Jump to a key chapter
Understanding Glider Aerodynamics
Exploring the world of glider aerodynamics opens up a fascinating understanding of how these engineless aircraft soar through the skies. By delving into the essentials of aerodynamic principles, one begins to appreciate the intricate balance and design considerations that allow gliders to glide.
The Basics of Glider Aircraft Aerodynamics
Glider aircraft, also known as sailplanes, are designed to fly without the use of engines. The core principles of aerodynamics that govern glider flight are lift, drag, weight, and thrust. Even without engines, gliders can achieve and sustain flight by exploiting these forces effectively. The aim is to maximise lift, minimise drag, and use the weight of the glider to generate forward motion through the air. Gliders are equipped with long wings, which provide a high aspect ratio that is crucial for efficient lift. These aircraft rely on updrafts of air, such as thermals, ridge lift, or wave lift, to gain altitude without the need for engine power.
Lift: The aerodynamic force that opposes the weight of the glider and keeps it airborne. It is generated by the movement of the glider through the air and its interaction with the wings.
Lift is created by the difference in air pressure on the top and bottom surfaces of the wings. This difference is a result of the airfoil shape of the wings, designed specifically to increase air velocity over the top surface, thereby reducing the pressure above the wing compared to below it, according to Bernoulli's principle. Efficient lift is crucial for gliders as it determines how well the glider can make use of rising air currents to stay aloft without engine power.
How the Aerodynamics of a Glider Determines its Flight
The flight dynamics of a glider are intricately tied to its aerodynamic properties. Directional stability, control, and performance in varying atmospheric conditions are all determined by how well a glider can manage aerodynamic forces. Pilots adjust the glider's attitude in relation to the oncoming air (pitch, roll, and yaw) using control surfaces like ailerons, elevators, and rudders. These adjustments help the glider to optimise its flight path, maintain altitude, or to ascend through exploiting atmospheric updrafts. The ability of a glider to stay airborne and navigate through the air efficiently depends largely on its lift-to-drag ratio. A higher ratio means that the glider can travel further forward for every unit of vertical drop, enhancing its glide range.
Lift-to-Drag Ratio: A measure of the efficiency of an aircraft's aerodynamic design, indicating how much lift the aircraft generates compared to its drag. A higher ratio is indicative of greater aerodynamic efficiency.
For instance, if a glider has a lift-to-drag ratio of 40:1, it means that for every 1 metre of descent, the glider can travel forward 40 metres, assuming ideal conditions. This efficiency enables gliders to make long flights without engine power.
The Impact of Design on Glider Aerodynamics
Design elements of a glider, such as wing shape, size, and the overall aerodynamics profile, play a pivotal role in its performance. Innovations in materials and aerodynamic theory have led to the development of gliders with highly sophisticated designs capable of remarkable feats of flight. Engineers must carefully consider the trade-offs between lift, drag, and weight during the design process. Wings are shaped and angled to maximise lift while minimising drag. The surface area of the wings also affects the glider's ability to catch thermals. By optimising these design features, engineers can enhance the performance, stability, and efficiency of gliders.
Designers often use computer simulations and wind tunnel testing to refine the aerodynamics of gliders, allowing for precise adjustments to the wing shape and other structures to achieve the desired flight characteristics.
The Fundamentals of Glider Design Theory
Diving into the fundamentals of glider design theory is a journey into the heart of aerodynamics tailored specifically for engineless flight. Understanding these principles is essential for designing gliders that optimise performance, efficiency, and safety.
Key Principles of Glider Design Theory
Glider design is anchored in mastering the balance between lift, drag, weight, and thrust—a puzzle without the assistance of engines. At the heart of this challenge is creating a design that maximises lift over drag, leveraging air currents and thermal lifts to glide through the sky effectively. Key components of glider design include the shape, size, and aspect ratio of the wings, which are critical in determining the aircraft's performance. Gliders favour long, slender wings to improve lift and reduce drag, enabling longer and more efficient flights.
Aspect Ratio: In the context of aerodynamics, the aspect ratio refers to the comparison between the length and width of the wings. A high aspect ratio, characterised by longer and narrower wings, is preferred in gliders for its efficiency in generating lift.
The concept of airfoil design is also essential in glider design theory. An airfoil's shape affects the airflow over the wing, influencing the lift and drag characteristics. Gliders utilise highly efficient airfoils to maximise lift and minimise drag, allowing them to soar effortlessly through the air. The choice of airfoil plays a crucial role in determining the glider's performance in different flight conditions.
Glider Lift to Drag Ratio Explained
The lift to drag ratio is a critical metric in aerodynamics, especially for gliders, where engine power is not an option. This ratio measures the efficiency of the glider's ability to convert downward motion into forward movement. A higher ratio signifies greater aerodynamic efficiency, allowing for longer flights with less descent. The ideal ratio varies depending on the glider's design and its intended use. Racing gliders, for example, might prioritise a higher lift to drag ratio for speed, while leisure gliders might focus on stability and ease of control.
To illustrate, a glider with a lift to drag ratio of 50:1 can travel 50 metres forward for every metre it descends. Achieving such efficiency is the result of meticulous design, focusing on wing aspect ratio, airfoil selection, and minimising unnecessary drag.
Innovations in Glider Design for Optimal Aerodynamics
The landscape of glider design has evolved significantly, with innovations focusing on enhancing aerodynamic efficiency. Advanced materials like carbon fibre composites have led to lighter, stronger structures that can withstand the demands of flight while minimising weight and drag. Cutting-edge technology in computational fluid dynamics (CFD) and aerodynamic modelling has further refined our understanding and capabilities in glider design. These tools allow designers to simulate airflow and optimise designs before physical prototypes are ever tested.
Modern gliders often incorporate winglets at the tips of the wings to reduce induced drag, significantly improving the lift to drag ratio and overall performance.
Exploring Hang Glider Aerodynamics
Hang glider aerodynamics represents a captivating intersection of physics, engineering, and the pure joy of flight. Understanding these principles provides a deeper appreciation for the elegance and efficiency of hang gliders and their operation.
The Physics Behind Hang Glider Aerodynamics
Hang gliders employ basic aerodynamic principles to achieve flight. Lift, the upward force that counteracts gravity, is generated as air flows over and under the wing's surface. The design of the wing, specifically its shape and angle of attack, plays a crucial role in this process. Drag is another key factor affecting hang gliders. It is the resistance the glider faces as it moves through the air. Pilots must navigate these forces, using thermal currents and wind gradients to stay aloft, demonstrating a practical application of aerodynamics.
Angle of Attack: The angle at which the wing meets the oncoming air flow. It is a critical factor in generating lift.
Understanding how lift is created is fundamental. As air flows over the wing, its speed increases over the top surface while pressure decreases, according to Bernoulli's principle. Simultaneously, the air pressure beneath the wing remains higher, pushing the wing up into the lower pressure zone. Manipulating the angle of attack can significantly affect the amount of lift generated, enabling the glider to rise or descend.
Hang gliders often adjust their centre of gravity to change the angle of attack, rather than moving the wings themselves.
Design Features that Enhance Hang Glider Performance
The performance of hang gliders is heavily influenced by their design. From the shape and size of the wings to the materials used in construction, every aspect is optimised for maximum aerodynamic efficiency. Key features include:
- High aspect ratio wings that reduce drag and improve lift.
- Aerodynamically efficient shapes that streamline the glider.
- Lightweight materials like carbon fibre and aluminium for strength without the weight.
An example of design optimisation can be seen in the winglets found on some hang gliders. These small, upward-curving surfaces at the wing tips help to reduce vortex drag, which occurs when air vortices form at the wing tips due to high-pressure air from beneath the wing mixing with low-pressure air above. By mitigating this drag, winglets improve the overall lift-to-drag ratio of the glider.
Safety Considerations in Hang Glider Aerodynamics
Safety in hang gliding is paramount, and aerodynamics play a significant role in ensuring that pilots can fly and land safely. The stability of the glider, its response to control inputs, and its ability to recover from adverse conditions are all influenced by its design. Modern hang gliders are designed to be inherently stable during flight, but pilots must be skilled in managing the glider's energy and momentum, especially during takeoff and landing phases. Safety features might include:
- Easy handling characteristics to ensure pilots can effectively control the glider.
- Emergency parachute systems designed to deploy in case of structural failure or loss of control.
- Aerodynamic stall resistance to prevent loss of control at low speeds.
Aerodynamic Performance of Hand Launch Glider
The aerodynamic performance of hand launch gliders is a critical aspect of their design and operation. By understanding and applying the principles of aerodynamics, designers can optimise gliders for maximum efficiency, allowing them to glide further and with more stability.
Designing for Performance: Hand Launch Glider Dynamics
When designing a hand launch glider, several aerodynamic factors come into play. The goal is to create a design that offers the best possible performance by balancing lift, drag, and weight. Key elements include the shape of the wings, the weight distribution, and the glider's overall structure. Effective design strategies focus on:
- Maximising lift-to-drag ratio to enhance glide distance and efficiency.
- Optimising wing loading to balance the lift generated against the weight of the glider.
- Refining the shape and orientation of the wings to ensure stability and control.
Further to wing design, understanding the Reynolds number, which represents the ratio of inertial forces to viscous forces within the flow around the glider, is critical. For hand launch gliders, operating at lower Reynolds numbers due to their smaller size and lower velocity compared to other aircraft, designers must carefully select airfoil shapes that remain efficient under these conditions.
Analysing the Efficiency of Hand Launch Gliders
Efficiency in hand launch gliders is often measured by their lift-to-drag ratio, a fundamental metric in aerodynamics. This ratio illustrates how effectively a glider can convert lift—generated as it moves through the air—into forward motion, against the drag it experiences. Analysis involves:
Lift-to-Drag Ratio | Interprets aerodynamic efficiency, seeking values that indicate a glider's ability to sustain flight over longer distances. |
Flight Duration | Ongoing monitoring of flight times under various conditions to assess performance. |
Simulation software can offer valuable insights during the design phase, allowing for aerodynamic analysis without the need for extensive physical prototypes.
Materials and Technologies Improving Hand Launch Glider Aerodynamics
Advances in materials and technology have a profound impact on the aerodynamics of hand launch gliders. By utilising lighter and stronger materials, designers can refine aerodynamic shapes, reduce weight, and consequently, improve efficiency. Innovations include:
- High-tech composites such as carbon fibre and Kevlar, offering superior strength-to-weight ratios.
- Micro-electromechanical systems (MEMS), including gyroscopes and accelerometers, enhancing stability and control.
- Aerodynamic software tools for precise modelling and simulation, enabling the optimisation of designs before construction.
Glider Aerodynamics - Key takeaways
- Glider Aircraft Aerodynamics: Gliders, also known as sailplanes, utilise aerodynamic forces—such as lift, drag, weight, and thrust—to fly without engines. They achieve and sustain flight by exploiting updrafts to gain altitude, requiring a high aspect ratio for efficient lift.
- Lift: An aerodynamic force opposing the weight of the glider, generated by airflow interaction with wing design, according to Bernoulli's principle, and essential for engineless flight.
- Lift-to-Drag Ratio: A measure of the aerodynamic efficiency of an aircraft that indicates how much lift is produced relative to drag; crucial for determining a glider's glide range and performance.
- Glider Design Theory: Focuses on balancing lift, drag, weight, and thrust without engines, utilising long, slender wings and efficient airfoils to optimise lift over drag and leverage air currents for gliding.
- Hang Glider Aerodynamics: Involves fundamental principles such as lift generation via wing shape and angle, the importance of drag reduction, and design elements like winglets to increase efficiency and safety.
Learn faster with the 12 flashcards about Glider Aerodynamics
Sign up for free to gain access to all our flashcards.
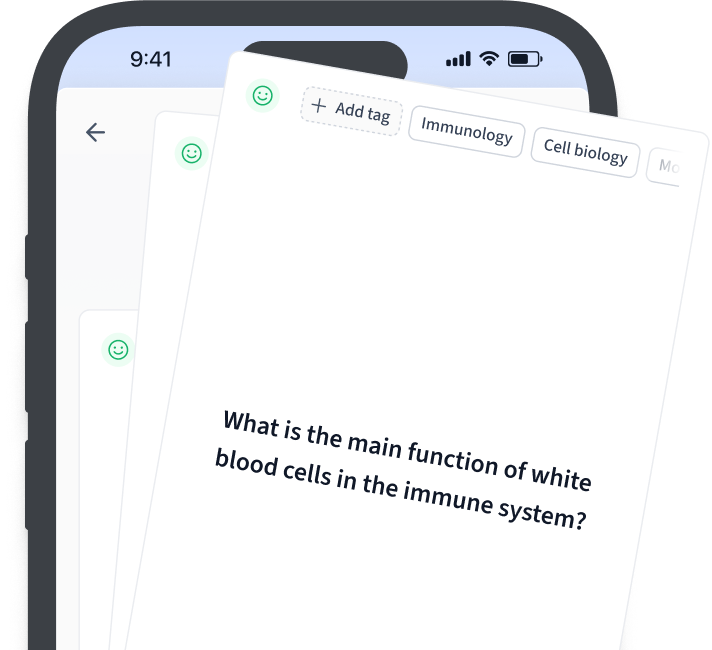
Frequently Asked Questions about Glider Aerodynamics
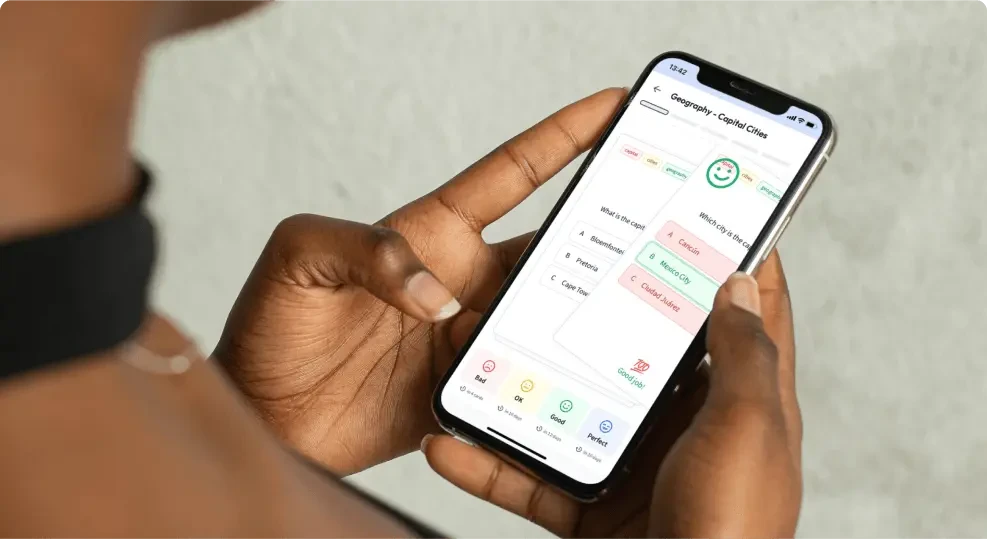
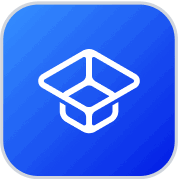
About StudySmarter
StudySmarter is a globally recognized educational technology company, offering a holistic learning platform designed for students of all ages and educational levels. Our platform provides learning support for a wide range of subjects, including STEM, Social Sciences, and Languages and also helps students to successfully master various tests and exams worldwide, such as GCSE, A Level, SAT, ACT, Abitur, and more. We offer an extensive library of learning materials, including interactive flashcards, comprehensive textbook solutions, and detailed explanations. The cutting-edge technology and tools we provide help students create their own learning materials. StudySmarter’s content is not only expert-verified but also regularly updated to ensure accuracy and relevance.
Learn more