Jump to a key chapter
Understanding Heat Exchangers in Aerospace Engineering
In the vast and technical world of aerospace engineering, heat exchangers play a pivotal role in managing the temperature of various systems onboard aircraft and spacecraft. These components are crucial for ensuring the machinery operates within safe temperature limits. Let's delve into the nuances of heat exchangers, their types, and how they are optimised for efficiency and performance within the aerospace industry.
What is a Heat Exchanger?
A heat exchanger is a device used to transfer heat between two or more fluids without them mixing. In aerospace, it allows for thermal energy transfer from one part of the aircraft or spacecraft to another, managing the internal and external temperature variations encountered during flights.
For instance, in high-speed jets, heat exchangers might cool the oil circulating through the engine using the colder external air, thus preventing the engine from overheating.
Types of Heat Exchangers in Aerospace
Aerospace engineering leverages various types of heat exchangers, each selected based on the specific requirements of the aircraft or spacecraft. Here are some common types:
- Shell and Tube Heat Exchangers: Used for their robust design, making them suitable for high-pressure applications.
- Plate Heat Exchangers: Known for their efficiency and compact size, ideal for space-limited applications.
- Fin Tube Heat Exchangers: Optimised for air-cooling processes, particularly useful in the cooling of avionics.
- Regenerative Heat Exchangers: These allow for a very efficient heat transfer by temporarily storing heat before transferring it, useful in applications where the same fluid circulates in a loop.
The choice of heat exchanger depends on factors like temperature, pressure, fluid types, and spatial constraints within the aircraft or spacecraft.
Heat Exchanger Efficiency and Performance
Efficiency and performance of heat exchangers in aerospace are critical, as they directly affect the vehicle's overall energy efficiency, safety, and reliability. Let’s break down how performance is measured and enhanced:
The efficiency of a heat exchanger refers to its ability to transfer heat from one fluid to another with minimal energy loss. Performance, on the other hand, encompasses the heat exchanger's overall effectiveness, reliability, and the balance between its cooling or heating capabilities and operational costs.
Key factors influencing the efficiency and performance include:
- Material: The thermal conductivity of the material affects heat transfer rates.
- Design: The design determines the flow patterns of the fluids, affecting heat transfer efficiency.
Surface Area: Larger surface areas promote better heat exchange. - Maintenance: Regular cleaning and inspection ensure optimal performance and prevent failures.
Among aerospace applications, improving the efficiency of heat exchangers can lead to significant advancements in fuel efficiency and emissions reduction. For instance, using advanced materials like graphene for heat exchangers could potentially enhance heat transfer rates far beyond current capabilities, opening new doors for energy efficiency improvements in aerospace and beyond.
Dive into Shell and Tube Heat Exchanger
Shell and tube heat exchangers are a cornerstone in both industrial and commercial applications, including the heating, ventilation, and air conditioning (HVAC) systems. These exchangers are prized for their efficiency, durability, and adaptability. Understanding the design principles behind them and how they are applied in HVAC systems can provide insights into their widespread usage and importance.
Design Principles of Shell and Tube Heat Exchanger
A shell and tube heat exchanger consists of a series of tubes, which one set of fluids runs through, enclosed within a shell, where another fluid flows over the tubes, exchanging heat without direct contact.
The design of shell and tube heat exchangers adheres to several key principles to maximise efficiency and longevity. These include:
- Thermal Design: Determining the size, arrangement, and materials of the tubes to ensure optimal heat transfer.
- Mechanical Design: Constructing the shell, tube sheets, and baffles to withstand pressure differences and prevent leakage.
- Flow Configuration: Arranging the direction of the fluids to either counterflow, parallel flow, or crossflow, affecting the heat exchanger's effectiveness.
Meticulous attention to these principles allows shell and tube heat exchangers to function reliably in diverse conditions, balancing the heat transfer efficiency with the mechanical integrity of the system.
For example, in a counterflow arrangement, the hot and cold fluids flow in opposite directions, allowing for a high temperature differential and therefore high heat transfer efficiency. This design is often preferred for applications requiring significant temperature changes in the fluids.
The choice of materials for the tubes—such as copper or stainless steel—is also crucial, affecting the heat exchanger's resistance to corrosion and its thermal conductivity.
Shell and Tube Heat Exchanger HVAC Applications
Within HVAC systems, shell and tube heat exchangers serve various crucial roles: from chilling water in air conditioning systems to heating spaces through hot water boilers. Their application in HVAC is broad, given their adaptability to different temperatures and pressures, as well as their reliability for both heating and cooling tasks.
Applications include:
- Water chillers, where they transfer heat from water to a refrigerant, cooling buildings.
- Boilers, where they transfer heat from combustion gases to water, heating buildings.
- Heat recovery steam generators, which capture exhaust heat to generate steam for heating or power generation.
The HVAC system (Heating, Ventilation, and Air Conditioning) ensures a building's indoor environment is thermally comfortable by regulating temperature, humidity, and air quality.
Shell and tube heat exchangers are highly efficient, making them ideal for these applications where energy transfer efficiency can directly impact operational costs and environmental footprint. Their ability to handle a variety of fluids and temperatures also allows HVAC systems to operate more smoothly and dependably across different climates and seasonal changes.
An interesting application of shell and tube heat exchangers in HVAC systems is in geothermal heating and cooling, where the exchanger can efficiently transfer heat between the building and the ground or groundwater, leveraging the stable temperatures below the Earth's surface for incredible efficiency benefits.
Exploring Plate Heat Exchangers
Plate heat exchangers represent a compact, efficient solution for thermal management in various settings, notably within industries that demand high-efficiency heat transfer with limited space. The unique construction and operational principles of plate heat exchangers allow them to excel where other types may fall short.
What Makes Plate Heat Exchanger Different?
A plate heat exchanger consists of multiple, thin, corrugated plates stacked together, forming channels for fluids to flow between them. Heat exchange occurs as one fluid moves along the odd-numbered channels while the other moves along the even-numbered channels, allowing for heat transfer through the plates.
The distinction of plate heat exchangers lies in their design and efficiency:
- Compact Structure: Their thin plate design occupies less space compared to shell and tube counterparts.
- High Heat Transfer Efficiency: The corrugated plates and close proximity of the fluids enhance the heat transfer rate.
- Easy Maintenance: The stackable design allows for easy dismantling, cleaning, and expansion.
Additionally, the materials used for the plates, typically metals with high thermal conductivity, further optimise heat transfer rates.
An example of a plate heat exchanger application is in the food and beverage industry, where they are used to cool large quantities of product in a relatively small space. The efficiency of these exchangers ensures that products are quickly cooled to safe temperatures, preserving their quality and safety.
The efficiency of plate heat exchangers also makes them suitable for applications requiring heat recovery, which can significantly reduce energy consumption.
Advantages of Plate Heat Exchanger in Aerospace Engineering
In the rigorous and demanding context of aerospace engineering, plate heat exchangers offer several significant advantages:
- Weight Reduction: Their compact and lightweight design is crucial for aircraft where every kilogram matters for fuel efficiency and performance.
- Thermal Efficiency: Their superior heat transfer capabilities ensure optimal thermal regulation of critical aerospace systems.
- Customisability: The modularity of plate heat exchimators allows for tailored configurations to meet specific aerospace needs.
Aerospace engineering encompasses the design, development, and maintenance of aircraft and spacecraft, demanding the highest standards of performance, including thermal management systems.
One fascinating use of plate heat exchangers in aerospace is within the Environmental Control Systems (ECS) of aircraft. These systems regulate the temperature, pressure, and air composition of the cabin and cockpit. Plate heat exchangers, with their precise temperature control ability, are integral to ECS, maintaining comfortable conditions for passengers and crew while efficiently managing the thermal loads generated by aircraft systems and external environmental conditions.
The adaptability of plate heat exchangers to varying temperatures and pressures also makes them suited for spacecraft, where the external thermal environment can be extreme.
Analysing Plate and Frame Heat Exchanger
Plate and frame heat exchangers are a type of heat exchanger design that offers high efficiency in heat transfer between two fluids. By dissecting their key features and understanding how to calculate their efficiency, one gains insight into their widespread application across various industries.
Key Features of Plate and Frame Heat Exchanger
Several distinctive features make the plate and frame heat exchanger choice for many applications:
- High Thermal Efficiency: The compact design and large surface area of the plates facilitate significant heat transfer in a smaller footprint.
- Low Cost: Relatively inexpensive to manufacture, they offer a cost-effective solution for thermal management needs.
- Modularity: The design allows for easy expansion or reduction in capacity by simply adding or removing plates.
- Easy Maintenance: Their construction enables easy disassembly for cleaning and maintenance, reducing downtime.
This combination of features makes plate and frame heat exchangers particularly suited to industries where space is at a premium, and efficiency is paramount.
Calculating Heat Exchanger Efficiency
The efficiency of a heat exchanger is often measured in terms of its effectiveness, which is the fraction of the maximum possible heat transfer that is actually achieved between the hot and cold fluids.
For example, if a plate and frame heat exchanger transfers 90% of the maximum possible heat from the hot fluid to the cold fluid, its effectiveness would be 0.9 or 90%.
To calculate the efficiency of a plate and frame heat exchanger, one needs to understand the specifics of the thermal operations involved. This includes knowing the temperatures and flow rates of both the hot and cold fluids entering and exiting the exchanger. The formula for calculating effectiveness is:
- Effectiveness (ε) = (Actual Heat Transfer) / (Maximum Possible Heat Transfer)
The actual heat transfer can be found with the specific heat capacity of the fluids and their flow rates, while the maximum possible heat transfer is determined by the temperature difference between the hot and cold fluids at the entrance of the heat exchanger.
Consideration of specific heat capacities of the fluids is crucial, as they directly impact the heat transfer capabilities of the heat exchanger.
When assessing the efficiency of plate and frame heat exchangers, it's also beneficial to consider the impact of fouling on performance. Over time, the deposition of materials on the plates can decrease thermal efficiency. Including a fouling factor in calculations allows for a more accurate estimation of long-term performance and scheduling of maintenance activities to restore optimal efficiency.
Heat Exchangers - Key takeaways
- A heat exchanger is a device that transfers heat between two or more fluids without them mixing, crucial in aerospace for temperature management.
- Common types of heat exchangers in aerospace: Shell and Tube Heat Exchanger (high-pressure robust design), Plate Heat Exchanger (compact and efficient), Fin Tube Heat Exchanger (for air-cooling), and Regenerative Heat Exchanger (efficient for looped fluid systems).
- Heat exchanger efficiency indicates the ability to transfer heat with minimal energy loss, while performance includes effectiveness, reliability, and operational costs balance.
- The Shell and Tube Heat Exchanger is valued in HVAC systems for its durability, adaptability, and efficiency, using design principles like thermal design, mechanical design, and flow configuration.
- Plate and Frame Heat Exchanger offers high thermal efficiency with a smaller footprint, modularity, and easy maintenance, making it suitable for various industries including aerospace.
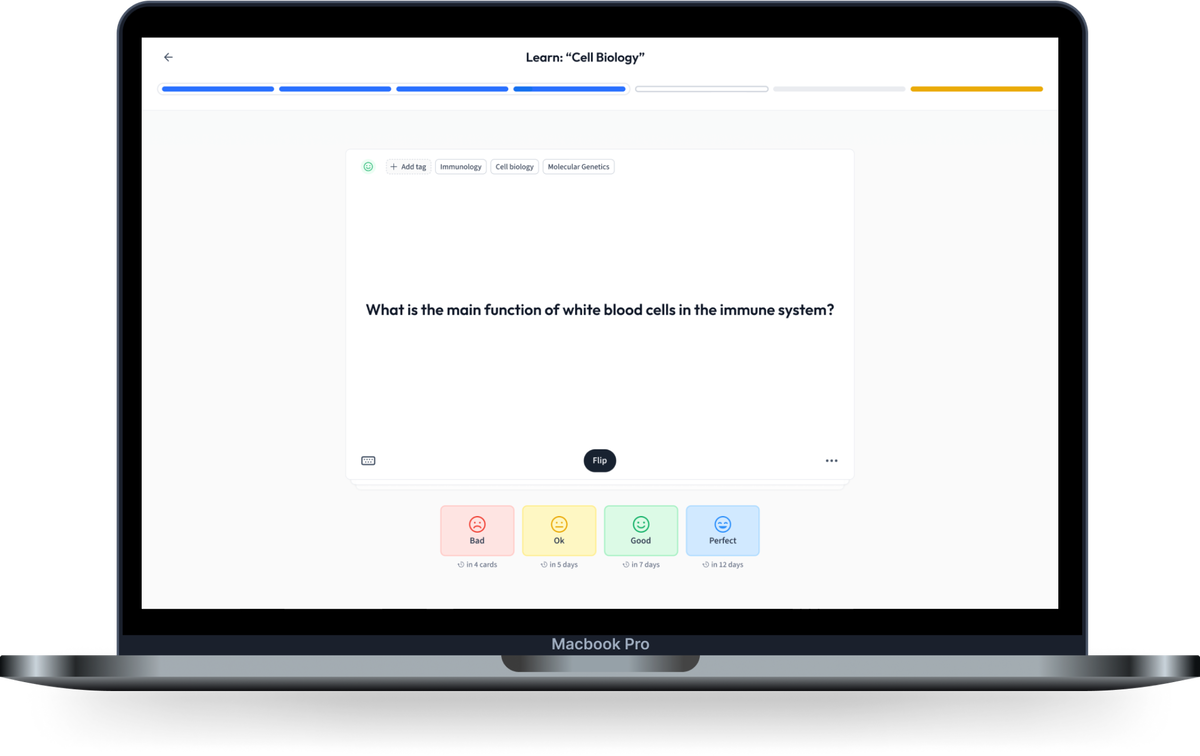
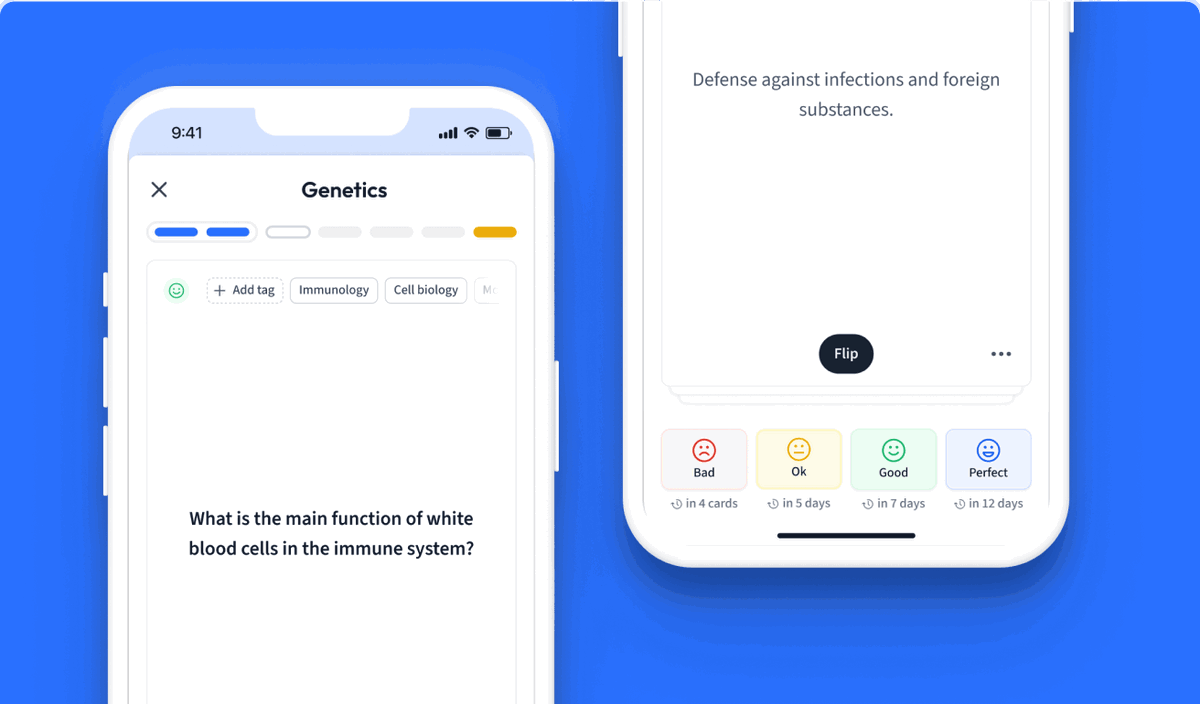
Learn with 12 Heat Exchangers flashcards in the free StudySmarter app
Already have an account? Log in
Frequently Asked Questions about Heat Exchangers
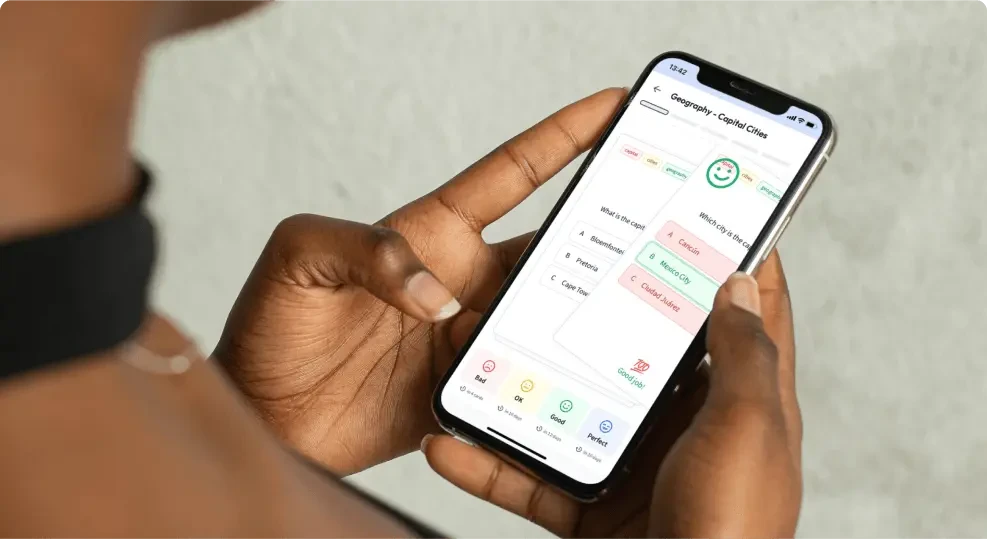
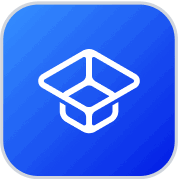
About StudySmarter
StudySmarter is a globally recognized educational technology company, offering a holistic learning platform designed for students of all ages and educational levels. Our platform provides learning support for a wide range of subjects, including STEM, Social Sciences, and Languages and also helps students to successfully master various tests and exams worldwide, such as GCSE, A Level, SAT, ACT, Abitur, and more. We offer an extensive library of learning materials, including interactive flashcards, comprehensive textbook solutions, and detailed explanations. The cutting-edge technology and tools we provide help students create their own learning materials. StudySmarter’s content is not only expert-verified but also regularly updated to ensure accuracy and relevance.
Learn more