Jump to a key chapter
Understanding Aerospace Engineering Theory
Aerospace engineering theory encompasses a broad range of concepts and principles that are essential to the design, development, and operation of aircraft and spacecraft. This field combines elements of mechanical engineering, electrical engineering, and materials science to address complex challenges in air and space travel.
Fundamentals of Aerospace Propulsion Theory and Engineering
The fundamentals of aerospace propulsion focus on the mechanisms that drive an aircraft or spacecraft forward. Understanding these principles is crucial for engineering efficient and powerful engines. Among the key concepts covered are the physics of thrust generation, the efficiency of propulsion systems, and the performance characteristics of different types of engines.
Thrust: The force applied by an engine or motor to move an aircraft or spacecraft in the opposite direction to the flow of exhaust gas or fluid.
Example: A jet engine generates thrust by expelling hot exhaust gases backward, pushing the aircraft forward as a reaction (Newton's third law of motion).
Different propulsion systems utilized in aerospace include jet engines, rocket motors, and propellers, each offering unique advantages and suited for specific flight conditions. It is essential to understand how these systems interact with the atmosphere or space environment to optimize performance.
Flight Dynamics and Control Theory for Aerospace Engineering
Flight dynamics and control theory address the physical laws and forces that govern the motion of aircraft and spacecraft. This area of aerospace engineering theory focuses on stability, control systems, and the manoeuvrability of flying vehicles.
Stability: The ability of an aircraft or spacecraft to maintain its course or to return to a desired path after being disturbed, without corrective action by the pilot or autopilot systems.
Example: An airplane is designed with specific tail fin and wing configurations to ensure it remains stable during flight and can recover from minor disturbances due to turbulent air.
Engineers use advanced mathematics and computer simulations to model flight dynamics, allowing them to predict how vehicles will respond to various conditions and control inputs. This understanding is vital for designing systems that can navigate accurately and safely through the Earth's atmosphere or space.
Introduction to Classical Laminate Theory Lesson 1: Advanced Course in Aerospace Engineering
The classical laminate theory is a sophisticated approach used in aerospace engineering to analyse and design composite materials. Composites, such as carbon fibre reinforced plastics, are frequently used in the aerospace industry for their strength and lightweight characteristics.
Classical Laminate Theory: A theory that models how different layers (laminates) of composite materials behave under various loading conditions, including stress, strain, and temperature changes.
Example: The wings of modern aircraft are often made from composite materials designed using classical laminate theory to withstand the aerodynamic forces encountered during flight while minimizing weight.
Learners will explore the mathematical models and principles behind the classical laminate theory, gaining insights into material selection, structural analysis, and the design considerations necessary for creating efficient aerospace structures.
Did you know? The use of composite materials in aerospace has significantly increased flight efficiency and reduced fuel consumption, showcasing the impact of advanced engineering theories in practical applications.
Orbital Mechanics in Aerospace Engineering
Orbital mechanics, a fundamental branch of aerospace engineering, plays a critical role in understanding and designing the trajectories of spacecraft and satellites. This field applies the principles of physics and mathematics to predict the paths that celestial bodies will follow under the influence of gravitational forces.
Orbital Mechanics Fundamental Concepts
At the heart of orbital mechanics are key concepts like Newton's laws of motion and the law of universal gravitation. These principles help in calculating the orbits of objects in space, ensuring satellites and spacecraft maintain their intended paths.
Orbit: The path of a body as it moves under the influence of gravitational force, typically around a larger body.
Example: The International Space Station (ISS) follows a low Earth orbit, circling the planet approximately every 90 minutes.
The basic equation of motion for a body in orbit, derived from Newton's laws and his gravitational theory, is given by: \[ F = \frac{Gm_1m_2}{r^2} \], where F is the gravitational force between two masses, G is the gravitational constant, m with subscripts represent the masses involved, and r is the distance between the centres of the two masses.
The Importance of Gravity and Velocity in Space Exploration
Gravity and velocity are pivotal in determining the success of space exploration missions. Managing these factors correctly allows spacecraft to enter orbits, perform manoeuvres, and travel between celestial bodies efficiently.
Escape velocity: The minimum speed needed for an object to break free from the gravitational attraction of a planet or moon without further propulsion.
Example: To leave Earth's gravitational field and travel to the moon, a spacecraft must reach the escape velocity of about 11.2 kilometres per second.
The relation between gravity and orbital velocity is crucial for keeping satellites in orbit. This balance is given by the equation: \[ v = \sqrt{\frac{Gm}{r}} \], where v is the orbital velocity needed to maintain an orbit at distance r from the centre of the Earth, and m is the mass of the Earth.
- Understanding this balance helps in planning fuel-efficient trajectories and maintaining satellites in their designated orbits.
- It also underpins the concept of gravitational assists or 'slingshot' manoeuvres used by interplanetary missions to gain speed without using fuel.
The interplay between gravity and velocity not only enables the stable orbiting of satellites but also facilitates complex manoeuvres like geostationary transfers and interplanetary travel. By harnessing gravitational forces from planets, spacecraft can 'slingshot' themselves into higher speeds and more distant trajectories, showcasing the exquisite dance between the force of gravity and the strategic use of velocity.
Aerospace Engineering Aerodynamics Principles
Aerospace engineering focuses heavily on understanding the forces of aerodynamics and how they interact with aircraft structures. These principles are crucial for designing aircraft that are both efficient and safe.
How Airflow and Pressure Impact Aircraft Design
The design of any aircraft must account for how air flows around the structure, which is determined by the science of aerodynamics. Airflow and pressure variations significantly impact an aircraft's performance characteristics, including its speed, stability, and fuel efficiency.
Aerodynamics: The study of the motion of air, particularly its interaction with a solid object, such as an airplane wing.
Example: An aerodynamically designed wing will produce lift by creating a pressure difference between the upper and lower surfaces.
The shape of wings, fuselage, and other components significantly influences how air flows around them. These shapes are designed to reduce drag—resistance to the aircraft's movement through the air—and to generate sufficient lift, which is the force that allows an aircraft to rise off the ground.
- Lift is generated primarily by the wings.
- Drag is minimised through streamlined shapes.
- Pressure differences around the aircraft parts are manipulated to improve performance and efficiency.
Understanding Lift, Drag, and Thrust in Aerospace Engineering
In aerospace engineering, understanding the forces of lift, drag, and thrust is essential for designing aircraft that can fly efficiently and safely. Lift is the force that directly opposes the weight of the aircraft and holds it in the air. Drag is the resistance an aircraft faces as it moves through the air. Thrust is the force produced by the aircraft's engines to move it forward through the air.
Drag: The aerodynamic force that opposes an aircraft's motion through the air.
Thrust: The force generated by the aircraft's engines to propel it forward.
Example: Jet engines provide thrust that propels the aircraft forward, allowing the wings to generate lift to counteract gravity.
The balance between these forces is delicate and varies with the speed of the aircraft, the shape of its body, and the density of the air. Engineers use sophisticated design and testing, including computational fluid dynamics (CFD) simulations, to understand and optimise these interactions for each aircraft design.
The mathematical foundation for understanding these forces is provided by the Navier-Stokes equations, which describe how the velocity field flowing around an object changes. These equations, although complex, are central to the computational simulations that predict airflow patterns, pressure distribution, and the resultant forces on an aircraft.
Did you know? The angle of attack of an aircraft wing—one of the critical design parameters—is the angle between the wing's chord line and the oncoming air. Adjusting this angle is a key method for controlling lift and drag during flight.
Materials Used in Aerospace Engineering
Materials used in aerospace engineering play a pivotal role in the development and operation of both aircraft and spacecraft. The selection of materials is critical, given the extreme conditions these vehicles face, such as high velocities, thermal stress, and the harsh environment of space.
Aerospace Materials Engineering Basics
The fundamentals of aerospace materials engineering involve understanding the properties and applications of various materials within the field. These materials must possess characteristics such as high strength-to-weight ratio, durability, and resistance to temperature extremes and corrosion.
High strength-to-weight ratio: A measure of a material's strength relative to its weight. This ratio is critical in aerospace engineering to ensure structures are both strong and lightweight.
Example: Titanium and its alloys, known for their high strength-to-weight ratio, are widely used in critical aerospace structures, such as engine components and airframes.
Aerospace engineers often work with a variety of metals, composites, and polymers:
- Metals such as aluminium, titanium, and steel are traditional choices due to their mechanical properties and durability.
- Composites like carbon fibre reinforced plastics offer exceptional strength and reduced weight, vital for fuel efficiency.
- Polymers, including rubbers and plastics, are selected for their flexibility, corrosion resistance, and low weight.
Innovations in Materials for Spacecraft and Aircraft Structures
Recent innovations in materials for spacecraft and aircraft structures have led to the development of advanced materials that push the boundaries of aerospace engineering. These advancements aim to enhance performance, increase safety, and reduce environmental impact.
Shape memory alloys (SMAs): Metal alloys that can return to their original shape after being deformed, useful for self-healing structures.
Did you know? Graphene, a single layer of carbon atoms arranged in a hexagonal lattice, is being explored for its potential in aerospace applications due to its strength, flexibility, and conductivity.
Innovations include the use of:
- Nano-materials and graphene for stronger and lighter structures.
- 3D printing (additive manufacturing) technologies for complex, lightweight component fabrication.
- Shape memory alloys (SMAs) for parts that can adapt or heal themselves under various conditions.
One of the most promising areas of innovation is the development of bio-inspired materials. These materials mimic the structure and properties of natural materials and organisms, offering novel solutions for self-healing structures, improved aerodynamics, and enhanced thermal insulation. By studying the wings of birds or the skin of sharks, engineers can design aircraft and spacecraft with revolutionary efficiency and safety features.
Aerospace Engineering Theory - Key takeaways
- Aerospace Engineering Theory: A combination of mechanical engineering, electrical engineering, and materials science to tackle air and space travel challenges.
- Aerospace Propulsion: Concepts including thrust generation, propulsion system efficiency, and various engine types such as jet engines, rocket motors, and propellers.
- Flight Dynamics and Control Theory: The study of forces governing the motion of flying vehicles, focusing on stability, control systems, and manoeuvrability.
- Classical Laminate Theory: An analytical method in advanced aerospace engineering for designing composite materials, accounting for stress, strain, and temperature effects on laminates.
- Orbital Mechanics: Application of physics and mathematics to predict spacecraft trajectories, involving concepts like Newton's laws of motion and the law of universal gravitation.
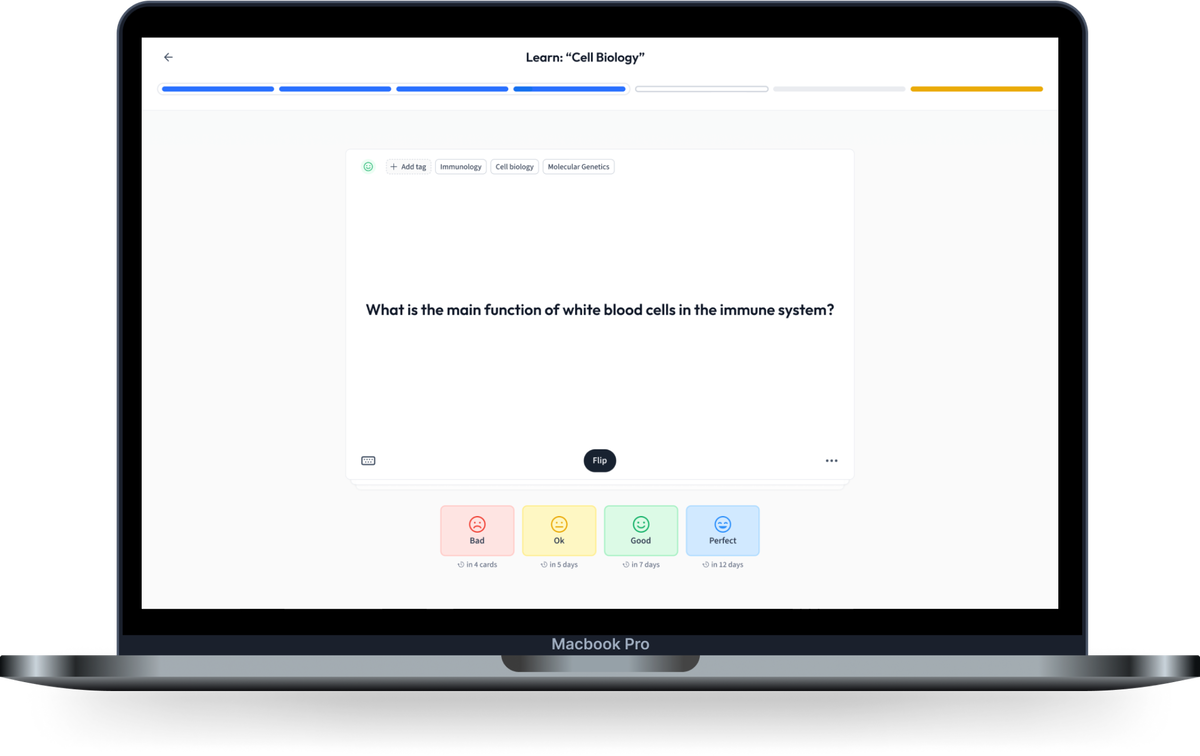
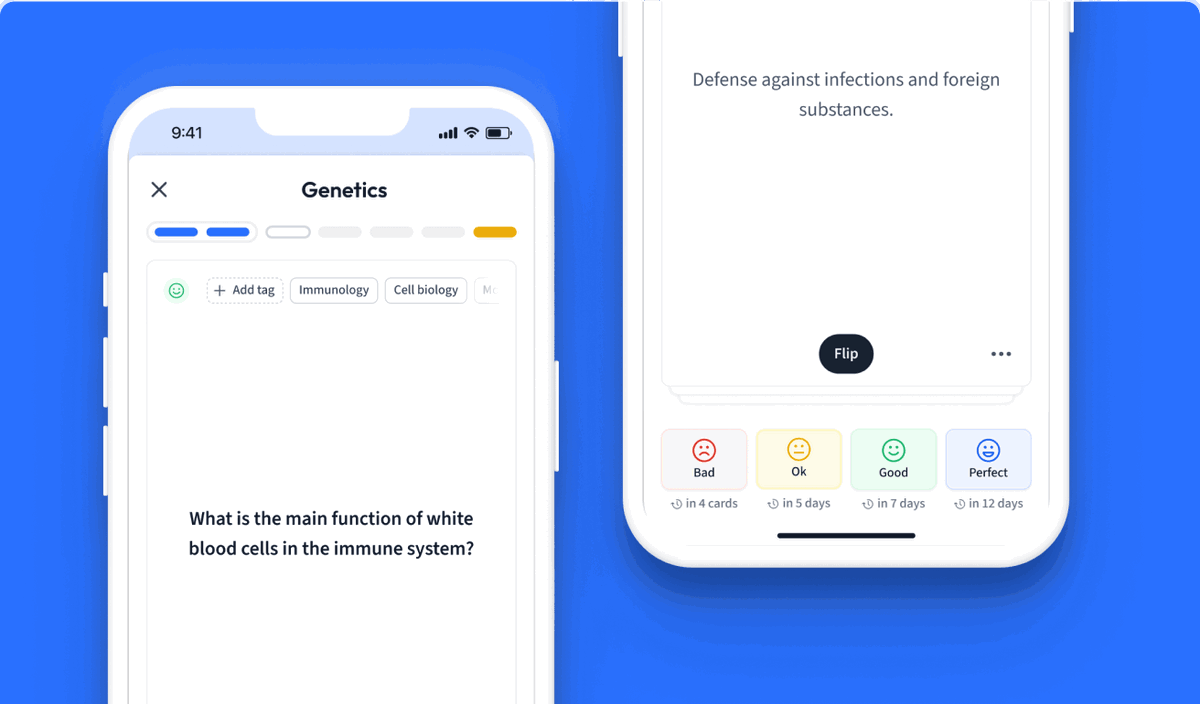
Learn with 12 Aerospace Engineering Theory flashcards in the free StudySmarter app
Already have an account? Log in
Frequently Asked Questions about Aerospace Engineering Theory
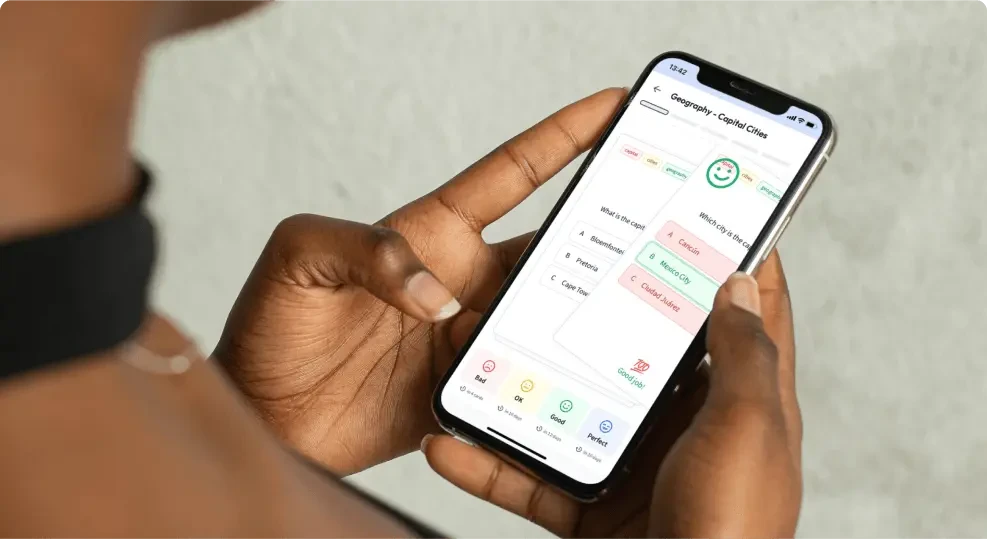
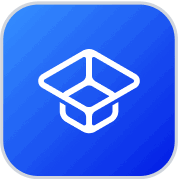
About StudySmarter
StudySmarter is a globally recognized educational technology company, offering a holistic learning platform designed for students of all ages and educational levels. Our platform provides learning support for a wide range of subjects, including STEM, Social Sciences, and Languages and also helps students to successfully master various tests and exams worldwide, such as GCSE, A Level, SAT, ACT, Abitur, and more. We offer an extensive library of learning materials, including interactive flashcards, comprehensive textbook solutions, and detailed explanations. The cutting-edge technology and tools we provide help students create their own learning materials. StudySmarter’s content is not only expert-verified but also regularly updated to ensure accuracy and relevance.
Learn more