Jump to a key chapter
What are Ceramic Matrix Composites?
Ceramic Matrix Composites (CMCs) represent a subgroup of composite materials that are developed from ceramic fibres embedded in a ceramic matrix. They offer a blend of properties such as high-temperature stability, corrosion resistance, and increased toughness over traditional ceramics, making them suitable for a wide range of high-performance applications.
Ceramic Matrix Composites Definition
Ceramic Matrix Composites (CMCs): A composite material consisting of ceramic fibres embedded in a ceramic matrix. The matrix supports the fibre reinforcement, distributing loads and improving the material's overall mechanical properties.
Ceramic Matrix Composites Properties
Ceramic Matrix Composites are renowned for their exceptional properties, which enable them to perform in extreme environments. These properties include:
- High-temperature stability: They retain structural integrity and mechanical properties at temperatures where metals and polymers would fail.
- Corrosion and wear resistance: CMCs resist degradation from chemical exposure and abrasive conditions, prolonging their service life.
- Low density: Their lightweight nature makes them ideal for aerospace and automotive applications where weight reduction is crucial.
- Enhanced thermal shock resistance: The ability to withstand rapid temperature changes without cracking or degrading.
The incorporation of fibers into the ceramic matrix not only improves its mechanical properties but also contributes to the composite's damage tolerance.
Examples of Ceramic Matrix Composites
Carbon reinforced carbon (C/C): Ideal for applications requiring the highest temperature resistance, such as rocket nozzles and brake systems for high-performance cars.
Silicon carbide reinforced silicon carbide (SiC/SiC): Known for its use in high-temperature turbine blades and nuclear reactor components, SiC/SiC composites offer exceptional heat resistance and durability.
Alumina reinforced with zirconia (Al2O3/ZrO2): This combination provides excellent wear resistance, making it suitable for biomedical implants and cutting tools.
The Mechanical Properties of Ceramic Matrix Composites
Ceramic Matrix Composites (CMCs) have garnered significant attention for their superior mechanical properties that make them indispensable in various high-performance engineering applications. Understanding these properties is crucial in exploiting the full potential of CMCs in design and application.
Understanding Ceramic Matrix Composites Mechanical Properties
The mechanical properties of Ceramic Matrix Composites are a result of their composite nature, which combines the toughness of the fibres with the stiffness and strength of the matrix. This synergy results in materials that exhibit unique characteristics:
- Tensile Strength: The ability of CMCs to withstand loads that tend to elongate the material. This is a critical property for components under tension.
- Compression Strength: The capacity of CMCs to withstand loads that tend to compress the material, especially important in structural applications.
- Flexural Strength: The capability of CMCs to resist deformation under bending loads, relevant for many structural and aerospace components.
- Toughness: Unlike traditional ceramics, CMCs can absorb significant amounts of energy before failure, thanks to the reinforcement provided by the fibres.
- Thermal Conductivity: CMCs can be tailored to have low or high thermal conductivity based on the requirement of the application.
The mechanical properties of Ceramic Matrix Composites often lead to their selection over traditional materials in demanding environments, such as in aerospace and automotive applications.
Factors influencing these mechanical properties include the type of fibres and matrix used, the quality of the fibre-matrix interface, the processing method, and the presence of any defects or voids. Each of these factors can significantly impact the overall performance of the CMC.
Fibre-Matrix Interface: The region where the fibre and the matrix interact within a composite material. The characteristics of this interface are crucial to the mechanical properties of the composite.
The optimization of the fibre-matrix interface is a key research area. A strong, yet not too rigid, interface is essential to ensure load transfer from the matrix to the fibres while allowing for some plastic deformation, which contributes to the material's toughness and resistance to crack propagation.
To delve deeper into the analysis of Mechanical Properties of Ceramic Matrix Composites, researchers often employ advanced techniques such as scanning electron microscopy (SEM) for microstructural analysis, and finite element modelling (FEM) to simulate and predict stress distribution and crack propagation in complex CMC structures. Such advanced methods offer insights into the fundamental behaviours of CMCs under various loading conditions, guiding the development of new composites with tailored properties.
Example of Application: Silicon carbide reinforced silicon carbide (SiC/SiC) composites are deployed in the hot-section components of jet engines. Their superior tensile and flexural strength, combined with excellent high-temperature stability, make them ideal for such demanding applications.
Advantages and Disadvantages of Ceramic Matrix Composites
Ceramic Matrix Composites (CMCs) offer a unique set of advantages and disadvantages that make them suitable for some applications while less desirable for others. By understanding both sides, engineers and designers can make informed decisions when selecting materials for their projects.
Exploring the Benefits of Ceramic Matrix Composites
The benefits of Ceramic Matrix Composites stem from their distinctive combination of ceramic materials and reinforcing fibres, offering improved performance in challenging environments. Some of the key advantages include:
- High-temperature performance: CMCs maintain their strength and integrity at temperatures where metals and conventional ceramics would weaken or melt.
- Corrosion resistance: These materials resist corrosion from chemicals and environmental factors, extending their usable life in aggressive environments.
- Wear resistance: The toughness and hardness of CMCs make them highly resistant to wear, useful in abrasive conditions.
- Lightweight: Compared to metals, CMCs are lighter, which is advantageous in aerospace and automotive applications where weight reduction is critical.
- Thermal shock resistance: CMCs are able to withstand rapid temperature changes without damage, unlike traditional ceramics that may crack under thermal stress.
The unique combination of durability, low weight, and high-temperature resistance makes CMCs particularly valuable in the aerospace and automotive industries, where performance and efficiency are paramount.
The Limitations of Ceramic Matrix Composites
Despite their impressive attributes, Ceramic Matrix Composites also possess certain limitations that restrict their use in some applications. Key drawbacks include:
- High cost: The manufacturing processes for CMCs are complex and resource-intensive, leading to higher costs compared to traditional materials.
- Brittleness: Although CMCs are tougher than conventional ceramics, they can still be brittle compared to metals, limiting their use in applications requiring ductility.
- Limited large-scale manufacturing capabilities: The production of CMCs can be challenging to scale up efficiently, which restricts the availability of large quantities for industrial use.
- Complexity in Joining: Connecting CMC components with other materials can be difficult, demanding specialised techniques.
- Specialised machining: CMCs require advanced machining tools and processes, adding to the overall cost of the finished product.
Example of Limitation in Application: The high cost and specialised machining requirements of Ceramic Matrix Composites make them less viable for consumer electronic applications where cost efficiency is a critical factor.
To address some of these limitations, significant research and development efforts are ongoing. Innovations in manufacturing technologies, such as additive manufacturing (3D printing of ceramics), and the development of new matrix materials and fibre coatings, aim to reduce costs, improve scalability, and enhance the mechanical properties of CMCs. These advancements have the potential to widen the applicability of CMCs across more industries by making them more cost-effective and versatile.
Applications of Ceramic Matrix Composites
Ceramic Matrix Composites (CMCs) have significantly expanded the horizon of high-performance materials, offering superior properties over traditional materials in numerous industrial applications. Understanding where and how these composites are used illuminates the importance of material engineering in today's technological advancements.
How Ceramic Matrix Composites are Used in Aerospace Engineering
In the realm of aerospace engineering, Ceramic Matrix Composites stand out for their remarkable ability to withstand the extreme conditions encountered during flight and space exploration. Their application in this sector includes:
- Engine Components: CMCs are crucial in manufacturing parts like turbine blades, vanes, and combustor liners. They tolerate high temperatures, allowing engines to operate more efficiently by reducing cooling requirements and increasing fuel efficiency.
- Thermal Protection Systems: Spacecraft benefit from CMCs in their thermal protection systems, where materials must survive the intense heat generated during re-entry into the Earth's atmosphere.
- Brake Systems: The high-temperature resistance and excellent wear properties of CMCs make them ideal for use in brake systems of aircraft, providing reliable performance under heavy loads and high-speed conditions.
Focusing on the use of Silicon Carbide reinforced Silicon Carbide (SiC/SiC) in aerospace, this composite not only demonstrates increased thermal resistance but also improves overall component performance under high stress. Advanced testing methodologies, including thermo-mechanical fatigue testing, illustrate how SiC/SiC composites maintain integrity over long durations, highlighting their suitability for critical aero-engine parts.
Other Critical Application Areas of Ceramic Matrix Composites
Beyond aerospace, Ceramic Matrix Composites find utility in several other demanding environments, showcasing the versatility of these materials:
- Automotive: The automotive industry uses CMCs for components like brake discs and exhaust systems, where high temperature and wear resistance are paramount.
- Energy Production: In power generation, particularly in nuclear reactors, CMCs are used for their radiation resistance and ability to withstand high temperatures without degrading.
- Biomedical Devices: The biocompatibility and strength of certain CMCs, such as alumina matrix composites, make them suitable for implantable devices, such as joint replacements.
The rapid advancement of Ceramic Matrix Composite technology is constantly opening new application areas, from electronics to protective coatings, thereby broadening the impact of these materials in modern engineering and manufacturing sectors.
Ceramic Matrix Composites - Key takeaways
- Ceramic Matrix Composites (CMCs) Definition: Composite materials made from ceramic fibres embedded in a ceramic matrix, characterised by high-temperature stability, corrosion resistance, and increased toughness.
- Ceramic Matrix Composites Properties: Notable for high-temperature stability, corrosion and wear resistance, low density, and enhanced thermal shock resistance.
- Examples of Ceramic Matrix Composites: Carbon reinforced carbon (C/C), silicon carbide reinforced silicon carbide (SiC/SiC), and alumina reinforced with zirconia (Al2O3/ZrO2).
- Advantages and Disadvantages of Ceramic Matrix Composites: High-temperature performance and lightweight but limited by high cost, complexity in joining, and the requirement for specialised machining.
- Application of Ceramic Matrix Composites: Extensively used in aerospace engineering (e.g., engine components), automotive (e.g., brake systems), energy production, and biomedical devices.
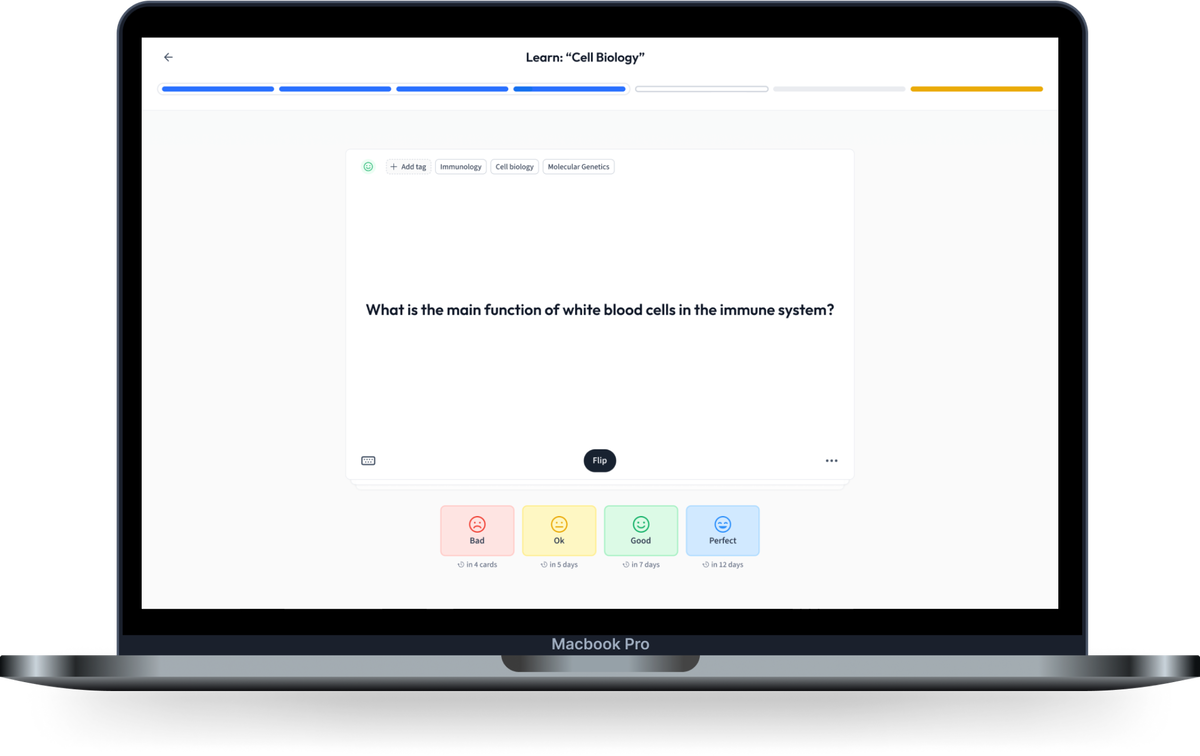
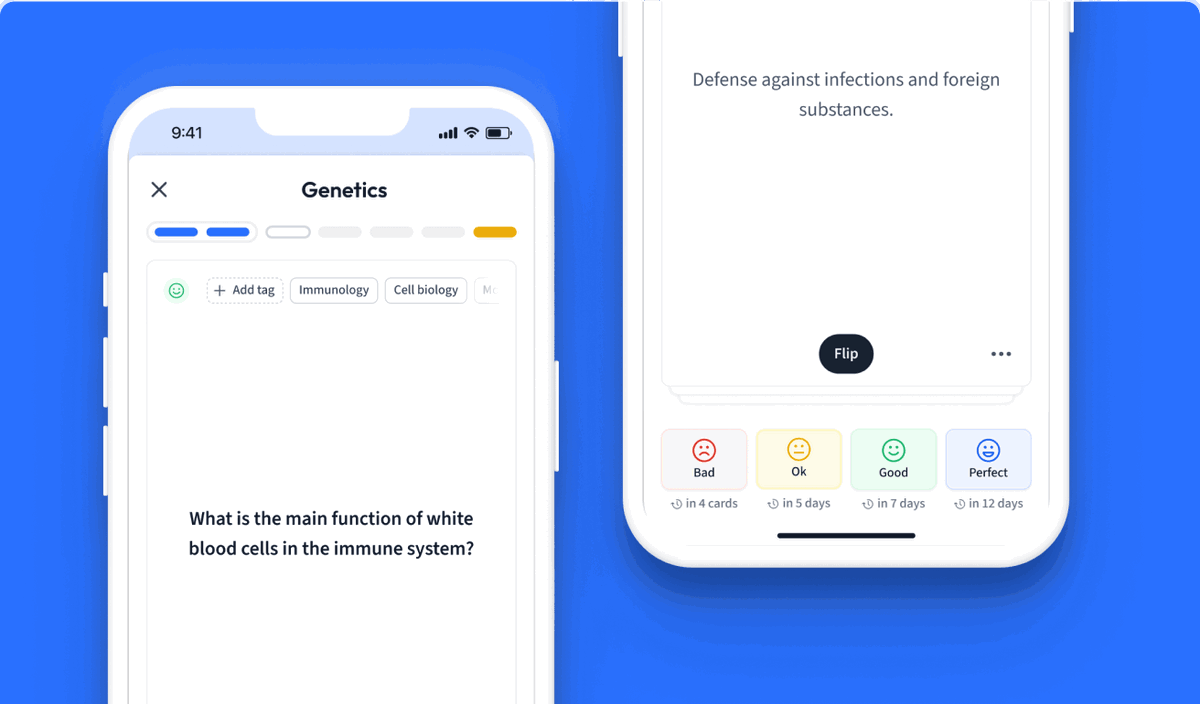
Learn with 12 Ceramic Matrix Composites flashcards in the free StudySmarter app
Already have an account? Log in
Frequently Asked Questions about Ceramic Matrix Composites
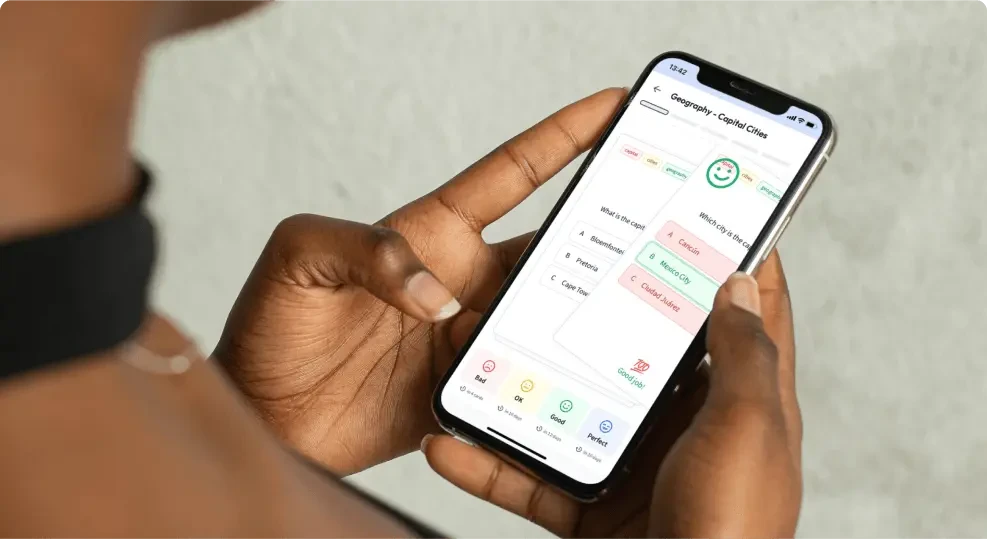
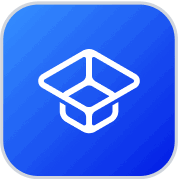
About StudySmarter
StudySmarter is a globally recognized educational technology company, offering a holistic learning platform designed for students of all ages and educational levels. Our platform provides learning support for a wide range of subjects, including STEM, Social Sciences, and Languages and also helps students to successfully master various tests and exams worldwide, such as GCSE, A Level, SAT, ACT, Abitur, and more. We offer an extensive library of learning materials, including interactive flashcards, comprehensive textbook solutions, and detailed explanations. The cutting-edge technology and tools we provide help students create their own learning materials. StudySmarter’s content is not only expert-verified but also regularly updated to ensure accuracy and relevance.
Learn more