Jump to a key chapter
Introduction to Aerospace Control Applications
Aerospace Control Applications encompass a wide range of technologies designed to manage and ensure the safety, stability, and efficiency of aircraft and spacecraft operations. From commercial airlines to space exploration missions, these applications play a crucial role in modern aerospace engineering, offering solutions for navigation, communication, and system management.
Understanding the Basics of Aerospace Control Systems
At the core of Aerospace Control Applications are control systems, which are engineered to monitor and adjust the behaviour of aerospace vehicles. These systems use a mixture of sensors, actuators, and algorithms to ensure that an aircraft or spacecraft follows its intended path, maintains its altitude, and remains stable during flight.
Aerospace Control System: An integrated system comprising sensors, actuators, and control algorithms designed to manage the behavior of aerospace vehicles to achieve desired performance outcomes.
For instance, an autopilot system in a commercial aircraft can be seen as a type of aerospace control application. It uses sensor data to adjust flight controls automatically, helping to maintain a steady course and altitude without constant input from the pilots.
These control systems can operate in manual, semi-automatic, or fully automatic modes, depending on the level of human intervention required.
- Sensors measure physical properties like speed, altitude, and orientation.
- Actuators execute commands from the control systems, adjusting parts like the rudder, ailerons, and engines.
- Control Algorithms process sensor inputs to make decisions about required adjustments to the aircraft or spacecraft's behavior.
The Role of Aerospace Control Applications in Modern Engineering
Aerospace Control Applications significantly influence the efficiency and safety of aerospace operations, impacting areas from commercial aviation to space exploration. These applications not only address the challenges of navigating through the Earth's atmosphere and space but also ensure that these missions can be performed with a high degree of precision and safety.
The advancements in aerospace control technologies have facilitated remarkable achievements, such as landing on the moon, sending unmanned probes to distant planets, and developing commercial aircraft that can fly longer distances with greater fuel efficiency and safety. These successes underscore the essential nature of control applications in pushing the boundaries of what is possible in aerospace engineering.
One of the most iconic examples of aerospace control application success is the Apollo Lunar Module, which safely landed astronauts on the moon. This module utilised a sophisticated control system that included guidance, navigation, and control (GNC) technologies to perform one of history's most challenging landings. The GNC system was responsible for executing complex maneuvers to descend from lunar orbit to the moon's surface, demonstrating the critical role of control systems in aerospace achievements.
Modern spacecraft use advanced forms of aerospace control applications, leveraging artificial intelligence and machine learning algorithms for more autonomous operations.
Optimal Control Theory with Aerospace Applications
The realm of Aerospace Engineering has seen transformative advancements through the integration of Optimal Control Theory. This mathematical framework, aimed at optimizing the performance of dynamic systems under given constraints, finds pivotal applications in aerospace to enhance vehicle efficiency, safety, and mission success.
Exploring Optimal Control Theory in Aerospace
Optimal Control Theory offers a systematic approach to determining the control strategy that yields the best performance according to a specific criterion, often involving the minimization or maximization of a cost function. It involves complex mathematical tools to model and solve control problems by considering future states in decision-making processes.
The application of Optimal Control Theory in aerospace ranges from flight trajectory optimization to fuel consumption and time efficiency, providing a vital toolbox for engineers to address challenges in vehicle guidance and control.
Optimal Control Theory: A mathematical framework for determining the control strategy that maximises or minimises a certain performance criterion, often represented as a cost function, over a defined period for dynamic systems.
An example of this theory in action is the trajectory optimisation for interplanetary missions, where the goal is to reach a target planet using minimal fuel. Here, the Optimal Control Theory is utilised to calculate the most fuel-efficient path, taking into account variables such as gravitational forces and spacecraft mass dynamics.
The development of efficient algorithms for solving optimal control problems is an active area of research in aerospace engineering, often involving simulation techniques for complex scenarios.
Real-World Aerospace Applications of Optimal Control Theory
Optimal Control Theory finds extensive use in enhancing the capabilities and performance of both aircraft and spacecraft. Its applications in real-world aerospace scenarios include autopilot systems for aircraft, where the technology optimizes flight paths for safety and fuel efficiency, and in spacecraft, for tasks such as orbit insertion and manoeuvres within space missions.
One notable application is in the area of satellite constellation deployment, where optimal control strategies ensure the placement of satellites in orbits that maximise coverage and communication capabilities while minimising the risk of collisions and redundancy.
Delving deeper into spacecraft manoeuvres, optimal control theory plays a critical role in rendezvous operations, which are crucial for satellite servicing missions and International Space Station (ISS) re-supply missions. By formulating and solving an optimal control problem, controllers can plot a trajectory that minimises fuel consumption while safely guiding the spacecraft to its target. This application underscores the importance of Optimal Control Theory in advancing sustainable and cost-effective space exploration.
Optimal Control Theory's versatility allows for its application not only in trajectory and mission planning but also in developing adaptive control systems for unmanned aerial vehicles (UAVs), which adjust flight parameters in real-time for mission success.
Adaptive Control for Aerospace Applications
Adaptive Control represents a fundamental approach within aerospace engineering, focusing on creating systems that can adjust and improve their performance in real-time, based on environmental and internal system changes. This approach is crucial for dealing with the unpredictable and dynamic conditions encountered in aerospace applications.
The Importance of Adaptive Control in Aerospace Systems
Adaptive control methodologies are essential in aerospace because they ensure the safety, reliability, and efficiency of flight operations. These systems can dynamically adjust to changes in aircraft dynamics, such as weight reduction due to fuel consumption, damages to control surfaces, or variable wind conditions.
Moreover, adaptive control systems are designed to automatically compensate for system uncertainties or changes without requiring manual recalibration, thus catering to the high-safety standards requisite in aerospace operations.
Adaptive Control: A system that modifies its behaviour in response to changes in the external environment or in the system itself, aiming to continue optimal performance.
The versatility of adaptive control systems makes them suitable for various aerospace applications, ranging from commercial aviation to unmanned aerial vehicles and space exploration missions.
Examples of Adaptive Control in Aerospace Engineering
In aerospace engineering, adaptive control technologies have been successfully implemented in a variety of contexts. Here are some examples illustrating their practical applications:
- Autopilot systems on commercial aircraft, which adjust flight parameters to maintain optimal flight paths and stability, even when faced with shifting weather conditions or system failures.
- Engine control units (ECUs) that adjust the fuel-to-air ratio for jet engines, optimising performance throughout the range of operational conditions.
- Attitude control systems in spacecraft, which ensure that the orientation is maintained for operations like satellite communication or solar panel alignment towards the sun.
An illustrative example of adaptive control in action can be found in the Mars Rover operations. The Rovers are equipped with adaptive control systems that allow them to navigate the Martian terrain by autonomously adjusting their speed and direction based on the immediate environment. This capability is crucial for avoiding obstacles and ensuring the Rover's mission objectives are achieved.
Delving deeper, the adaptive control system within the Mars Rover utilises a combination of sensors and algorithms to constantly update its internal model of the terrain. By processing real-time data from its environment, the system can predict potential hazards and adjust its course accordingly. This example showcases the sophisticated nature of adaptive control systems and their ability to process complex data sets for autonomous decision-making in aerospace engineering.
Adaptive control systems in aerospace often incorporate advanced technologies such as machine learning and AI to improve their decision-making capabilities over time.
Advanced Aerospace Control Techniques
Advanced aerospace control techniques are pivotal in pushing the boundaries of what is achievable in the modern aerospace sector. These techniques enable aircraft and spacecraft to perform complex tasks, ranging from routine flight operations to intricate manoeuvres in space, with increased efficiency, safety, and reliability.
Robust and Adaptive Control with Aerospace Applications
Robust and adaptive control systems are designed to maintain high performance of aerospace vehicles in the face of uncertainties and changing operational conditions. They use algorithms that can adjust control strategies based on real-time feedback, ensuring reliable functionality even when parameters vary unpredictably.
Robust control focuses on ensuring system stability and performance despite fluctuations, while adaptive control modifies system parameters in real-time to cope with environmental changes and system dynamics.
Adaptive Control: A type of control system that dynamically adjusts its parameters and control strategy in response to changes in the internal or external environment, to maintain or improve system performance.
An example of robust and adaptive control is in maintaining an aircraft's flight in turbulent weather conditions. The system dynamically adjusts the aircraft's control surfaces to counteract the effects of sudden wind changes, preserving stability and comfort.
Linear Parameter Varying Control and Its Application to Aerospace Systems
Linear Parameter Varying (LPV) control is an advanced method that deals with systems whose dynamics can be described by linear models that vary with certain parameters. In aerospace, LPV control is used to design controllers for vehicles whose flight dynamics change significantly during operation, such as transitions from subsonic to supersonic flight.
Linear Parameter Varying Control: A control strategy that manages systems modelled by linear equations, where the parameters of these equations change over time or operational conditions.
The control system of a variable-sweep wing aircraft employs LPV control to manage the aerodynamics as the wing's shape changes during flight, ensuring optimal performance across a wide range of airspeeds and manoeuvres.
Dynamics and Control of Highly Flexible Structures for Aerospace Applications
The dynamics and control of highly flexible structures, such as long-span wings or deployable space structures, present unique challenges in aerospace engineering. These structures can experience significant deformations during operation, affecting the stability and control of the vehicle or system.
Modern control techniques, including both feedback and feedforward control strategies, are employed to manage these dynamic deformations. Such controls are essential for the successful operation of aerospace vehicles and equipment, ensuring that they can withstand and adapt to the physical stresses encountered during flight and space missions.
The control of highly flexible structures often involves sophisticated modelling and simulation to predict dynamic behaviour and to design effective control strategies.
Deployable space structures, such as solar arrays and antennas, utilise control systems that must compensate for the lack of damping in the space environment. These systems incorporate advanced algorithms to predict and mitigate potential oscillations and deformations, ensuring the structure's functionality and longevity in orbit.
Aerospace Control Applications - Key takeaways
- Aerospace Control Applications: Technologies managing safety, stability, and efficiency of aircraft and spacecraft operations.
- Aerospace Control System: Integrated system with sensors, actuators, and algorithms ensuring the desired performance of aerospace vehicles.
- Optimal Control Theory: Mathematical framework optimizing dynamic systems' performance under constraints, commonly used for trajectory optimization and fuel consumption in aerospace.
- Adaptive Control: Systems that adjust performance in real-time to environmental and internal changes, key for unpredictable conditions in aerospace.
- Linear Parameter Varying Control: Strategy for managing systems with dynamics represented by time or condition-varying linear models, crucial for vehicles with changing flight dynamics.
Learn faster with the 12 flashcards about Aerospace Control Applications
Sign up for free to gain access to all our flashcards.
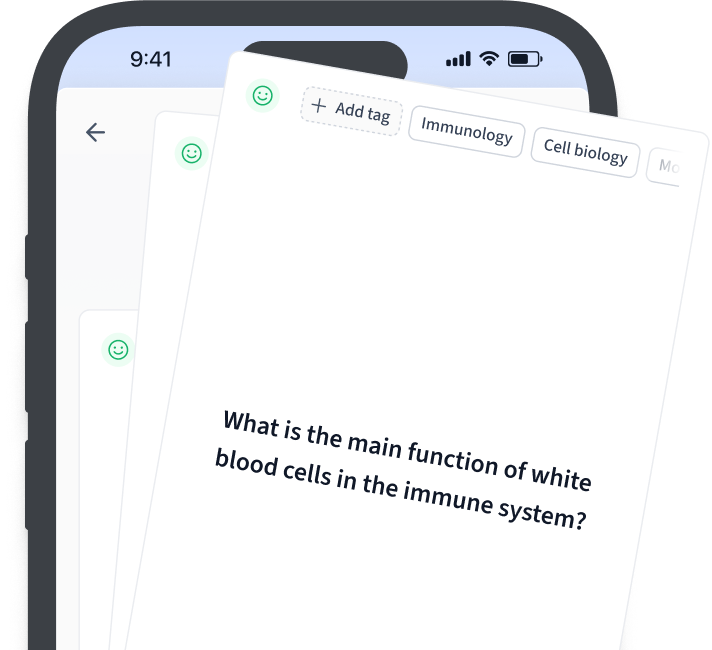
Frequently Asked Questions about Aerospace Control Applications
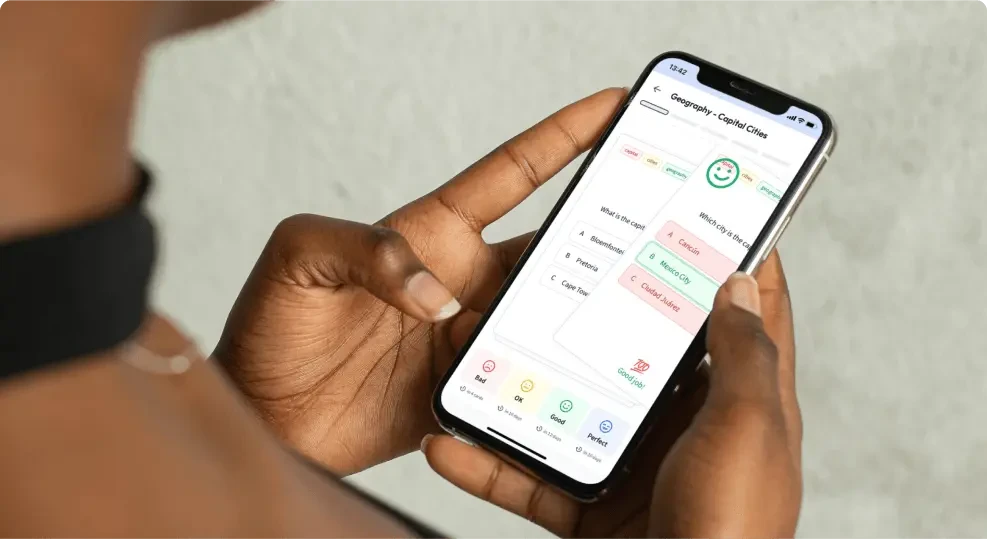
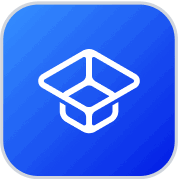
About StudySmarter
StudySmarter is a globally recognized educational technology company, offering a holistic learning platform designed for students of all ages and educational levels. Our platform provides learning support for a wide range of subjects, including STEM, Social Sciences, and Languages and also helps students to successfully master various tests and exams worldwide, such as GCSE, A Level, SAT, ACT, Abitur, and more. We offer an extensive library of learning materials, including interactive flashcards, comprehensive textbook solutions, and detailed explanations. The cutting-edge technology and tools we provide help students create their own learning materials. StudySmarter’s content is not only expert-verified but also regularly updated to ensure accuracy and relevance.
Learn more