Jump to a key chapter
Overview of Aerospace Composite Structures
The exploration and utilisation of aerospace composite structures have significantly transformed the aerospace industry. These advanced materials are designed to meet the unique demands of aerospace engineering, offering unprecedented strength, durability, and weight efficiency. Aerospace composite structures play a pivotal role in the development and operation of modern aircraft and spacecraft, marking a revolutionary step forward from traditional materials like aluminium and steel.
What are Composite Materials and Structures in Aerospace Engineering?
Composite materials in aerospace engineering refer to a combination of two or more distinct materials to create a new material with enhanced properties. These composites typically consist of a fibrous material, such as carbon or glass fibres, embedded in a resin matrix. The resulting structure offers a superior strength-to-weight ratio, corrosion resistance, and durability compared to traditional aerospace materials.
Aerospace Composite Structures: Engineered materials made by combining fibrous reinforcements (e.g., carbon, glass fibres) with a matrix (e.g., epoxy resin) to produce materials that are specifically designed for aerospace applications, offering high strength, lightweight, and resistance to environmental impacts.
An example of an aerospace composite structure is the carbon fibre reinforced polymer (CFRP) used in the manufacturing of aircraft wings. These wings benefit from the high strength and low weight of CFRP, leading to more fuel-efficient aircraft.
The unique properties of aerospace composites, such as their ability to withstand significant stress while remaining lightweight, have made them indispensable in the construction of high-performance aerospace vehicles.
Importance of Composite Structures Aerospace Applications
Composite structures have become central to aerospace applications due to their significant advantages over traditional materials. The application of these materials spans across various components of aerospace vehicles, including fuselage, wings, propellers, and even interior elements. Composite structures contribute to aerospace design and functionality by offering:
- Reduced weight, leading to improved fuel efficiency and payload capacity.
- Enhanced strength and stiffness, allowing for the design of safer and more resilient aerospace vehicles.
- Corrosion resistance, resulting in lower maintenance costs and longer service life.
- Greater design flexibility, enabling more aerodynamic shapes and innovative configurations.
The evolution of aerospace composite structures has been driven by continual advancements in materials science and manufacturing techniques. From the early use of fibreglass in secondary structures to the sophisticated carbon and glass fibre composites used today, aerospace engineering has consistently pushed the boundaries of what is possible. Modern manufacturing methods, such as automated fibre placement and resin transfer moulding, have significantly improved the quality and consistency of composite components, further enhancing their performance and reliability in aerospace applications.
Design and Analysis of Composite Structures with Applications to Aerospace
Understanding the design and analysis of composite structures is crucial in the realm of aerospace engineering. As you delve into this topic, you'll discover how these advanced materials have revolutionised the way aircraft and spacecraft are built, offering significant advantages in terms of strength, weight, and durability over traditional materials.
Key Factors in Designing Advanced Aerospace Composite Structures
The design of advanced aerospace composite structures requires careful consideration of several key factors to ensure they meet the rigorous demands of aerospace applications. These factors include material selection, structural configuration, manufacturing techniques, and performance under operating conditions. By understanding and optimising these elements, engineers can create composite structures that effectively contribute to the innovation and efficiency of aerospace vehicles.
Composite Structure Design Factors: Critical considerations in the creation of composite materials for aerospace applications, including material types, structural designs, manufacturing processes, and performance criteria.
For instance, when designing aircraft wings using composite materials, engineers must ensure that the chosen material can withstand the aerodynamic loads and stresses encountered during flight. This requires selecting the appropriate fibre and matrix materials, determining the optimal fibre orientation, and employing manufacturing techniques that ensure the integrity and strength of the composite structure.
The selection of the matrix and reinforcement materials largely depends on the specific requirements of the aerospace application, including temperature resistance, structural rigidity, and weight constraints.
The evolution of composite materials within aerospace engineering underscores the sector's ongoing quest for improvement. Innovations in material science and engineering have enabled the development of composites that can endure higher temperatures and exhibit greater strength and toughness. This progress has been pivotal in advancing the performance and efficiency of aerospace structures, pushing the boundaries of what is possible in aerospace engineering.
Tools and Techniques for Analysing Composite Structures
Analyzing aerospace composite structures involves a suite of sophisticated tools and techniques. These methods enable engineers to predict how composites will behave under various conditions, ensuring they meet safety and performance standards. Computational tools, such as finite element analysis (FEA), along with physical testing methods, play an essential role in the evaluation of composite materials in aerospace engineering.
A common tool used in the analysis of aerospace composite structures is Finite Element Analysis (FEA). This computational technique models how composite materials deform, break, or fail under different types of loads. For example, FEA can simulate the impact of aerodynamic forces on an aircraft's composite wing, helping engineers to identify potential points of failure and optimise the design for greater efficiency and safety.
In addition to computational analysis, physical testing of composite materials under simulated environmental conditions is crucial for validating their performance in real-world aerospace applications.
Beyond FEA, other techniques such as digital image correlation (DIC) and acoustic emission (AE) monitoring further enrich the toolset available for the analysis of composite structures. DIC offers a powerful way to visualise the strain distribution over the surface of a composite structure under load, while AE monitoring can detect the sound of fibres breaking within the composite, offering early warning signs of potential failure. Together, these tools enable a comprehensive understanding of the behaviour of composite materials under a wide array of conditions, leading to safer, more reliable aerospace structures.
Advanced Composites and Aerospace Structural Systems
Advanced composites have become a cornerstone in the development of aerospace structural systems, offering unparalleled strength-to-weight ratios, reduced maintenance needs, and improved fuel efficiency. These materials, including carbon fibre and glass fibre composites, are defining the future of aerospace design and manufacturing.
Innovations in Advanced Aerospace Composite Structures
Innovations in aerospace composite structures have been pivotal in pushing the boundaries of what's achievable in aerospace design and functionality. From lighter, more efficient commercial aircraft to robust spacecraft able to withstand the rigours of space travel, the advancements in composite technology are continually shaping the industry.
- Use of Nano-enhanced composites for increased strength and temperature resistance.
- Development of bio-based composites aiming for sustainability in aerospace materials.
- Advancements in automated fibre placement (AFP) technologies, allowing for more complex and precise composite structures.
An example of innovation in this field is the Boeing 787 Dreamliner, which utilises advanced composite materials for approximately 50% of its primary structure, including the fuselage and wing. This significant use of composites is a testament to their importance in modern aerospace engineering, offering reduced weight and enhanced fuel efficiency.
The Airbus A350 XWB is another example where over 50% of its structure is made up of composite materials, underscoring the vital role of composites in the next generation of aircraft design.
While the use of composites in aerospace is not new, the continuous innovation in composite material science has led to the development of next-generation composites like Carbon Nanotube-reinforced polymers (CNTRP). These materials boast superior mechanical properties, including increased strength and resistance to impacts, while maintaining the lightweight characteristics vital for aerospace applications. The evolution of composite materials is closely tied to advancements in manufacturing processes, such as 3D printing of composites, opening new possibilities for complex, lightweight aerospace structures with reduced production times and costs.
Aerospace Structural Systems and Their Evolution
The evolution of aerospace structural systems has been marked by significant milestones driven by advancements in materials science, engineering practices, and manufacturing technologies. Starting from metal-based structures to the now prevalent composite-based frameworks, aerospace structural systems have become more efficient, reliable, and versatile.
Generation | Material | Advantages |
1st | Metals (Aluminium, Titanium) | Durable, widely available |
2nd | Fibreglass composites | Lightweight, corrosion resistant |
3rd | Carbon fibre composites | Superior strength-to-weight, fatigue resistance |
4th (Emerging) | Nano-enhanced composites | Ultra-high strength, thermal stability |
Carbon Fibre Reinforced Polymers (CFRP) are advanced composite materials used extensively in aerospace structural systems. Composed of carbon fibres and a polymer matrix, CFRP offers exceptional strength and stiffness, low weight, and resistance to fatigue and corrosion, making it ideal for critical aerospace applications such as the fuselage, wings, and tails of modern aircraft.
The use of CFRP in the Airbus A350 XWB not only reduces the aircraft's overall weight but also lowers its maintenance requirements compared to traditional metal structures. This example illustrates the transformative impact of advanced composites on the efficiency and sustainability of aerospace vehicles.
The shift towards more sustainable materials in aerospace engineering, such as bio-based composites, reflects the industry's commitment to reducing its environmental footprint while enhancing performance and cost-effectiveness.
Structural Health Monitoring of Aerospace Composites
Structural Health Monitoring (SHM) plays a crucial role in ensuring the safety, reliability, and longevity of aerospace composite structures. By integrating sensors and systems designed to detect damage or changes in material properties, SHM systems enable real-time monitoring and early detection of issues, potentially reducing maintenance costs and increasing aircraft availability.
Techniques for Monitoring the Health of Aerospace Composite Structures
Several techniques have been developed for monitoring the health of aerospace composite structures. These techniques vary in complexity, cost, and detection capabilities, making each suitable for different applications within the aerospace industry.
- Acoustic Emission (AE): Detects the sound waves produced by the growth of cracks or disbands within the material.
- Ultrasonic Testing (UT): Utilises high-frequency sound waves to detect internal flaws or defects.
- Digital Image Correlation (DIC): A non-contact method that captures sequence images to measure deformation and strain.
- Fibre Optic Sensors: Embedded within the composite material to provide data on strain, temperature, and pressure changes.
- Electrical Impedance Tomography (EIT): Monitors the electrical conductivity or permittivity of the composite material to identify damage.
Structural Health Monitoring (SHM): An integrated system that utilises various sensing and data processing techniques to continuously monitor the condition of a structure, detecting and assessing damage to ensure its operational integrity and safety.
Incorporating fibre optic sensors into the wings of an aircraft allows for the detection of minor strains that could indicate the onset of structural failure. These sensors, by measuring subtle changes in light transmission caused by deformation, provide critical data without adding significant weight or complexity to the aircraft structure.
The choice of a particular SHM technique or a combination of techniques depends largely on the specific requirements of the aerospace structure being monitored, including the types of materials used, the expected types of damage, and the criticality of the component to overall flight safety.
Case Studies: Successful Health Monitoring of Aerospace Structures
There are numerous instances where the application of SHM techniques has significantly contributed to the safety and efficiency of aerospace operations. A few notable case studies illustrate the successful implementation of SHM in real-world situations.
- The Boeing 787 Dreamliner: Extensively uses carbon fibre reinforced polymer (CFRP) composites and incorporates advanced SHM techniques to monitor the integrity of its fuselage and wings, enhancing safety and reducing maintenance downtime.
- Airbus A380: Employs a variety of SHM systems, including acoustic emission sensors, to detect damage in its composite components, ensuring operational integrity throughout the aircraft's service life.
- F-35 Lightning II: Features an integrated health monitoring system that utilises both fibre optic sensors and ultrasonic testing to assess the condition of its composite structures, facilitating a proactive approach to maintenance and repairs.
The successful implementation of SHM systems in aircraft like the Boeing 787 Dreamliner and the Airbus A380 demonstrates the significant advantages of integrating these technologies into aerospace composite structures. By enabling early detection of potential failures, SHM systems not only enhance safety but also improve the economic efficiency of aircraft operation by reducing unplanned downtime and extending the service life of critical components. As aerospace technology continues to evolve, the development of more sophisticated SHM techniques will undoubtedly play a key role in future advancements, potentially incorporating artificial intelligence and machine learning to further improve the accuracy and efficiency of structural health assessments.
Aerospace Composite Structures - Key takeaways
- Aerospace Composite Structures: Engineered materials made for aerospace applications, combining high strength, lightweight, and resistance to environmental impacts.
- Composite Materials in Aerospace Engineering: Materials consisting of fibrous material embedded in a resin matrix, offering superior strength-to-weight ratio and corrosion resistance.
- Design and Analysis of Composite Structures: Key considerations include material selection, structural configuration, and manufacturing techniques to meet aerospace demands.
- Structural Health Monitoring (SHM): Integrated systems using sensors and data processing for real-time monitoring and early damage detection in aerospace composites.
- Finite Element Analysis (FEA): A computational tool for modelling how aerospace composite structures behave under various loads, crucial for design optimisation and safety.
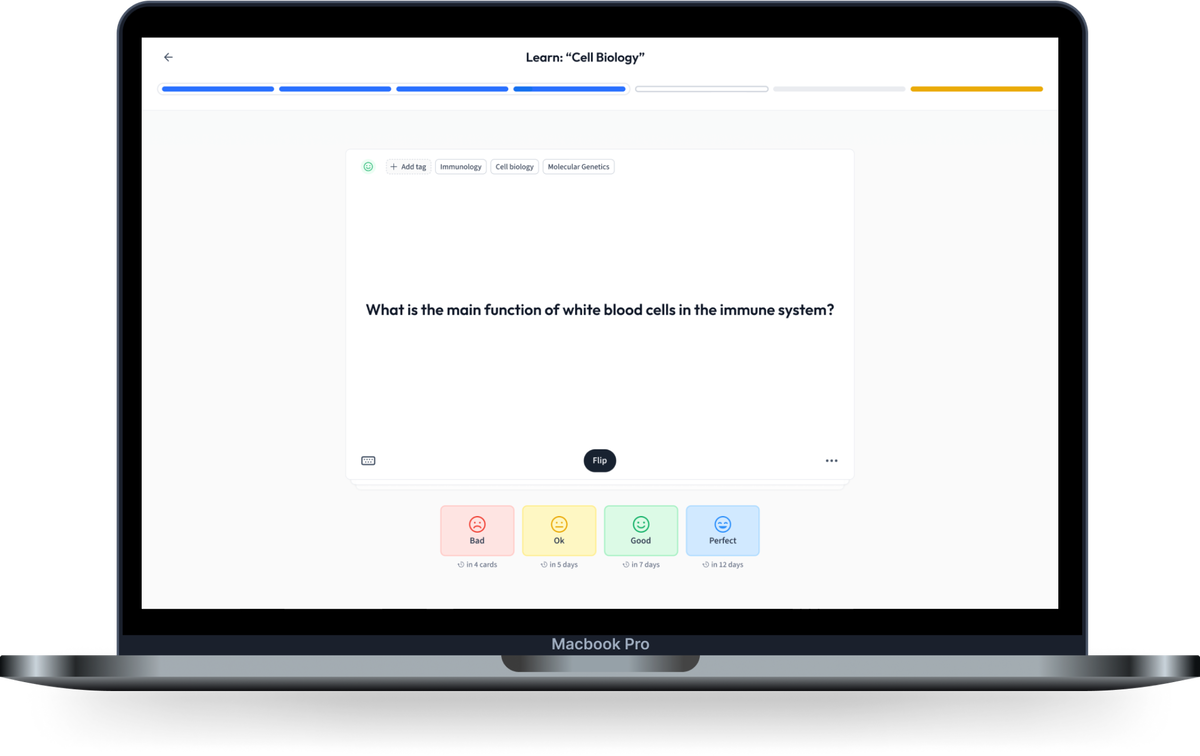
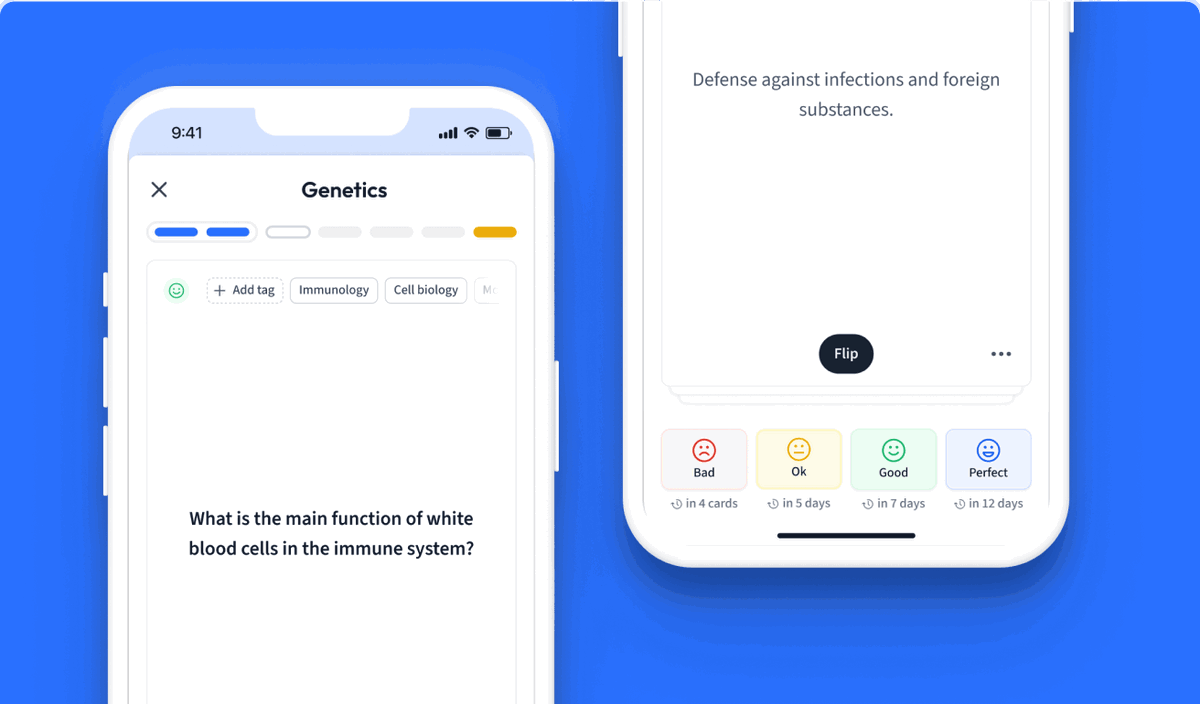
Learn with 12 Aerospace Composite Structures flashcards in the free StudySmarter app
Already have an account? Log in
Frequently Asked Questions about Aerospace Composite Structures
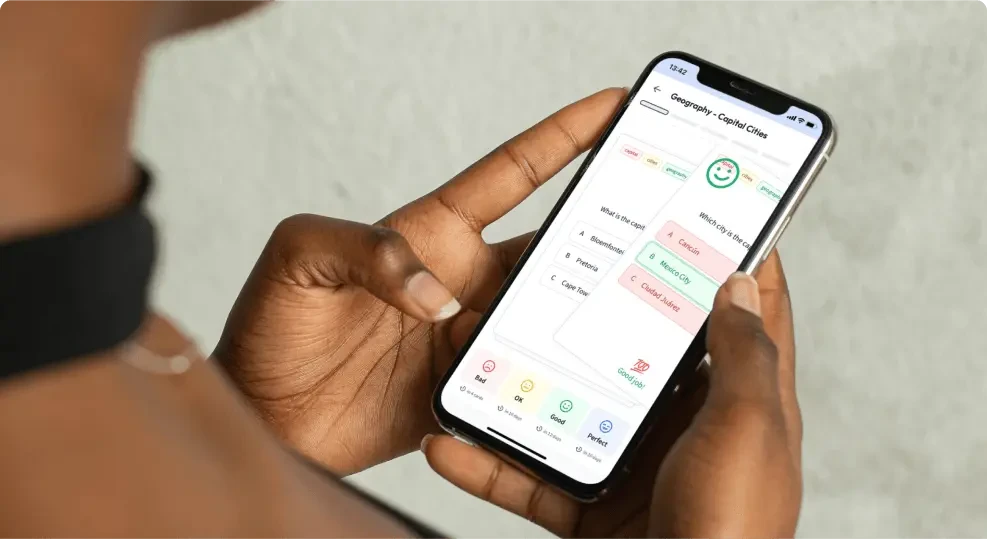
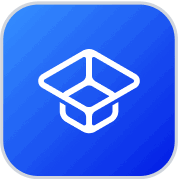
About StudySmarter
StudySmarter is a globally recognized educational technology company, offering a holistic learning platform designed for students of all ages and educational levels. Our platform provides learning support for a wide range of subjects, including STEM, Social Sciences, and Languages and also helps students to successfully master various tests and exams worldwide, such as GCSE, A Level, SAT, ACT, Abitur, and more. We offer an extensive library of learning materials, including interactive flashcards, comprehensive textbook solutions, and detailed explanations. The cutting-edge technology and tools we provide help students create their own learning materials. StudySmarter’s content is not only expert-verified but also regularly updated to ensure accuracy and relevance.
Learn more