Jump to a key chapter
Understanding Convection Heat Transfer
In exploring the fascinating world of engineering, understanding how heat moves through environments is crucial. Convection heat transfer is one pivotal concept that underpins many of the processes and technologies you interact with daily. From the natural cooling of your body to the sophisticated systems that keep buildings and electronic devices operating within safe temperature ranges, convection plays an integral role.Here, we'll delve into what convection heat transfer is, its importance, and the various types it encapsulates. This knowledge not only broadens your understanding of thermal management solutions but also equips you with insights into the engineering principles that optimise our everyday lives.
What is Convection Heat Transfer?
Convection Heat Transfer: Is the transfer of thermal energy between an area of higher temperature and one of lower temperature, facilitated by the fluid (liquid or gas) movement.
This mechanism is behind many phenomena in nature and technology, where heat needs to migrate from one place to another. In simple terms, when a fluid such as air or water gets heated, it increases in volume and decreases in density. This lower-density fluid then rises, while cooler, denser fluid moves in to take its place, creating a cycle that results in the efficient transfer of heat.Convection can be seen in numerous everyday occurrences - a boiling kettle, the circulation of air by a fan in a hot room, or even the weather patterns across the globe. The principle of convection is also applied in engineering designs to optimise the cooling of machinery, electronics, and buildings.
Did you know that the Earth's mantle moves by convection, contributing to phenomena like volcanic eruptions and continental drift?
Types of Convection Heat Transfer
Convection heat transfer can manifest in two primary forms: natural (or free) convection and forced convection. Each of these plays a key role in engineering and environmental phenomena, leveraging the principle of fluid movement to facilitate heat transfer.
Natural Convection occurs without any external force applied. It relies on the natural tendency of fluid to move when it is heated, becoming less dense and rising. This type is commonly observed in household heating, geological phenomena, and even in the cooling of electronic devices in some contexts.Forced Convection, on the other hand, involves an external source, such as a pump or fan, to accelerate the fluid movement. This type is vital in many industrial processes and systems such as air-conditioning units, automotive cooling systems, and industrial heat exchangers.
To illustrate, consider a radiator in a room as an example of natural convection. The air in contact with the radiator warms up, becomes less dense, and rises. Cooler air then moves in to replace it, creating a circulation pattern that distributes heat throughout the room. Conversely, in forced convection, a fan behind a radiator can push colder air across its surface more effectively, amplifying the heat transfer rate and making the heating process more efficient.
Understanding the nuances between natural and forced convection is critical for designing systems that require precise thermal management. Engineers select the type of convection based on factors such as the required rate of heat transfer, the environmental conditions, and energy efficiency considerations.
Type | Driving Force | Common Applications |
Natural Convection | Temperature differences within the fluid | Home heating, geographical phenomena |
Forced Convection | External mechanical devices (fans, pumps) | Cooling of electronic equipment, automotive cooling systems |
Exploring the Convection Heat Transfer Equation
The principles of heat transfer are fundamental in engineering, playing a critical role in everything from climate control in buildings to the thermal management of electronic devices. Among the various mechanisms of heat transfer, convection brings unique challenges and opportunities for innovation. Understanding the convection heat transfer equation not only allows engineers to design more efficient systems but also aids students in grasping the complexities of thermal physics. In this section, we'll dive into the components and significance of this equation, providing a basis for advanced study and practical application.By dissecting the formula that governs convection heat transfer, you gain insight into how engineering principles are applied to real-world problems, enhancing the efficiency and effectiveness of thermal management solutions.
Breaking Down the Heat Transfer Formula for Convection
At the heart of understanding convection heat transfer is its governing equation, which quantifies the rate at which heat is transferred from one place to another through a fluid medium. This equation is essential for designing and optimising a wide range of engineering systems.The general formula for convective heat transfer can be expressed as: Q = h * A * (T_s - T_f), where:
- Q represents the rate of heat transfer,
- h is the convective heat transfer coefficient,
- A denotes the surface area through which heat is being transferred, and
- T_s and T_f are the temperatures of the surface and the fluid, respectively.
For example, in a heating, ventilation, and air conditioning (HVAC) system, engineers use this equation to determine the size and type of units needed to efficiently maintain the desired temperature in a building. By adjusting variables such as the surface area of the heat exchanger or the properties of the fluid, designers can optimise the system for energy efficiency and performance.
Interestingly, the value of the convective heat transfer coefficient, h, varies significantly depending on the fluid's properties and the flow conditions, making it one of the more challenging variables to accurately determine in the equation.
The Role of the Convective Heat Transfer Coefficient
The convective heat transfer coefficient (h) serves as a measure of the efficiency with which heat can be transferred between a fluid and a surface. Its value is influenced by a multitude of factors, including the type of fluid, its velocity, the surface geometry, and whether the convection is natural or forced. This coefficient is pivotal in the equation for convection heat transfer, as it encapsulates the combined effect of the fluid's thermal conductivity, density, and viscosity, along with the conditions of flow.
- Natural convection typically results in lower h values due to the sole reliance on temperature-induced density differences to drive fluid movement.
- Forced convection, involving external mechanisms like fans or pumps to move the fluid, usually achieves higher h values, indicating more efficient heat transfer.
Exploring further, the process of determining the convective heat transfer coefficient often involves complex calculations and empirical correlations, considering the intricate interplay between fluid dynamics and thermodynamics. For instance, in forced convection scenarios through a pipe, the Nusselt number—a dimensionless quantity expressing the ratio of convective to conductive heat transfer—plays a key role in calculating h. This complexity underscores the importance of meticulous design and testing in thermal engineering, ensuring systems are both effective and efficient.Engineers use a variety of tools, including computational fluid dynamics (CFD) software, to simulate and optimise these processes. Such simulations help predict how altering different variables affects the convective heat transfer coefficient and, by extension, the overall heat transfer rate. It's this level of detailed analysis that enables the development of cutting-edge technologies, from more efficient engines to advanced climate control systems.
Practical Convection Heat Transfer Examples
Convection heat transfer, an essential principle in thermal energy movement, is evident in both natural environments and engineered systems. This process, which involves the transfer of heat by the movement of fluids (liquids or gases), underpins a variety of everyday phenomena and industrial applications. By examining concrete examples of convection, you can gain a deeper understanding of this mechanism and appreciate its importance in designing solutions to managing heat in various contexts.Below, we explore instances where convection heat transfer plays a pivotal role, from warming your home to the intricate cooling systems that protect critical technology components.
Everyday Examples of Convection Heat Transfer
Convection heat transfer is not just a topic of study; it’s a phenomenon that you experience daily. Here are a few examples where convection occurs in everyday life:
- Weather Patterns: Convection drives major weather phenomena. Warm air rises and cool air descends, creating wind and storm patterns.
- Boiling Water: When water in a pot heats up, hot water rises and cooler water moves down to replace it, demonstrating natural convection.
- Heating a Room: Radiators heat the air closest to them. The hot air rises and spreads out, warming the room through convection currents.
- Human Body: Your body also cools down through convection. Heat is transferred from your body to the cooler air around it, especially noticeable in windy conditions.
Imagine using a fan on a hot summer day. The fan moves air over your skin, facilitating the transfer of heat away from your body via convection. This example not only illustrates how convection aids in cooling but also highlights the role of forced convection (using external equipment to move the fluid) in enhancing the comfort of your immediate environment.
Did you know that the principles of convection are also applied in culinary practices? For instance, convection ovens enhance cooking efficiency by circulating hot air around the food, which cooks the food more evenly and often more quickly.
Convection Heat Transfer in Engineering Applications
Convection heat transfer is foundational in engineering, providing the mechanisms behind crucial technologies and systems. Here are key areas where convection is applied to solve real-world problems:
- Electronics Cooling: Devices such as computers and smartphones rely on convection to dissipate heat and prevent overheating.
- Refrigeration and Air Conditioning: These systems use convective cycles to move heat out of spaces or compartments, maintaining desired temperature levels.
- Industrial Processes: Many manufacturing processes require precise temperature control, achieved through engineered convection heat transfer systems.
- Automotive Engines: Cooling systems in vehicles often utilise convection to maintain optimal operating temperatures for the engine.
A fascinating case study in engineering application of convection is the cooling of large data centres. These critical infrastructures, housing thousands of servers, generate significant amounts of heat. Efficiently managing this heat is vital to prevent system failures and ensure performance. Data centres use a combination of natural and forced convection cooling techniques to achieve this. Air conditioning systems (forced convection) work alongside strategic room layouts that promote natural air movement (natural convection) around server racks. This integration illustrates how engineers harness and optimise convection heat transfer in complex, high-stakes environments.Beyond simply removing heat, the application of convection in engineering often involves nuanced design considerations, such as energy efficiency, noise reduction, and maximising heat transfer rates. As technology advances, the role of convection in thermal management continues to evolve, reflecting the innovation and adaptability inherent in the field of engineering.
The Convective Heat Transfer Coefficient of Air
The convective heat transfer coefficient of air is a key parameter in the engineering and scientific study of heat transfer processes. This coefficient measures the effectiveness of air in transferring heat through convection, which involves the movement of air as it heats up and cools down. Understanding how to calculate this coefficient and the factors that affect its value is crucial for designing efficient HVAC systems, cooling electronic devices, and optimising industrial processes.This section explores the methodology for calculating the convective heat transfer coefficient of air and delves into the various factors that influence its magnitude. This understanding is essential for engineers and scientists who work with systems where air is the primary medium for heat transfer.
Calculating the Convective Heat Transfer Coefficient of Air
Calculating the convective heat transfer coefficient of air requires understanding the conditions under which heat transfer occurs, including the properties of air at the relevant temperature and pressure, the flow characteristics, and the surface over which air is moving. The basic formula for calculating the convective heat transfer coefficient (h) is derived from Newton's law of cooling and is given by:h = Q / (A * \( Delta T\)),where:
- Q is the rate of heat transfer (Watts),
- A is the surface area (m^2),
- \( Delta T\) is the temperature difference between the surface and the air (°C).
For instance, in designing a cooling system for a computer processor, engineers must calculate the convective heat transfer coefficient to determine how effectively air, possibly moved by fans, can cool the processor. If the processor generates 100 Watts of heat, and there's a surface area of 0.01 m^2 with a 50°C temperature difference between the processor surface and the air, the convective heat transfer coefficient can be calculated using the formula provided.
The value of the convective heat transfer coefficient of air can vary widely, depending on factors such as temperature, pressure, and airflow characteristics. This variability underscores the importance of accurate measurement and calculation in design and optimisation tasks.
Factors Affecting the Convective Heat Transfer Coefficient
The convective heat transfer coefficient of air is not a constant; it is influenced by several factors relating to the air properties, flow conditions, and the physical characteristics of the surface. Recognising and understanding these factors are essential for accurate calculation and effective utilisation of this coefficient in thermal management applications.Key factors include:
- Temperature and Pressure: As air temperature and pressure change, its density and viscosity also change, affecting the convective heat transfer process.
- Flow Type: Laminar flow (smooth, with parallel layers) usually results in lower convective heat transfer coefficients than turbulent flow (chaotic, with mixing layers).
- Surface Texture: A rough surface can promote turbulent flow, enhancing the convective heat transfer coefficient compared to a smooth surface.
- Flow Velocity: Increased airflow over a surface generally increases the convective heat transfer coefficient, as it enhances the amount of air interacting with the surface per unit time.
- Convection Heat Transfer: Process of thermal energy movement between an area of higher temperature and a lower one via fluid movement.
- Convective Heat Transfer Coefficient (h): A measure of the heat transfer efficiency between a surface and fluid, influenced by fluid properties, flow velocity, and surface texture.
- Natural Convection: Heat transfer driven by internal temperature differences within the fluid, causing fluid movement without external forces.
- Forced Convection: Heat transfer enhanced by external forces, such as fans or pumps, to accelerate fluid movement over a surface.
- Heat Transfer Formula for Convection: Expressed as Q = h * A * (Ts - Tf), where Q is the rate of heat transfer, A is surface area, Ts is the surface temperature, and Tf is the fluid temperature.
The complex interplay between these factors makes predicting the convective heat transfer coefficient of air challenging yet fascinating. For example, enhancing airflow may lead to a more turbulent regime, significantly impacting heat transfer rates. This is crucial in the cooling of electronic components where space and noise constraints limit the use of large fans. Engineers often use computational fluid dynamics (CFD) simulations to accurately predict how changes in design or operating conditions affect the convective heat transfer coefficient, enabling them to optimise systems for both efficiency and reliability.Moreover, empirical correlations—mathematically derived relationships based on experimental data—are extensively used to estimate the convective heat transfer coefficient under various conditions. These correlations often require inputs like the Reynolds number, which characterises the flow regime, and the Prandtl number, relating to the properties of air. Through careful application of these principles, engineers can design systems that maintain optimal temperatures, ensuring the longevity and performance of critical components.
Convection Heat Transfer - Key takeaways
Learn faster with the 12 flashcards about Convection Heat Transfer
Sign up for free to gain access to all our flashcards.
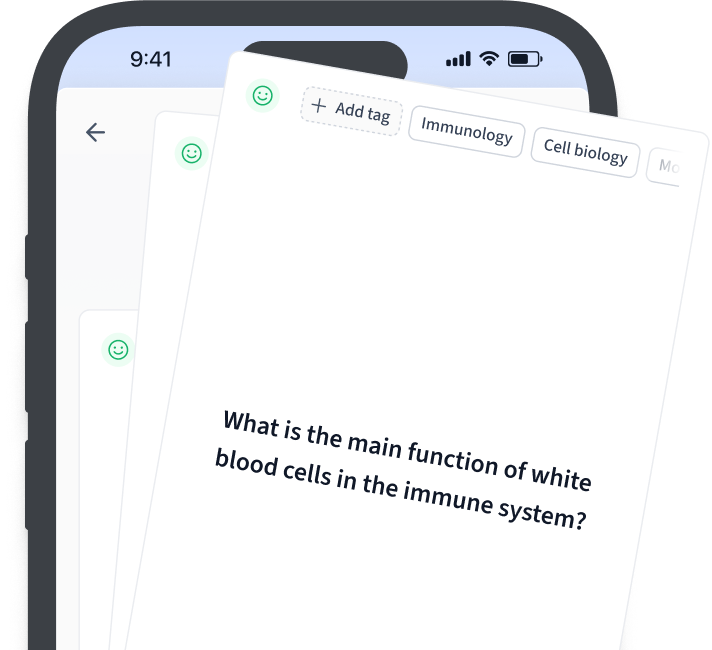
Frequently Asked Questions about Convection Heat Transfer
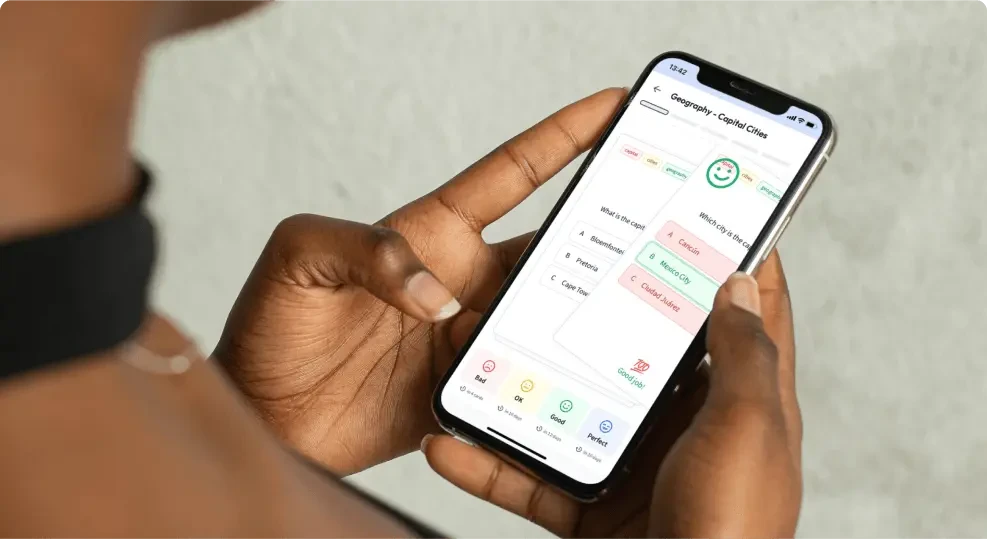
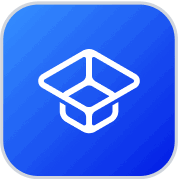
About StudySmarter
StudySmarter is a globally recognized educational technology company, offering a holistic learning platform designed for students of all ages and educational levels. Our platform provides learning support for a wide range of subjects, including STEM, Social Sciences, and Languages and also helps students to successfully master various tests and exams worldwide, such as GCSE, A Level, SAT, ACT, Abitur, and more. We offer an extensive library of learning materials, including interactive flashcards, comprehensive textbook solutions, and detailed explanations. The cutting-edge technology and tools we provide help students create their own learning materials. StudySmarter’s content is not only expert-verified but also regularly updated to ensure accuracy and relevance.
Learn more