Jump to a key chapter
What is Metal Fatigue?
Understanding Metal Fatigue Definition
Metal Fatigue: The progressive and localized structural damage that occurs when a material is subjected to cyclic loading. The nominally applied stress levels might be lower than the maximum strength of the material.
When metals are under the influence of cyclic stresses, they tend to develop fatigue. These stresses can induce micro-cracks within the material, which gradually propagate and can eventually lead to failure. This process can take place over thousands, millions, or even billions of cycles, depending on the material, the magnitude of the applied stress, the environment, and other factors.
Metal fatigue is not about a single catastrophic failure arising out of a single application of stress. It's an evolutionary process, where damage accumulates over time due to repeated application of stress. Engineers study metal fatigue to understand how materials behave under real-world conditions, where constant or variable stresses and strains are common. This helps in the prediction of a component's lifespan and in the development of more resilient materials.
Example: A common household example of metal fatigue is the breaking of a metal wire coat hanger. By bending the hanger back and forth repeatedly, micro-cracks begin to form at the point of bending. As the bending continues, these cracks expand and weaken the structure until it breaks. This demonstrates the principle of metal fatigue on a scale that is easy to observe.
Aircraft wings and mechanical parts often suffer from metal fatigue due to the repetitive stresses of takeoffs, flights, and landings.
Common Misconceptions About Metal Fatigue
There are several misconceptions about metal fatigue that can lead to a misunderstanding of its nature and effects. These misconceptions can significantly influence how problems related to metal fatigue are approached and solved.
- Misconception 1: Metal fatigue occurs only under high-stress conditions.
- Misconception 2: A material's maximum strength indicates its resistance to fatigue.
- Misconception 3: Metal fatigue is instantaneous and unpredictable.
Understanding that metal fatigue can occur even under low-stress conditions but over repetitive cycles is crucial for accurate material design and engineering. Additionally, the maximum strength of a material does not necessarily translate to high fatigue resistance. Each material reacts differently to cyclic stresses, and their fatigue life can be influenced by various factors including surface finish, temperature, and the presence of corrosive elements. The unpredictable nature of metal fatigue can often be mitigated through careful design and regular maintenance. Meticulous inspections and usage monitoring can identify potential fatigue issues before they lead to failure.
Causes of Metal Fatigue
Metal fatigue emerges as a critical concern in the realm of engineering, influencing the integrity and longevity of metal structures and components. Grasping the underlying causes of metal fatigue is pivotal in mitigating its adverse effects.
The Role of Stress Cycles in Metal Fatigue
The occurrence of metal fatigue is intricately linked to the repetitive application of stress cycles on a metal component. These stress cycles, even if of a lower magnitude than the material's ultimate strength, can induce the initiation and growth of cracks, leading to eventual failure. The frequent loading and unloading of stresses, such as those experienced by aircraft components or bridges, result in small, often microscopic, damages that accumulate over time. This process is not instantaneous but develops progressively, highlighting the significance of understanding stress cycles in predicting and preventing metal fatigue.
Example: Consider the repeated bending of a metal paperclip. Initially, the metal withstands the deformations, but as the bending persists, the material begins to weaken at specific points, illustrating how repeated stress cycles can lead to metal fatigue.
In engineering, the S-N curve is a fundamental tool used to predict the life of a material under cyclic stress. It plots the amplitude of cyclic stress against the logarithm of the number of cycles to failure.
Environmental Factors Contributing to Metal Fatigue
Beyond the mechanical stress cycles, environmental conditions play a profound role in accelerating or instigating metal fatigue. Factors such as temperature variations, humidity, corrosive environments, and exposure to UV light can exacerbate the formation and propagation of cracks in metals. Corrosion fatigue, in particular, results from the combined effects of cyclic stress and chemical attack, significantly reducing a metal's resistance to fatigue. Similarly, elevated temperatures can decrease a material's endurance limit, making it more susceptible to fatigue under conditions that would otherwise be considered safe.
The phenomenon of thermo-mechanical fatigue highlights the complex interplay between mechanical stresses and temperature fluctuations. In environments such as jet engines, where components are subjected to both high cyclic stresses and significant temperature gradients, the risk of metal fatigue is substantially increased. This underscores the importance of environmental considerations in the design and material selection process for engineering applications.
Importance of Material Quality in Metal Fatigue Resistance
The inherent quality of the metal significantly influences its ability to withstand fatigue. Material defects such as inclusions, voids, and irregular grain structures can act as sites for crack initiation, facilitating the onset of metal fatigue. Consequently, the selection of high-grade materials with minimal imperfections is a critical design consideration.Additionally, the method of material processing and treatment plays a substantial role in enhancing fatigue resistance. Techniques such as heat treatment, surface hardening, and shot peening can improve the material's resistance to cyclic stress, thus elevating its fatigue threshold. Understanding the relationship between material quality and metal fatigue resistance aids in the proactive design of more durable and reliable components.
Advanced composites and metals alloyed with elements like titanium or nickel are preferred in applications requiring high fatigue resistance, such as aerospace engineering.
Metal Fatigue Examples in Aerospace Engineering
Metal fatigue in aerospace engineering presents a crucial concern due to the significant safety and operational implications. Examples of metal fatigue within this field offer insights into both the challenges and advancements in managing fatigue-related issues.
Historical Metal Fatigue Failures in Aircraft
The history of aviation is punctuated with incidents where metal fatigue played a pivotal role in structural failures. Studying these incidents provides valuable lessons for current and future aerospace engineering projects.One notable example is the series of de Havilland Comet airliner crashes in the 1950s. Investigations pinpointed metal fatigue, exacerbated by square windows and the resulting stress concentrations, as the primary cause of these catastrophic failures. This led to the redesigning of aircraft windows with rounded corners to distribute stresses more evenly.
Example: A famous incident highlighting the impact of metal fatigue is the 1988 Aloha Airlines Flight 243. A significant portion of the aircraft's roof tore off mid-flight due to metal fatigue around rivet holes, leading to a critical reevaluation of aging aircraft maintenance programs.
Crack detection methods have evolved, with techniques like dye penetrant inspection and ultrasonic testing now common practices in aerospace engineering to identify potential metal fatigue.
Analysing Crack Initiation in Metal Fatigue
Crack initiation is the precursor to metal fatigue failure, and its understanding is crucial in extending the lifespan of aerospace components. It usually begins at points of stress concentration such as holes, notches, or material defects, and is significantly influenced by factors like load intensity and material properties.Through advanced analytical methods such as Finite Element Analysis (FEA), engineers can simulate stress distributions within components and identify potential crack initiation sites. This predictive capability is invaluable in designing components that are more resistant to metal fatigue.
The science behind crack initiation often delves into the microscopic level, where material imperfections or dislocations play a substantial role. Metal fatigue might start at a microscopic scale not visible to the naked eye, but these micro-cracks can grow under repeated stress cycles to form visible cracks that lead to failure.
Lessons Learnt from Metal Fatigue in Aerospace Components
The lessons learnt from past failures and subsequent research into metal fatigue are continually informing the development of more durable aerospace components. Today, the integration of better materials, design practices, and maintenance strategies reflects the industry's commitment to safety.
- Material selection has shifted towards alloys that exhibit higher fatigue strength and resistance to crack propagation.
- Design practices now include the allocation of ‘safe-life’ or ‘fail-safe’ criteria, ensuring components are replaced before reaching their fatigue life or are designed to sustain damage without catastrophic failure.
- Maintenance strategies have evolved to include regular inspections and the use of non-destructive testing methods to detect early signs of fatigue.
The application of shot peening to introduce compressive residual stresses on the surface of components is an engineering response aimed at increasing resistance to crack initiation and growth.
Preventing and Detecting Metal Fatigue Failure
Preventing and detecting metal fatigue failure is pivotal to ensuring the longevity and safety of engineering structures and components. Through advanced techniques and best practices, engineers can address the challenges posed by metal fatigue.
Techniques for Identifying Metal Fatigue Early
Identifying metal fatigue at early stages is crucial for taking preventive measures before any significant damage or failure occurs. Various techniques have been developed for this purpose, including visual inspections, non-destructive testing (NDT), and monitoring systems.
- Visual Inspections: The simplest method, involving regular checks for signs of wear, cracks, or deformation.
- Non-Destructive Testing (NDT): Techniques such as ultrasonic testing, magnetic particle testing, and dye penetrant inspection are employed to detect flaws or cracks without damaging the component.
- Monitoring Systems: Advanced sensor technologies can continuously monitor stresses and strains on components, alerting engineers to potential fatigue issues.
Example: Ultrasonic testing is widely used in aerospace to detect cracks in the aircraft fuselage and wings. This method utilizes high-frequency sound waves to identify discontinuities in the material structure.
Among NDT methods, dye penetrant inspection is particularly effective for identifying surface cracks, including those invisible to the naked eye.
Advances in Materials Engineering to Combat Metal Fatigue
Materials engineering has made significant strides in developing metals and alloys with improved resistance to metal fatigue. These advances include the creation of high-strength alloys, surface engineering techniques, and the application of composite materials.
- High-Strength Alloys: New alloys are engineered to have a higher resistance to fatigue by improving tensile strength and ductility.
- Surface Engineering: Techniques like shot peening and laser peening introduce compressive residual stresses on the surface, enhancing fatigue resistance.
- Composite Materials: Incorporating fibres such as carbon or glass into a polymer matrix can significantly increase fatigue resistance compared to traditional metals.
One innovative approach in materials engineering is the development of shape memory alloys (SMAs). SMAs can return to their original shape after deformation, showing remarkable resistance to fatigue. This property is particularly useful in applications requiring materials to withstand cyclic loads without suffering degradation.
Implementing Best Practices in Maintenance to Prevent Metal Fatigue
Effective maintenance practices are essential for managing and mitigating the risks associated with metal fatigue. These practices not only involve regular inspections and repairs but also incorporate strategies for predictive maintenance and life cycle management.
- Regular Inspections and Repairs: Scheduled inspections allow for early detection of potential fatigue, while timely repairs prevent minor issues from escalating.
- Predictive Maintenance: Using data from monitoring systems to predict when a component may fail, allowing for maintenance before an actual failure occurs.
- Life Cycle Management: Understanding the expected lifecycle of components and replacing them proactively before fatigue leads to failure.
Predictive maintenance has become more accessible with the advent of Internet of Things (IoT) technologies, enabling real-time monitoring of components for signs of metal fatigue.
Metal Fatigue - Key takeaways
- Metal Fatigue Definition: A progressive, localized structural damage occurring from cyclic loading, often at stress levels lower than the material's maximum strength.
- Causes of Metal Fatigue: Initiated by micro-crack formation under cyclic stress; influenced by factors like material quality, environmental conditions, and stress cycles.
- Crack Initiation in Metal Fatigue: Often occurs at points of stress concentration; predictive tools like Finite Element Analysis help identify potential initiation sites.
- Misconceptions about Metal Fatigue: It can happen even under low-stress conditions, maximum strength doesn't equate to fatigue resistance, and it's not always instantaneous or unpredictable.
- Metal Fatigue Examples: Includes the breaking of a wire coat hanger from repeated bending, and aircraft failures due to repeated stress from takeoffs and landings.
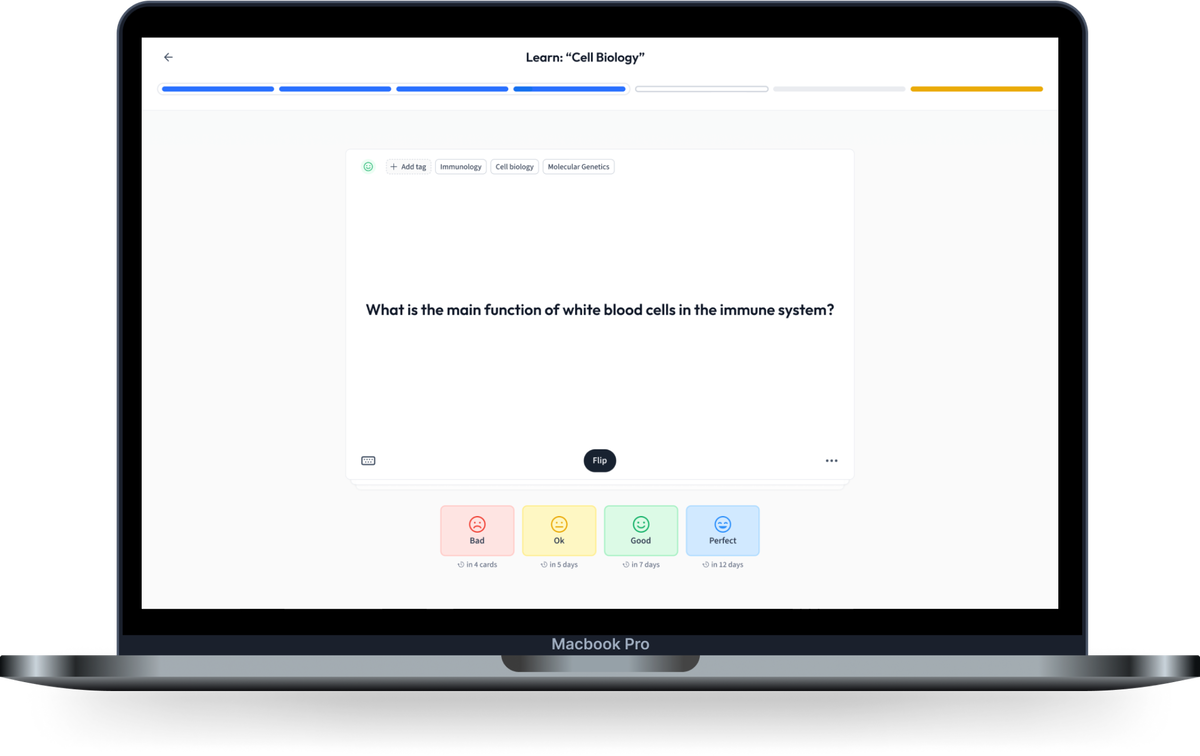
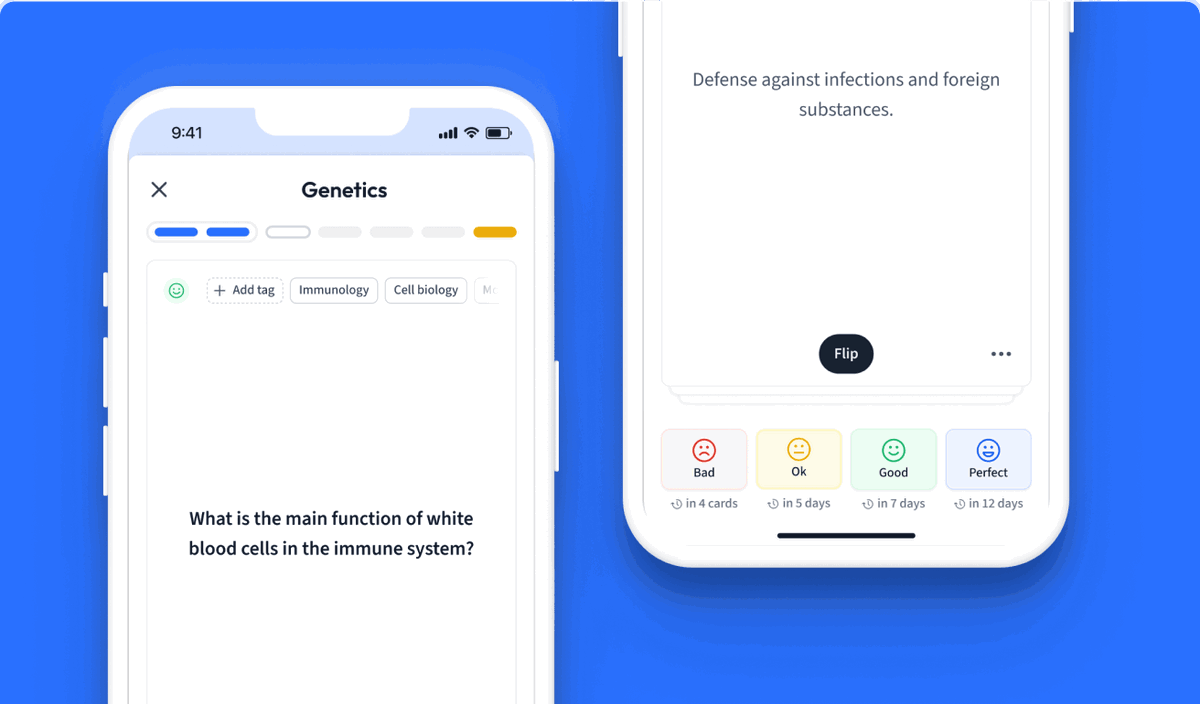
Learn with 12 Metal Fatigue flashcards in the free StudySmarter app
Already have an account? Log in
Frequently Asked Questions about Metal Fatigue
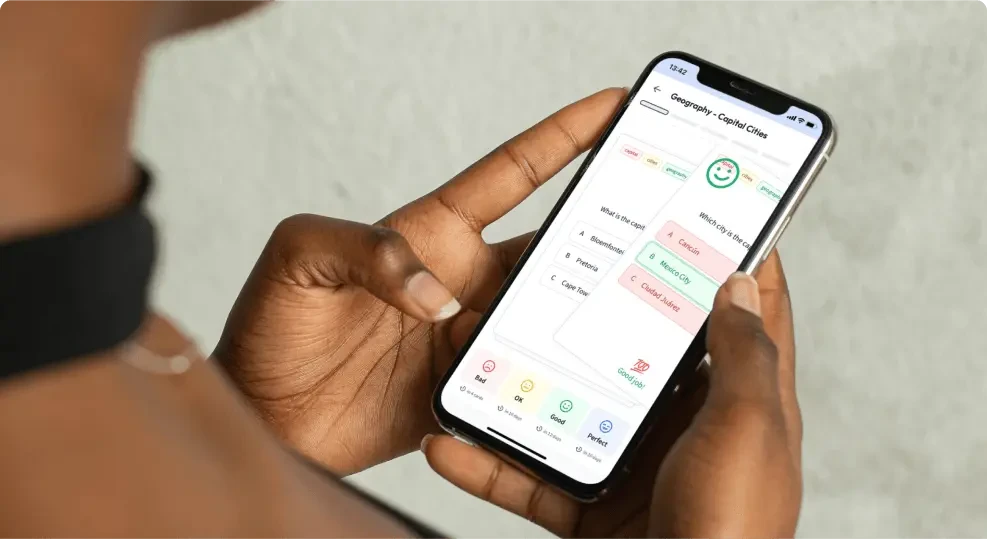
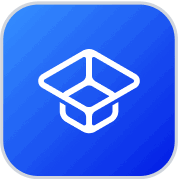
About StudySmarter
StudySmarter is a globally recognized educational technology company, offering a holistic learning platform designed for students of all ages and educational levels. Our platform provides learning support for a wide range of subjects, including STEM, Social Sciences, and Languages and also helps students to successfully master various tests and exams worldwide, such as GCSE, A Level, SAT, ACT, Abitur, and more. We offer an extensive library of learning materials, including interactive flashcards, comprehensive textbook solutions, and detailed explanations. The cutting-edge technology and tools we provide help students create their own learning materials. StudySmarter’s content is not only expert-verified but also regularly updated to ensure accuracy and relevance.
Learn more