer promising avenues for aeroelastic control. Active flutter suppression involves the use of control surfaces or other mechanisms to counteract the aerodynamic forces that induce flutter. Meanwhile, adaptive materials can change their stiffness in response to aerodynamic conditions, thereby altering the structure's natural frequency to avoid flutter.Potential Impact: These advances could redefine aircraft design principles, leading to safer, more efficient, and more resilient aircraft capable of operating in a wider range of conditions.
Dynamic Aeroelastic Testing Procedure
The dynamic aeroelastic testing procedure is a systematic approach used in aerospace engineering to study the interactions between aerodynamic forces, structural elasticity, and inertial effects on aircraft structures. These procedures are essential for ensuring the safety and efficiency of aircraft designs.Through various tests, including wind tunnel testing, ground vibration tests, and flight tests, engineers can identify and mitigate potential aeroelastic issues such as flutter, divergence, and control reversal, which could otherwise compromise aircraft performance and safety.
Steps Involved in Dynamic Aeroelastic Testing
Dynamic aeroelastic testing is a multifaceted process, encompassing a series of critical steps designed to thoroughly evaluate and ensure aircraft integrity against aeroelastic phenomena:
- Identification of Aeroelastic Phenomena: The first step involves identifying potential aeroelastic risks such as flutter or divergence that could affect the aircraft during its operational life.
- Development of Mathematical Models: Mathematical models are developed to simulate the behaviour of the aircraft under various aerodynamic loads.
- Execution of Ground Tests: Ground-based tests, such as ground vibration tests (GVT), are conducted to gather data on the structural dynamics of the aircraft.
- Wind Tunnel Testing: Models of the aircraft are subjected to controlled aerodynamic environments in a wind tunnel to study their aeroelastic behaviour.
- Flight Testing: Flight tests are conducted to observe and record the aircraft's aeroelastic characteristics in actual flight conditions.
- Data Analysis and Mitigation Strategies: The final step involves analysing the collected data to identify any aeroelastic issues and developing mitigation strategies to address them.
Aeroelasticity Testing Mathematical Models
Mathematical models are indispensable tools in the field of aeroelasticity testing, providing a critical link between theoretical concepts and real-world phenomena. These models help engineers to predict how aircraft structures will respond to various aerodynamic forces, allowing for the design of safer, more reliable aircraft.To develop these models, engineers utilise principles from fluid dynamics and structural mechanics, often employing sophisticated computational tools such as Computational Fluid Dynamics (CFD) and Finite Element Analysis (FEA).
Flutter Speed Equation: One fundamental formula used in the analysis of aeroelastic flutter is the flutter speed equation, given by: \[ V_f = rac{2 heta}{ ho S B^2} imes rac{K_g + K_a}{K_e} imes rac{T}{L} imes 4 heta^2 imes rac{1}{ ho S B^2} imes rac{K_g}{K_e} imes rac{T}{L} imes (1-rac{F_{aero}}{F_{inert}}) imes rac{C_l imes ext{chord}}{B} imes rac{D}{4} imes rac{C_d}{C_l^2} imes rac{A}{B} imes rac{ ho S}{M} imes (1 + rac{E}{G}) imes rac{I}{J} imes 2 heta imes rac{D}{C_d} imes rac{M}{ ho S B^2} imes V_f^2 imes rac{C_l}{C_d} imes rac{ ext{chord}}{B} imes rac{D}{4} imes rac{A}{B} imes V_f^2 imes rac{1}{M} imes V_f imes (1 + E imes rac{I}{J}) imes 2 heta imes rac{C_l}{C_d} imes rac{M}{ ho S B^2} imes V_f ext{, where} heta, ho, S, B, K_g, K_a, K_e, T, L, F_{aero}, F_{inert}, C_l, C_d, chord, D, A, M, E, G, I, ext{and} J ext{represent various physical parameters.}
These models encompass various factors, including the structural properties of the aircraft, the fluid dynamics of air moving over the aircraft surfaces, and the interaction between these two domains. The complexity of these models can vary significantly, from simple analytical models that provide a basic understanding of aeroelastic phenomena to highly complex numerical simulations that can accurately predict aeroelastic responses in a wide range of flight conditions.Ultimately, the goal of developing these mathematical models is to ensure that aircraft are designed with sufficient margins of safety to prevent aeroelastic instabilities such as flutter, which can lead to structural failure and potentially catastrophic outcomes.
Example: In wind tunnel testing, engineers may use a simplified mathematical model to predict the onset of flutter at different wind speeds. This model helps in designing the experiment and interpreting the results, allowing for adjustments to the aircraft design to increase its flutter margin.
Wing and Rotor Aeroelastic Test Systems
Wing and rotor aeroelastic test systems are essential components in the aerospace industry, designed to simulate and study the effects of aerodynamic forces on aircraft wings and rotor blades. These systems help ensure that designs are safe, effective, and efficient before full-scale production and deployment.
Designing Aeroelastic Test Systems for Wings and Rotors
Developing aeroelastic test systems for wings and rotors involves several critical considerations to replicate real-world conditions as accurately as possible. Engineers must factor in the specific aerodynamic forces acting on wings and rotors, the structural materials' properties, and how these elements interact under various flight conditions.The design process begins with understanding the specific requirements of the wing or rotor, including size, material composition, and expected operational conditions. This foundation allows engineers to create test systems that provide valuable data on the aeroelastic behaviours of these components, such as flutter, buffeting, and other dynamic responses to aerodynamic loads.
Key elements in designing these test systems include:
- Replicating realistic aerodynamic environments through advanced simulation techniques or wind tunnel tests.
- Choosing materials and structural configurations that accurately reflect the wing or rotor's design and operational stresses.
- Incorporating precise measurement and data acquisition tools to capture dynamic responses to aerodynamic forces.
- Ensuring that the system can simulate a wide range of operating conditions to identify potential aeroelastic issues under diverse scenarios.
Aeroelasticity Testing Principles Explained
The fundamental principles of aeroelasticity testing involve understanding and observing how wings, rotors, and other aeroelastic structures respond to aerodynamic forces. These tests simulate operational stress and environmental conditions, revealing potential design vulnerabilities and enabling corrections before actual flight.A critical aspect of aeroelasticity testing is the study of how different materials and structures deflect, vibrate, or undergo deformation under aerodynamic loads. This understanding is vital in preventing aeroelastic phenomena like flutter, a dynamic instability that can lead to structural failure.
Flutter: A dangerous aeroelastic phenomenon where aerodynamic forces cause a structure to oscillate uncontrollably. It is critical to identify and mitigate any tendency towards flutter in the design of wings and rotors.
Example: During a wing aeroelastic test, engineers might simulate flight conditions that cause the wing to enter a flutter mode. Observing this reaction allows for adjustments in wing geometry, material choices, or damping mechanisms to stabilise the wing and prevent flutter in actual flight conditions.
These principles extend to rotorcraft as well, where rotor blades face complex aerodynamic interactions. The dynamic nature of rotor operation — transitioning between various flight regimes such as hover, forward flight, and autorotation — demands sophisticated testing systems designed to accurately capture the unique aeroelastic characteristics of rotors.Ultimately, the goal of aeroelasticity testing is to ensure that wings and rotors can withstand dynamic aerodynamic forces without compromising safety or performance. This is achieved through rigorous testing and analysis, leading to designs that are both robust and efficient.
Advances in computational power and methods have significantly enhanced the scope and accuracy of aeroelasticity testing, allowing for more complex and realistic simulations of aerodynamic forces and their effects on wings and rotors.
Aeroelasticity Testing - Key takeaways
- Aeroelasticity Testing Definition: The process of evaluating how airborne vehicles or structures respond to aerodynamic forces, elastic counter forces, and inertial loads to predict and mitigate potential aerodynamic instabilities such as flutter.
- Aeroelasticity Flutter Testing Techniques: Experimental and computational methods to assess and mitigate flutter, which involves determining the flutter margin and assessing damping characteristics of the structure.
- Dynamic Aeroelastic Testing Procedure: A systematic approach encompassing identification of aeroelastic phenomena, development of mathematical models, execution of various tests (e.g., ground vibration tests, wind tunnel testing), and analysis and development of mitigation strategies.
- Aeroelasticity Testing Mathematical Models: Utilise principles from fluid dynamics and structural mechanics to simulate aircraft response to aerodynamic forces, featuring complex equations such as the flutter speed equation to predict aeroelastic phenomena.
- Wing and Rotor Aeroelastic Test Systems: Test systems designed to simulate and study the effects of aerodynamic forces on aircraft wings and rotor blades, which involve replicating real-world conditions and capturing dynamic responses to identify aeroelastic issues.
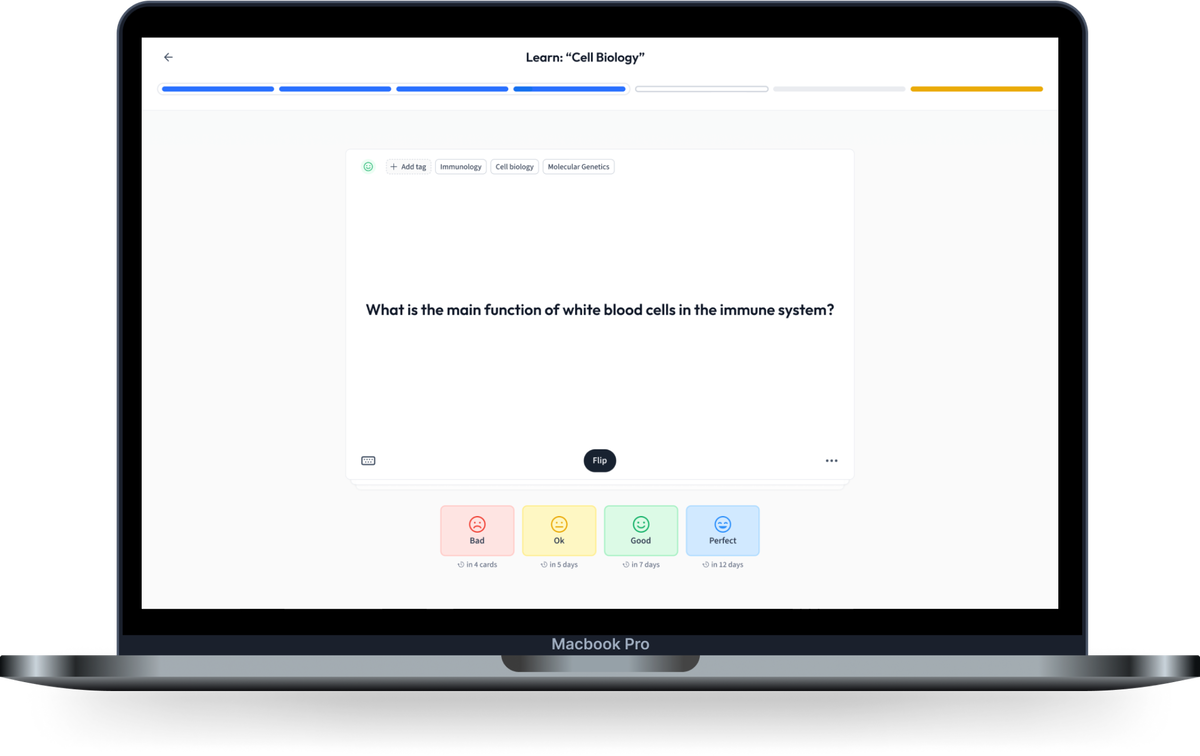
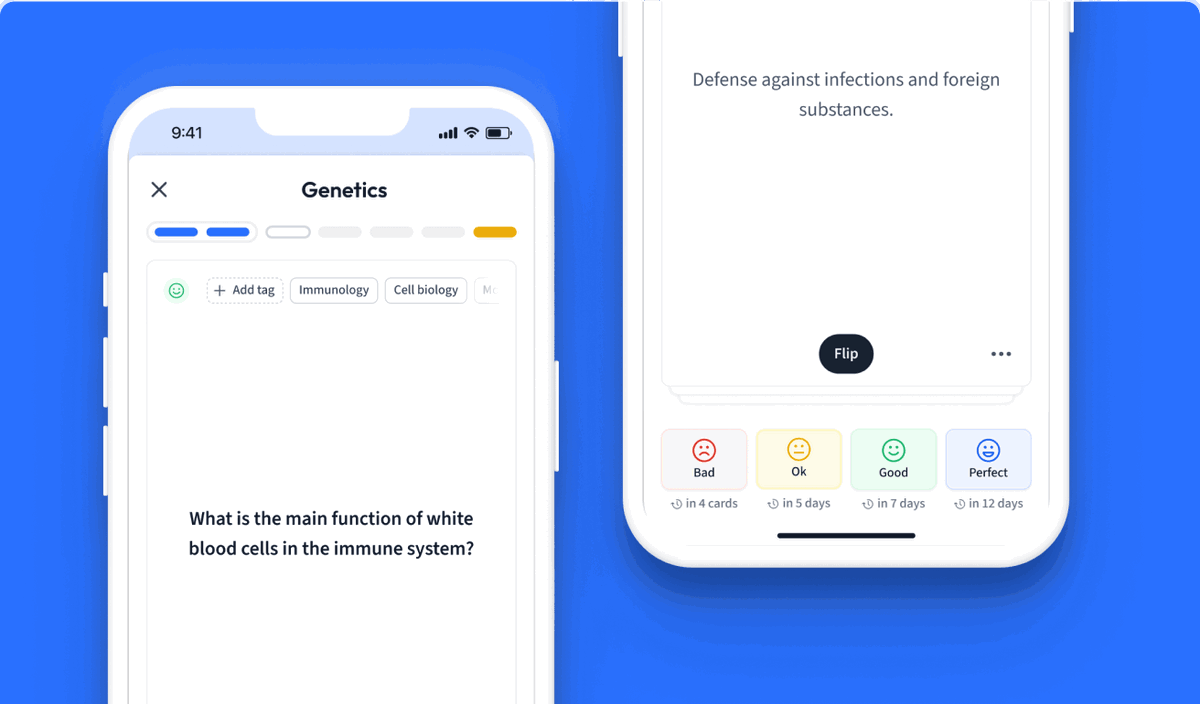
Learn with 12 Aeroelasticity Testing flashcards in the free StudySmarter app
Already have an account? Log in
Frequently Asked Questions about Aeroelasticity Testing
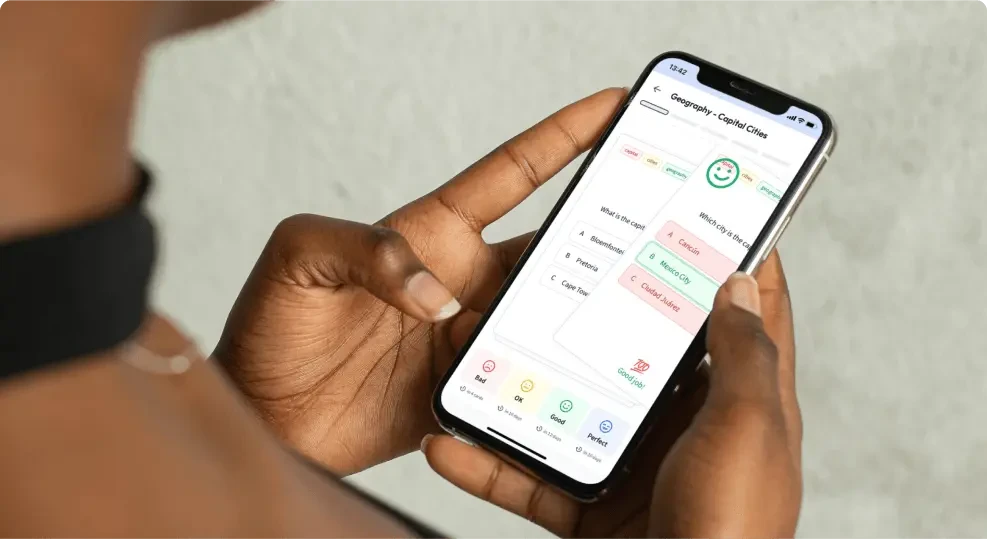
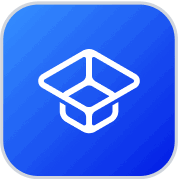
About StudySmarter
StudySmarter is a globally recognized educational technology company, offering a holistic learning platform designed for students of all ages and educational levels. Our platform provides learning support for a wide range of subjects, including STEM, Social Sciences, and Languages and also helps students to successfully master various tests and exams worldwide, such as GCSE, A Level, SAT, ACT, Abitur, and more. We offer an extensive library of learning materials, including interactive flashcards, comprehensive textbook solutions, and detailed explanations. The cutting-edge technology and tools we provide help students create their own learning materials. StudySmarter’s content is not only expert-verified but also regularly updated to ensure accuracy and relevance.
Learn more