Jump to a key chapter
What is Hypervelocity Impact?
Hypervelocity impact refers to the scenario where two objects collide at speeds much greater than the speed of sound in their medium. In the context of engineering, particularly in aerospace, this phenomenon is crucial in understanding and mitigating the risks associated with space debris and micrometeoroids colliding with spacecraft, satellites, and planetary bodies. The outcomes and effects of such collisions are studied to improve the durability and survivability of human-made structures in space.
Defining Hypervelocity Impact in Aerospace
In the aerospace sector, hypervelocity impact is emblematic of speeds exceeding 3 kilometres per second, far surpassing the speed of sound on Earth. Such extreme velocities mean that even small particles can deliver significant energy on impact, posing a substantial risk to spacecraft and satellites.Examples of hypervelocity impacts in aerospace include collisions with space debris, micrometeorites, and other artificial objects travelling at high speeds in low Earth orbit. Understanding these impacts is critical for the design and protection of space vehicles and their components.
The Physics Behind Hypervelocity Impact
The physics of hypervelocity impact revolves around the conversion of kinetic energy into other forms, such as heat and deformation, upon collision. At hypervelocities, materials behave differently compared to low-speed impacts. They may vaporise, melt, or undergo severe plastic deformation.
- Kinetic Energy Conversion: The primary aspect of hypervelocity impact physics, where the kinetic energy of a moving object is transformed upon collision, frequently resulting in extreme temperatures and material phase changes.
- Shock Waves: These are generated by the high-speed collision and propagate through the materials, causing additional internal damage beyond the initial impact site.
- Crater Formation: The impact often results in a crater, whose size and shape are dictated by the speed of the impact and the material properties of both the impactor and the target.
Real-World Examples of Hypervelocity Impact
Hypervelocity impacts are not solely the domain of academic interest or space exploration challenges; they play a significant role in various real-world applications and occurrences.
- Spacecraft Shielding: The International Space Station and other spacecraft are equipped with specially designed shields to protect against hypervelocity impacts from micrometeoroids and space debris. These shields are crafted to absorb and dissipate the energy of the impacting particles, minimising damage.
- Planetary Geology: The surfaces of the Moon, Mars, and other celestial bodies are scarred with craters formed by hypervelocity impacts. Studying these craters gives scientists insight into the history and composition of these planetary bodies.
- Ballistic Testing: On Earth, hypervelocity impacts are simulated to test and improve military armour and spacecraft shielding, employing projectiles fired at targets at high speeds to study the impact effects.
Hypervelocity Impact in Spacecraft Design
Designing spacecraft to withstand hypervelocity impacts is a critical challenge in aerospace engineering. Given the high speeds involved, even small particles of space debris can pose significant threats to operational missions. Engineers employ a variety of strategies and materials to protect spacecraft from these potentially devastating collisions.
Protecting Spacecraft from Hypervelocity Impact
To safeguard spacecraft against hypervelocity impacts, engineering teams integrate advanced materials and innovative designs into the vehicle's structure.
- Whipple Shields: A popular method involves the use of Whipple shields, a protective barrier designed to disperse the energy of impacting particles, reducing their potential for damage.
- Multi-Layer Insulation: Additionally, multi-layer insulation (MLI) can provide both thermal protection and impact resistance, serving a dual purpose in spacecraft design.
- Advanced Materials: The choice of materials is essential, with some spacecraft incorporating carbon fibre, Kevlar, or aluminium alloys for strength and resilience against impacts.
Whipple shields are named after the American astronomer Fred Whipple, who first proposed this design concept in 1947.
ESA Space Debris Studies Hypervelocity Impact Sample
The European Space Agency (ESA) has conducted extensive research into hypervelocity impacts through the Space Debris Studies programme. This research aims to better understand the dynamics and effects of these impacts on spacecraft materials and components.A notable study involved firing tiny projectiles at speeds up to 8 km/s at sample spacecraft materials to simulate space debris collisions. The results offer invaluable insights into how different materials react to hypervelocity impacts, guiding the development of more resilient spacecraft designs.The findings emphasise the importance of ongoing research and innovation in spacecraft design and materials science, proving critical for future missions and the safety of astronauts and satellites alike.
The physics behind hypervelocity impacts reveals a fascinating interplay of forces and material responses. At such extreme speeds, traditional understandings of material strength and collision dynamics are often upended. Principles like hydrodynamic flow, where solids behave more like fluids under the stress of impact, become significantly more relevant. This area of study not only enriches our understanding of material science but also underscores the complexity of protecting spacecraft against seemingly minor threats that carry major consequences.
Simulation of Hypervelocity Impact
Simulating hypervelocity impact scenarios is crucial in understanding and mitigating the effects of such events in aerospace engineering and defense applications. Advanced computational methods enable engineers and scientists to predict the outcomes of high-speed collisions without the need for extensive physical testing.
A Hybrid Particle-Finite Element Method for Hypervelocity Impact Simulation
The hybrid particle-finite element method (HPFEM) is a cutting-edge approach for simulating hypervelocity impacts. It marries two powerful computational techniques: the finite element method (FEM), which excels at modelling continuous materials and structural responses, and the particle method, which is adept at simulating large deformations and fluid dynamics.HPFEM allows for a detailed analysis of the impact event, from the initial contact and penetration to the subsequent material deformation and damage propagation within the target material. By combining the strengths of both methods, HPFEM offers a more comprehensive and accurate simulation of hypervelocity impacts, especially in cases involving complex material behaviours and interactions.
In HPFEM, the finite element method handles the majority of the structural and material response simulation, providing high-fidelity models of stress, strain, and deformation. The particle method comes into play by modelling the behaviour of debris, ejected material, and any fluid-like phases that occur during and after the impact. This hybrid approach allows for simulations that capture both the solid mechanics of the impactor and target, as well as the fluid dynamics of melted or vaporised materials.This multifaceted simulation technique is particularly useful in aerospace engineering for designing spacecraft shields, predicting damage from micrometeorite or space debris impacts, and in military applications where armour penetration and fragmentation are key considerations.
The success of HPFEM simulations heavily relies on accurate material models and the precise calibration of simulation parameters, which are often obtained from experimental data.
Understanding Hypervelocity Impact Testing
Hypervelocity impact testing involves experimental setups that recreate high-speed collisions under controlled laboratory conditions. These tests are essential for validating simulations, understanding material responses, and designing protective systems against such impacts.The core of hypervelocity impact testing is the use of gas guns, light-gas guns, or laser-driven devices to accelerate projectiles to hypersonic speeds, mimicking the velocities of space debris, micrometeoroids, or ballistic projectiles. Researchers focus on measuring the damage on targets, which can range from aerospace materials to military armour platings, to improve theoretical models and simulation accuracy.
Hypervelocity impact testing facilities often employ high-speed cameras and diagnostic tools to capture the impact event and its immediate aftermath, offering valuable insights into the physics at play.
The dynamics of hypervelocity impacts reveal complex phenomena, such as shock wave propagation, extreme material deformation, and vaporisation. Test data help refine the constitutive models of materials — mathematical descriptions of how materials respond under different loads and conditions. These models are critical for the predictive accuracy of simulations like HPFEM, influencing the development of materials and protective strategies in various industries, from aerospace to defence.Impact testing not only illuminates the immediate effects of a hypervelocity collision but also contributes to a broader understanding of material science, including failure modes, strength under extreme conditions, and the behaviour of composite materials. The synergy between experimental testing and computational simulation pushes the boundaries of what's possible in engineering and materials science.
The Consequences of Hypervelocity Impact
The aftermath of a hypervelocity impact offers invaluable insights into the dynamics of high-speed collisions in space. These impacts, characterised by speeds greatly exceeding the speed of sound, manifest in various forms across engineering and space exploration. By analysing the craters and ejecta produced, scientists and engineers can glean critical information on impact dynamics, material responses, and even historical cosmic events.
Analysing Hypervelocity Impact Crater
An impact crater formed by a hypervelocity impact serves as a record of the collision's energy, direction, and the impacting object's composition. Craters are analysed based on several parameters:
- Diameter and Depth: Offering clues about the energy and mass of the impacting body.
- Morphology: Indicates the angle of impact and the surface characteristics at the moment of collision.
- Ejecta Distribution: Helps in understanding the material properties of both the impactor and the target.
Hypervelocity Impact Crater: A depression on a solid surface, formed when a space object collides with another at speeds so high that the crushed and vaporised debris from the impactor and the surface are ejected outwards, creating a characteristic crater feature.
What is Hypervelocity Impact Ejecta?
Hypervelocity impact ejecta refers to the debris that is launched away from the crater at the time of impact. This material provides a snapshot of the collision's immediate aftermath, including the state of the projectile and target at the moment of impact. Ejecta can encompass a range of materials:
- Vaporised Material: Particles that were melted and vaporised due to the extreme heat of the impact.
- Melted and Solid Fragments: Bits of the target and projectile that are either partially melted or remain intact.
- Secondary Crater Materials: Material that falls back to the surface, creating secondary craters around the main impact site.
Hypervelocity Impact Ejecta: The varied materials expelled from the impact site during a collision at hypervelocity, providing critical insight into the impact conditions and the materials involved.
Hypervelocity Impact Symposium Overview
The Hypervelocity Impact Symposium (HVIS) is an essential congregation of researchers, engineers, and academics interested in the study of high-speed impacts. This symposium covers a wide range of topics:
- Recent advancements in simulation and modelling of hypervelocity impacts.
- Innovative materials and designs for impact mitigation.
- Analysis of space debris and its management.
- Insights into natural hypervelocity impacts in the solar system.
The HVIS proceedings are a rich resource for anyone interested in the consequences of hypervelocity impacts, including detailed studies on crater formation and ejecta analysis.
Hypervelocity Impact - Key takeaways
- Hypervelocity Impact: Collision of objects at speeds greatly exceeding the speed of sound, significant in aerospace for space debris and micrometeoroid mitigation.
- Kinetic Energy Conversion: Critical aspect of hypervelocity impact physics causing extreme temperatures and material phase changes upon collision.
- Hybrid Particle-Finite Element Method (HPFEM): Advanced simulation technique combining FEM and particle method for detailed impact analysis in hypervelocity impact scenarios.
- Hypervelocity Impact Testing: Laboratory experiments mimicking high-speed collisions to validate simulations and understand material responses for spacecraft and military applications.
- Hypervelocity Impact Crater and Ejecta: Analysis of the aftermath of hypervelocity impacts providing insights into impact dynamics and development of impact mitigation strategies.
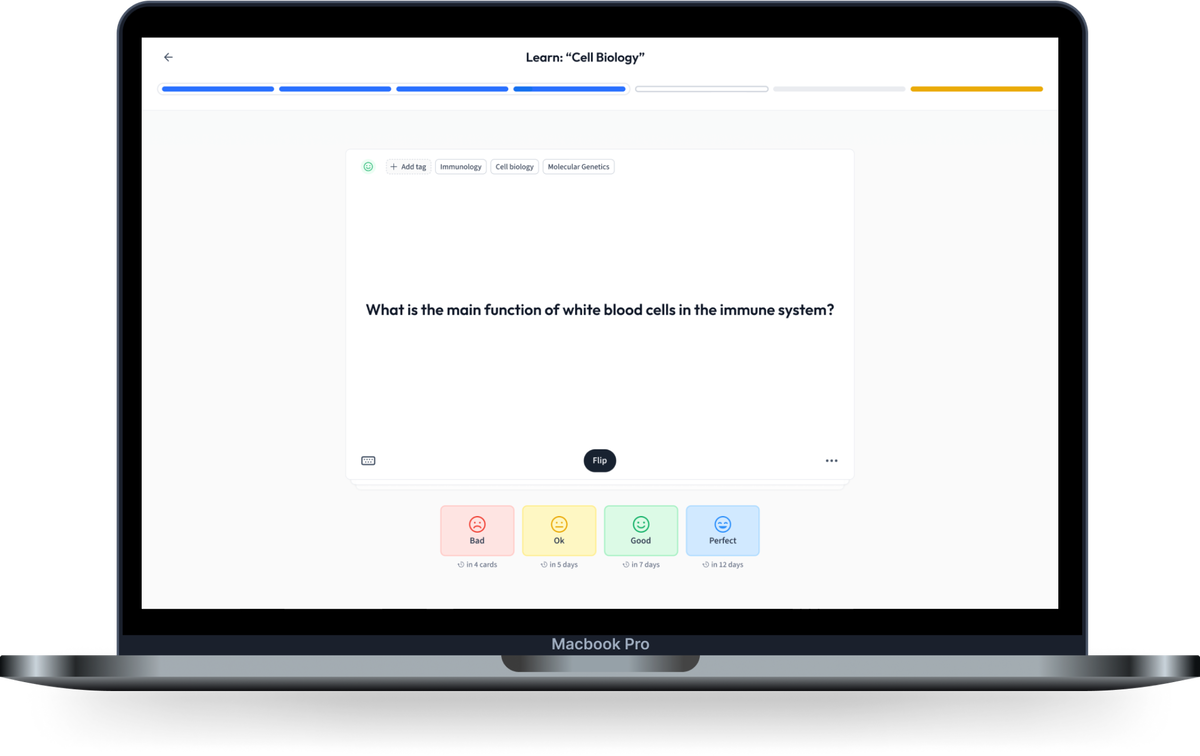
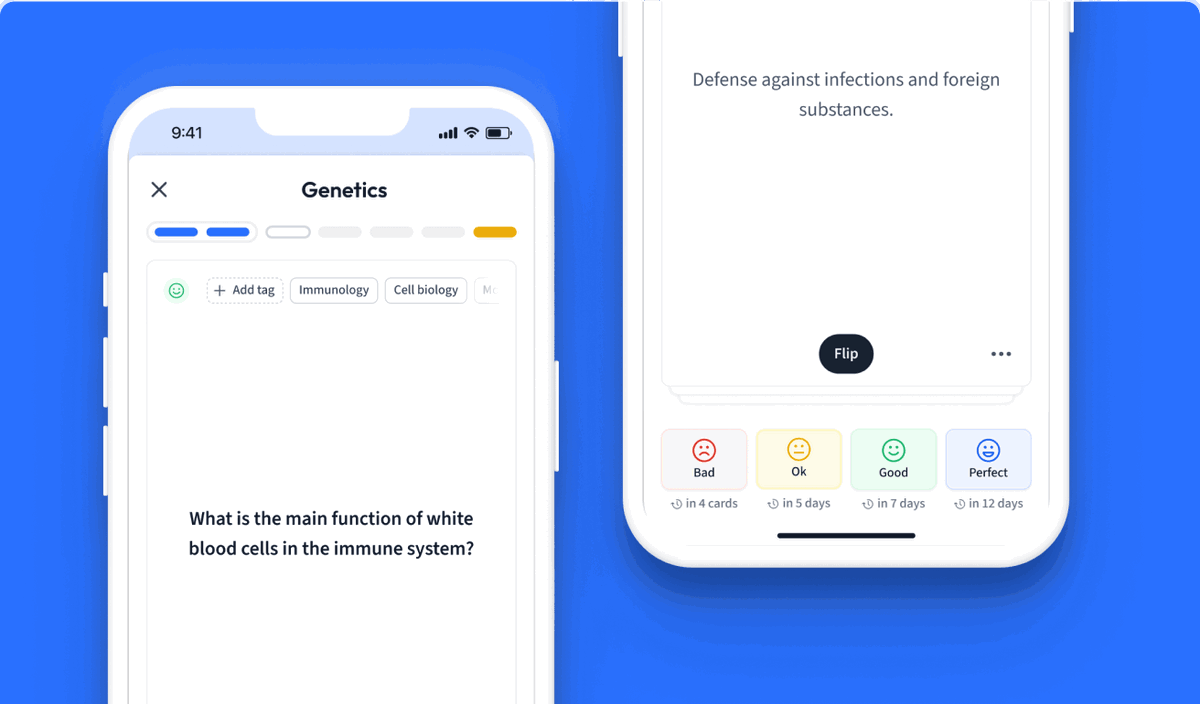
Learn with 12 Hypervelocity Impact flashcards in the free StudySmarter app
Already have an account? Log in
Frequently Asked Questions about Hypervelocity Impact
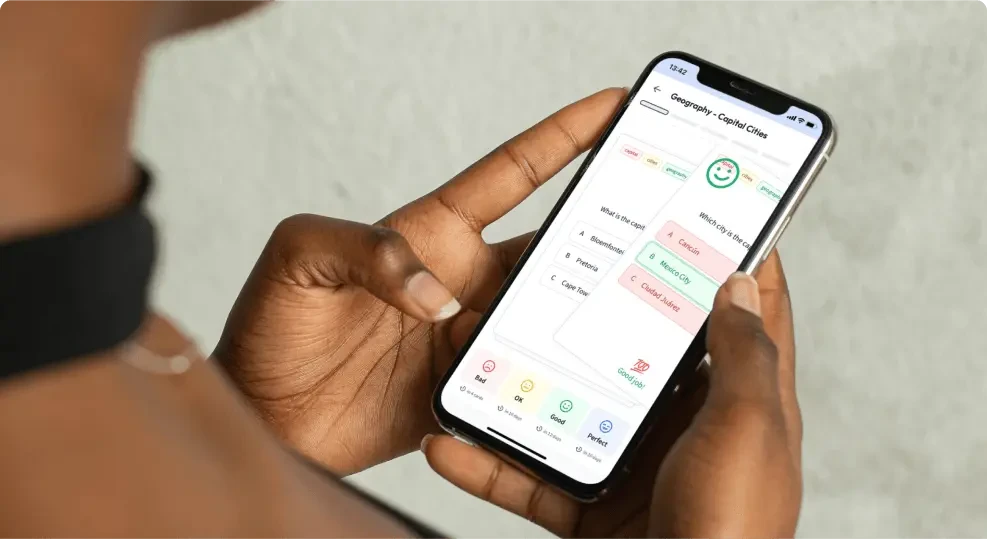
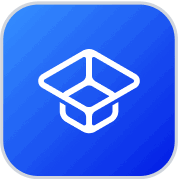
About StudySmarter
StudySmarter is a globally recognized educational technology company, offering a holistic learning platform designed for students of all ages and educational levels. Our platform provides learning support for a wide range of subjects, including STEM, Social Sciences, and Languages and also helps students to successfully master various tests and exams worldwide, such as GCSE, A Level, SAT, ACT, Abitur, and more. We offer an extensive library of learning materials, including interactive flashcards, comprehensive textbook solutions, and detailed explanations. The cutting-edge technology and tools we provide help students create their own learning materials. StudySmarter’s content is not only expert-verified but also regularly updated to ensure accuracy and relevance.
Learn more