Jump to a key chapter
Understanding Flight Regime
Flight regime refers to the different conditions under which an aircraft operates, from take-off to high-altitude cruise, and finally to landing. Understanding these regimes is crucial for engineers and pilots to ensure aircraft performance and safety.
Definition of Regimes of Flight
Flight Regime: The distinct stages of flight which an aircraft passes through, including take-off, ascent, cruise, descent, and landing. Each stage has unique aerodynamic, mechanical, and operational characteristics.
Aircraft flight is categorised into various regimes that highlight the phase of flight and the corresponding conditions that influence aerodynamic forces and flight characteristics. These regimes encompass the complete journey of an aircraft, defining specific flight conditions such as speed, altitude, and engine operation.For instance, during take-off and ascent, aircraft face increasing air density and varying aerodynamic forces. Conversely, during high-altitude cruise, the aircraft operates in thinner air, affecting lift, drag, and engine performance.
Example of Flight Regime: Consider a commercial airliner's journey from one city to another. The take-off regime involves high engine power and lift generation to overcome gravity and achieve flight. During ascent, the aeroplane adjusts its angle and throttle to reach cruising altitude efficiently. At cruise, it maintains a steady altitude, speed, and often, the highest efficiency regarding fuel consumption. Descent and landing regimes require reducing altitude and speed safely to approach and touch down on the runway.
Aircraft designers and pilots pay close attention to each flight regime to maximise performance and safety. Understanding how variables like air density and temperature affect flight helps in designing more reliable and efficient planes.
The concept of Mach number is pivotal in discussing flight regimes, especially when moving from subsonic to supersonic speeds. The Mach number represents the ratio of the aircraft's speed to the speed of sound. At different altitudes, the speed of sound varies due to temperature differences, which in turn affects the Mach number at which an aircraft is flying. For instance, breaking the sound barrier (Mach 1) is a critical event, necessitating special design considerations for aircraft intending to operate in such conditions. This aspect of flight regimes underscores the complexity of aircraft performance and the importance of precision engineering.
Flight Regimes Explained
Flight regimes categorise the speed and operational environments of an aircraft into distinctive phases. These regimes are essential for understanding the aerodynamic principles that dictate aircraft design and performance.By dividing the flight path into segments based on speed, engineers and pilots can better prepare for and manage the different challenges associated with each regime.
Flight Regimes Subsonic
Subsonic Flight Regime: This regime encompasses speeds below the speed of sound (Mach 1), typically up to Mach 0.8. It is characterised by smooth airflow over the aircraft's entire surface.
In the subsonic regime, the aerodynamics are mostly predictable, allowing for stable and efficient flight. Aircraft wings and airframes are designed to optimise performance at these speeds, which include the majority of commercial and general aviation flights.Achieving high efficiency in fuel consumption and noise reduction is a primary focus within this regime, influencing wing shapes, engine types, and other design factors.
Example: Most commercial airliners operate in the subsonic flight regime during long-haul flights. For example, a Boeing 737 or an Airbus A320 typically cruises at speeds just below the sound barrier, ensuring optimal fuel efficiency and passenger comfort.
Though travel in the subsonic regime is slower compared to supersonic flights, it remains the mainstay of commercial aviation due to its efficiency and lower costs.
Transonic Flight Regime
Transonic Flight Regime: This regime occurs at speeds approximately between Mach 0.8 and Mach 1.2. Transitioning through this phase, airflow around the aircraft varies from subsonic to supersonic, leading to complex aerodynamic phenomena such as shock waves.
The transonic regime is notable for the appearance of shock waves on the aircraft, which can cause sudden changes in air pressure and temperature. These effects challenge aircraft stability and control, making design considerations crucial. Engineers focus on designing swept-back wings and specialised airframes to mitigate adverse transonic effects.Operating within this regime requires precise control over speed and altitude, particularly for military jets or commercial airliners that approach the speed of sound.
Example: Fighter jets commonly operate in the transonic regime during combat manoeuvres or when transitioning between subsonic and supersonic speeds. This operation requires aircraft structures capable of handling abrupt changes in aerodynamic forces.
Supersonic and Hypersonic Flight Regime
Supersonic Flight Regime: Occurs at speeds greater than the speed of sound (Mach 1) to Mach 5. Hypersonic Flight Regime: Encompasses speeds beyond Mach 5, where air molecules behave differently, affecting aerodynamics significantly.
The supersonic regime, extending to Mach 5, is marked by a significant increase in aerodynamic heating, air pressure, and resistance. Aircraft in this regime, such as military fighters and reconnaissance aircraft, have sharp edges and thin wings to reduce drag and manage the intense heat generated.The hypersonic regime, beyond Mach 5, is an area of intense interest for military and space applications. Vehicles operating at hypersonic speeds face extreme temperatures and require materials that can withstand severe thermal stress. Research in this area aims at developing new technologies for future air and space travel.
Example: The SR-71 Blackbird, a United States Air Force reconnaissance aircraft, operated at supersonic speeds, allowing it to outrun threats. Meanwhile, space shuttles re-entering Earth's atmosphere experience hypersonic speeds, demanding heat shields to protect against extreme temperatures.
Supersonic and hypersonic flights represent the frontier of aerospace engineering, challenging current understanding and technology with the promise of revolutionising travel and military capabilities.
The advancement in materials science plays a critical role in enabling supersonic and hypersonic flight. Materials like titanium alloys in the SR-71 Blackbird and ceramic-based composites on space shuttle heat shields are examples of engineering innovations designed to endure the harsh conditions of high-speed flight. Looking ahead, the development of scramjets (supersonic combustion ramjets) promises to push the boundaries of hypersonic travel further, potentially transforming aerial combat and reducing travel times across the globe significantly.
Aerodynamics in Different Flight Regimes
Exploring the realm of aerodynamics within different flight regimes reveals how an aircraft's ability to generate lift, control, and overall performance seamlessly adapt to the changing conditions of the atmosphere. From the low-speed manoeuvres just after take-off to the high-speed, high-altitude cruise phase, the principles of aerodynamics guide the aircraft's design and operational strategies.Understanding how aerodynamics vary across these regimes not only enhances the safety and efficiency of flight operations but also drives innovation in aerospace engineering, leading to more advanced and capable aircraft.
How Aerodynamics Vary Across Flight Regimes
In the subsonic regime, where the majority of commercial aircraft operate, aerodynamic efficiency is vital for fuel economy and noise reduction. The flow of air around the aircraft is smooth and predictable, with aerofoil shapes optimised to reduce drag and enhance lift.Transitioning into the transonic regime, aerodynamics become more complex. As aircraft approach the speed of sound, shock waves form, leading to increased drag and potentially unstable flight conditions. Advanced aerodynamic design, such as swept-wing or delta-wing configurations, helps to mitigate these challenges.The supersonic regime, beyond the speed of sound, introduces even more aerodynamic phenomena. Drag increases significantly, and the aircraft experiences changes in temperature and pressure that affect its structural integrity. Designing for supersonic flight requires materials and shapes that can withstand these extreme conditions.Hypersonic flight, typically above Mach 5, pushes the boundaries of aerodynamics and materials science. Airflows around the aircraft become so hot and fast that traditional methods of measuring and mitigating drag and heat are no longer adequate. This regime is the forefront of current aerospace research, aiming to uncover new materials and cooling techniques to protect the aircraft and its passengers.
Aerodynamics: The study of the properties of moving air and the interaction between the air and solid objects moving through it. This scientific discipline is crucial for understanding and improving aircraft performance across different flight regimes.
Example: Consider a commercial jet transitioning from take-off (subsonic) to cruising altitude (near transonic). Initially, the aircraft experiences relatively low speeds and high air density, optimising for lift over drag. As the aircraft ascends and accelerates, it must contend with reducing air density and the onset of shock waves, requiring adjustments in thrust and wing configuration to maintain efficient flight.
The boundary between subsonic and supersonic flight, known as the sonic barrier, was once thought to be insurmountable. Today, it stands as a testament to human ingenuity in aerospace engineering, with aircraft capable of safely and efficiently navigating through various flight regimes.
One fascinating aspect of transonic and supersonic flight is the phenomenon of shock waves. When an aircraft travels at the speed of sound, the air particles in front of the aircraft cannot move out of the way quickly enough, creating a steep pressure gradient. This can be seen in the famous images of supersonic jets with visible shock waves, illustrating the incredible force and energy involved in breaking the sound barrier.The understanding and management of these shock waves are critical for the safe operation of supersonic aircraft, influencing the design of everything from passenger windows to wing shapes. Innovations in computational fluid dynamics (CFD) have enabled engineers to simulate and study these effects in detail, paving the way for the next generation of supersonic and hypersonic aircraft.
The Importance of Recognising Flight Regimes
Recognising flight regimes is foundational in aerospace engineering, serving as a critical aspect in the development, testing, and operation of aircraft. Different flight regimes pose unique challenges and opportunities for engineers, requiring tailored approaches in aircraft design, performance optimisation, and safety measures.From subsonic to hypersonic speeds, the physical principles governing flight vary significantly. This variability affects everything from aerodynamic efficiency to structural integrity, making the identification and understanding of flight regimes a cornerstone of aerospace innovation.
Significance in Aerospace Engineering
In aerospace engineering, the significance of recognising flight regimes extends beyond theoretical understanding. It influences practical design decisions, safety protocols, and operational strategies. Each regime encompasses distinct physical phenomena that must be accounted for to ensure optimal aircraft performance and safety.
Flight Regime: A flight regime is categorised by the aircraft's speed relative to the speed of sound, defining unique aerodynamic conditions and operational challenges encountered during flight.
Understanding these regimes allows engineers to:
- Design aircraft structures and components that can withstand varying aerodynamic forces
- Develop propulsion systems suited for specific speed ranges
- Create control systems that maintain stability and manoeuvrability across different flight phases
- Optimise fuel efficiency and minimise environmental impact
Example: The design of the Concorde, the iconic supersonic passenger jet, illustrates the importance of recognising flight regimes in aerospace engineering. Engineers had to solve complex challenges associated with supersonic flight, such as intense aerodynamic heating, increased drag, and noise pollution. This led to innovations such as the delta wing, which provided the necessary lift and reduced drag at high speeds, and specialised materials to withstand high temperatures.
Flight regime recognition is not solely the domain of aircraft designers. Pilots also rely on an understanding of different flight regimes to make informed decisions during manual control and to utilise automated systems effectively.
The transition from subsonic to supersonic flight was a monumental achievement in aerospace engineering. This transition involves passing through the sound barrier, a once-daunting challenge that required innovations in aircraft design, material science, and propulsion. Exploring the sound barrier transition illustrates the adaptive nature of aerospace engineering in overcoming physical limits.Developments in transonic aerodynamics, such as area ruling and swept wings, helped reduce the effects of shock waves and drag, enabling aircraft like the F-104 Starfighter to achieve and maintain supersonic speeds. These achievements underscore the importance of understanding flight regimes, as they directly influence the capabilities and performance of aircraft.
Flight Regime - Key takeaways
- Flight Regime: Categories of aircraft operation characterized by specific conditions such as speed, altitude, and engine performance, encompassing take-off, ascent, cruise, descent, and landing phases.
- Subsonic Flight Regime: Includes speeds below the speed of sound (Mach 1), up to Mach 0.8, where airflow over the aircraft is smooth and aerodynamic forces are predictable.
- Transonic Flight Regime: Covers speeds approximately between Mach 0.8 and Mach 1.2, marked by changing airflow from subsonic to supersonic, leading to complex aerodynamic phenomena such as shock waves.
- Supersonic and Hypersonic Flight Regime: Supersonic extends from speeds over Mach 1 to Mach 5, and hypersonic is beyond Mach 5, both involving extreme aerodynamics and temperature conditions requiring advanced materials and designs.
- Aerodynamics: The study of air movement and its interaction with solid objects, essential for aircraft performance across different flight regimes, guiding design and operational strategies.
Learn faster with the 12 flashcards about Flight Regime
Sign up for free to gain access to all our flashcards.
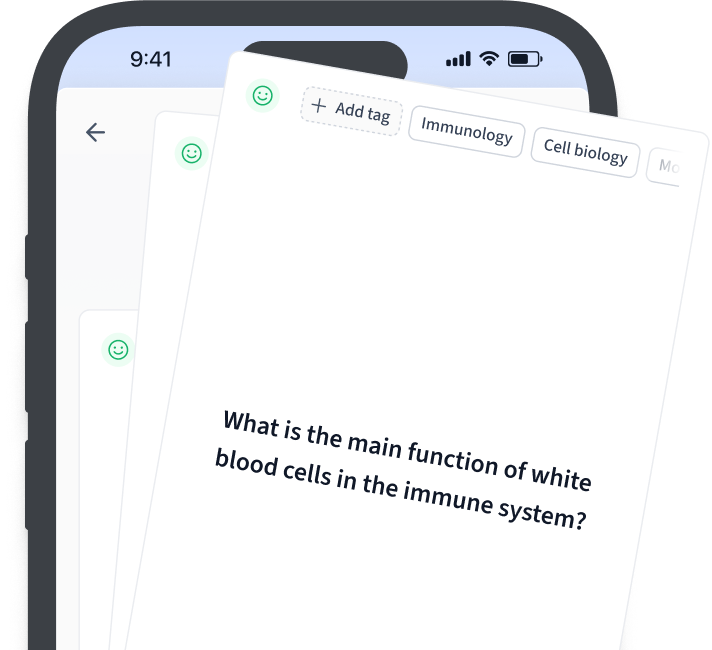
Frequently Asked Questions about Flight Regime
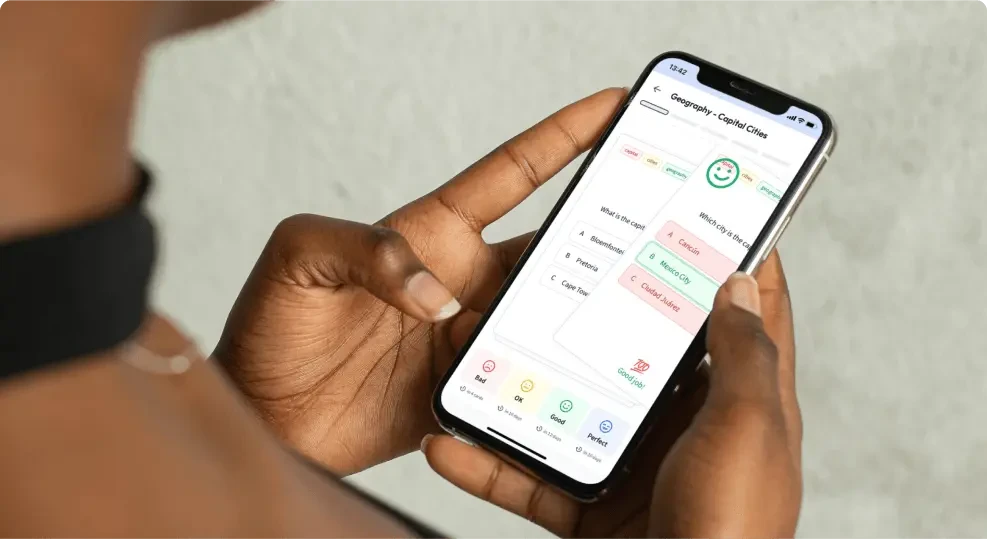
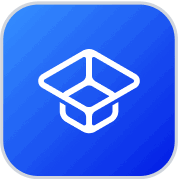
About StudySmarter
StudySmarter is a globally recognized educational technology company, offering a holistic learning platform designed for students of all ages and educational levels. Our platform provides learning support for a wide range of subjects, including STEM, Social Sciences, and Languages and also helps students to successfully master various tests and exams worldwide, such as GCSE, A Level, SAT, ACT, Abitur, and more. We offer an extensive library of learning materials, including interactive flashcards, comprehensive textbook solutions, and detailed explanations. The cutting-edge technology and tools we provide help students create their own learning materials. StudySmarter’s content is not only expert-verified but also regularly updated to ensure accuracy and relevance.
Learn more