Jump to a key chapter
Understanding Orbital Simulation
Orbital simulation plays a critical role in the field of engineering, especially in aerospace, where understanding the motion of planets, satellites, and spacecraft relative to one another is essential. Such simulations provide insights into the mechanics of how bodies move in space, influenced by various forces, foremost being gravity.
The Basics of Orbital Dynamics Fundamentals
Orbital dynamics is the study of the motion of objects in space under the influence of forces such as gravity. This branch of physics is vital for designing and analysing the trajectories of spacecraft and satellites. At its core, the subject is governed by Newton's laws of motion and universal gravitation.
Orbital dynamics: A branch of classical mechanics that focuses on the motion of celestial bodies and artificial satellites in space, primarily under the influence of gravitational forces.
Example: The movement of the Moon around the Earth can be explained using orbital dynamics, where the gravitational pull from the Earth influences the Moon's path, causing it to orbit in an elliptical fashion.
The primary equations used in orbital dynamics include:
- Newton's Second Law: \( F = ma \), where \( F \) is the force applied, \( m \) is the mass of the object, and \( a \) is the acceleration.
- Universal Law of Gravitation: \( F = G\frac{m_1m_2}{r^2} \), where \( G \) is the gravitational constant, \( m_1 \) and \( m_2 \) are the masses of two bodies, and \( r \) is the distance between their centres.
Exploring the Gravity and Orbits PhET Simulation
The Gravity and Orbits PhET Simulation is an interactive, web-based tool that helps visualise the orbits of planets and other celestial bodies around the Sun. It serves as an educational resource, allowing users to explore how gravity affects the motion of objects in the solar system.
This simulation is ideal for introducing the concept of gravitational forces and orbits in a visually engaging manner.
Users can
- Adjust the mass and distance of planets and stars to see how these factors influence orbital paths,
- Experiment with different initial velocities,
- Observe the effects of gravitational forces between different celestial bodies.
Using the NAAP Planetary Orbit Simulator
The NAAP Planetary Orbit Simulator is another invaluable online tool that offers a more detailed examination of planetary orbits. It enables users to study various aspects of orbits, including season changes, eccentricity, and the effect of a star's mass on a planet's orbit.
NAAP Planetary Orbit Simulator: A sophisticated web-based simulator developed by the National Astronomical Observatory of Japan (NAAP) that models the orbits of planets in our solar system and beyond.
Key features of the NAAP simulator include:
- Illustrations of Kepler's laws of planetary motion,
- Real-time modelling of orbits with adjustable variables,
- Capability to simulate the orbital paths of exoplanets.
Orbital Mechanics for Engineering Students
Orbital mechanics is a fundamental branch of aerospace engineering that involves the study of the motions of artificial and natural celestial bodies. Understanding the principles of orbital mechanics is essential for the development and operation of space missions, including satellite launches, planetary exploration, and deep-space voyages.
Introduction to Planet Orbit Simulator
Planet Orbit Simulators are sophisticated software tools designed to model and analyse the trajectories of planets, satellites, and spacecraft within the solar system and beyond. These simulators utilise the laws of physics, particularly Newton's laws of motion and the law of universal gravitation, to predict the paths that celestial bodies follow through space.
Planet Orbit Simulator: A computational tool that enables the simulation of celestial mechanics, including the orbits of planets, moons, and artificial satellites around larger celestial bodies.
Example: NASA's Eyes on the Solar System is an example of a Planet Orbit Simulator that allows users to virtually explore the cosmos, tracking the real-time positions of spacecraft and celestial bodies.
Exploring the capabilities of Planet Orbit Simulators reveals the intricacies of gravitational interactions in the cosmos. Users can adjust variables such as mass, velocity, and distance to see their effects on orbital dynamics. This hands-on approach not only enhances one's understanding of celestial mechanics but also stimulates curiosity about the universe's complex behaviour.
How Orbital Mechanics Influence Space Exploration
Orbital mechanics is crucial in planning and executing space missions, influencing the trajectory design, fuel requirements, and launch windows. It dictates how spacecraft navigate through space, perform gravity assists, and achieve stable orbits or land on celestial bodies.
Gravity assists, also known as slingshot manoeuvres, utilise the gravitational pull of planets to boost a spacecraft's velocity.
The principles of orbital mechanics ensure that spacecraft can reach their intended destinations with the highest efficiency. For instance, the trajectory of a mission to Mars must account for the movement of both Earth and Mars to optimise the launch window and minimise fuel consumption.Through orbital simulations, engineers can predict and fine-tune these trajectories, significantly improving the mission's success rate and cost-effectiveness.
The historical Apollo moon missions serve as a testament to the impact of orbital mechanics on space exploration. NASA's meticulous calculations and use of orbital mechanics ensured not only the safe landing and return of astronauts but also the precise timing needed to land at specific locations on the Moon. Similar principles have been applied to send rovers to Mars, orbiters to Saturn, and probes beyond the solar system.
How Can Gravity Be Simulated in an Orbiting Space Station?
Simulating gravity in an orbiting space station represents one of the most challenging yet fascinating aspects of aerospace engineering. Creating a semblance of Earth's gravitational force in the microgravity environment of space is crucial for ensuring the health and performance of astronauts during long-term missions.
The Principles Behind Simulating Gravity
The principle behind simulating gravity in space stations revolves around creating artificial gravity through centripetal force. This technique is based on Newton's laws of motion, specifically the law which states that for any object moving in a circle of radius \(r\) at velocity \(v\), the centripetal force \(F\) required to keep it moving in that circle can be calculated as \(F = \frac{mv^2}{r}\), where \(m\) is the mass of the object.
Centripetal Force: A force that acts on a body moving in a circular path and is directed towards the centre around which the body is moving.
Example: Imagine tying a ball to a string and spinning it around in a circle. The ball experiences a force (centripetal force) that keeps it moving in a circular path. In a space station, this concept can be scaled up, using the station's rotation to create a similar force that simulates gravity for the astronauts inside.
The concept of centripetal force for gravity simulation was popularised by the science fiction movie '2001: A Space Odyssey', which depicted a rotating space station that created artificial gravity.
Techniques to simulate gravity involve designing space stations or modules that rotate, generating centripetal force to mimic the gravitational pull. The outer walls of the rotating space habitat would serve as the 'floor' where astronauts could walk, with the 'force' keeping them grounded, akin to how gravity works on Earth.
Practical Applications in Aerospace Engineering
The practical applications of simulating gravity in aerospace engineering are manifold, directly impacting the well-being of astronauts and the success of long-duration spaceflight missions. These applications are crucial for:
- Maintaining astronaut health by preserving muscle mass and bone density,
- Facilitating normal fluid distribution within the body,
- Improving the functionality and comfort of living and working environments in space.
Artificial Gravity: A simulated force perceived as similar to gravity, created through mechanical means such as the rotation of a spacecraft or space habitat, to counteract the effects of weightlessness in space.
The implementation of artificial gravity on a space station is a complex engineering challenge that involves considerations of rotational velocity, station design, and the potential effects on the human body. Studies on artificial gravity often incorporate advanced computer simulations to understand how different rotation rates and habitat sizes influence the viability and comfort of such an environment. These simulations predict how objects and humans would interact within a space designed to mimic Earth-like gravity, ensuring the practicality of daily activities and the long-term feasibility of habitation.
Applying Orbital Simulation in Real-World Scenarios
Orbital simulation is a cutting-edge tool that finds extensive application in various sectors beyond aerospace engineering. By utilising sophisticated computational models, it's possible to simulate and analyse the complex interactions between celestial bodies. These simulations can significantly impact the design, planning, and execution of projects involving satellites, interplanetary missions, and even telecommunications infrastructure.
Designing Projects Using Orbital Mechanics
The application of orbital mechanics is crucial in the design and development of space missions. With the advance of technology, engineers utilise simulations to plan the trajectories of spacecraft, ensuring they reach their intended destinations while consuming minimal fuel. Simulations provide insights into the gravitational influences of celestial bodies on the spacecraft, enabling the calculation of optimal launch windows and trajectory paths. Such precision is essential for the success of missions to distant planets or for placing satellites into specific orbits.
Orbital mechanics: The branch of physics that studies the movement of objects in space, including planets, satellites, and spacecraft, under the influence of gravity and other forces.
Applications of orbital mechanics in project design extend to:
- Assessment and mitigation of potential collision risks for satellites in crowded orbits,
- Development of efficient space debris removal strategies,
- Design of interplanetary missions that utilise gravity assists for speed augmentation.
Challenges and Solutions in Simulating Orbits
Simulating orbits presents unique challenges, primarily due to the complex nature of gravitational fields and the perturbative forces that affect celestial bodies. Accuracy in simulation requires consideration of numerous factors, including the non-uniformity of celestial bodies, atmospheric drag, solar radiation pressure, and third-body effects. Solutions to these challenges often involve the enhancement of simulation software with sophisticated algorithms that can model these forces with high precision. Additionally, the integration of real-world data from space missions helps refine and validate simulation models, improving their reliability and accuracy.
Example: In designing a satellite's orbit, engineers must simulate the effects of Earth's gravity, atmospheric drag, and the gravitational pull from the moon and sun. Advanced orbital simulations allow for adjustments in the satellite's trajectory to account for these variables, ensuring long-term stability and reducing the risk of collisions.
In addition to gravitational forces, simulating orbits also requires careful consideration of the spacecraft's propulsion system and fuel capacity, as these factors are crucial for course corrections and maintaining the intended orbit.
One of the most significant challenges in orbital simulation is the n-body problem, which concerns the prediction of movements of celestial bodies interacting with each other through gravity. Traditional simulation methods simplify these interactions by assuming two-body dynamics, but real-world scenarios often involve complex interactions between multiple bodies. Innovations in computational physics and the use of numerical methods have led to more accurate solutions to the n-body problem, allowing for more reliable simulations that can predict the long-term stability of orbits in multi-body systems.
Orbital Simulation - Key takeaways
- Orbital Simulation: A critical tool in aerospace engineering for understanding the motion of celestial bodies, dependent on forces such as gravity.
- Orbital Dynamics Fundamentals: Governed by Newton's laws of motion and the Universal Law of Gravitation, it involves the study of objects' motion in space due to gravitational pull and other forces.
- Gravity and Orbits PhET Simulation: An interactive platform for visualising planetary orbits and experimenting with gravitational effects in an educational context.
- Planet Orbit Simulator: Computational tools that model celestial mechanics using physics laws to predict the paths of planets and artificial satellites.
- Simulating Gravity in Space: Achieved through the application of centripetal force, enabling the creation of artificial gravity in orbiting space stations to maintain astronaut health.
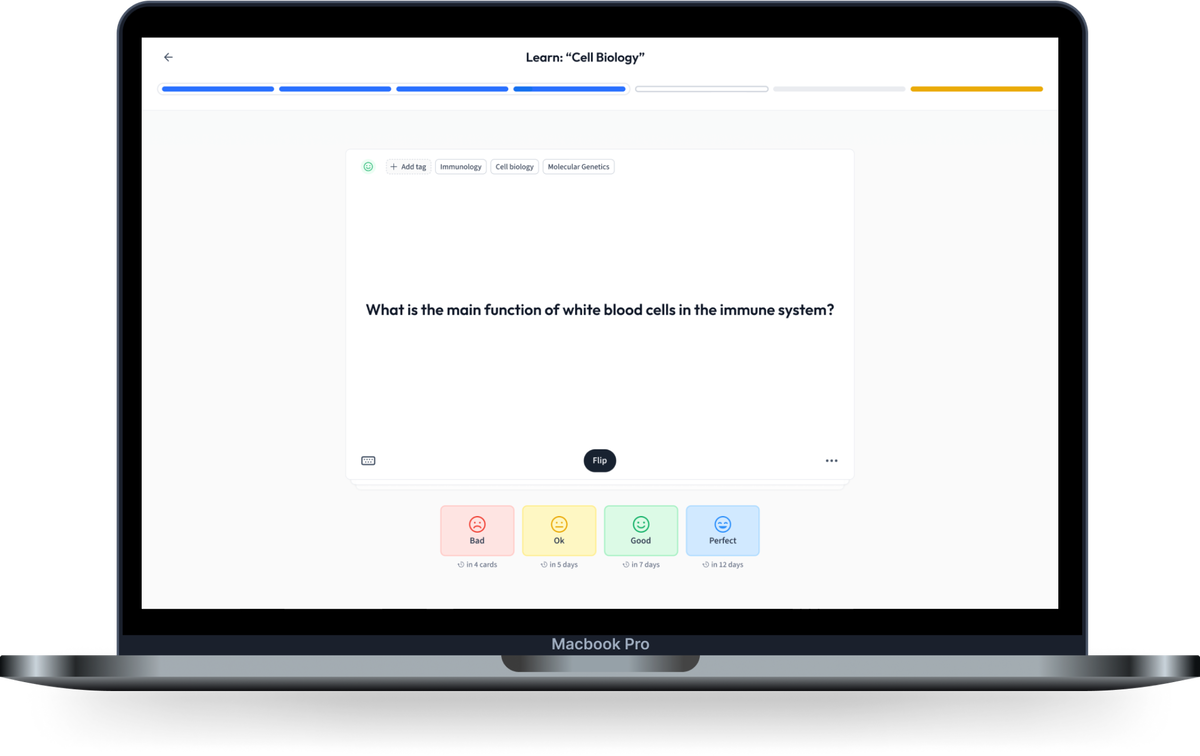
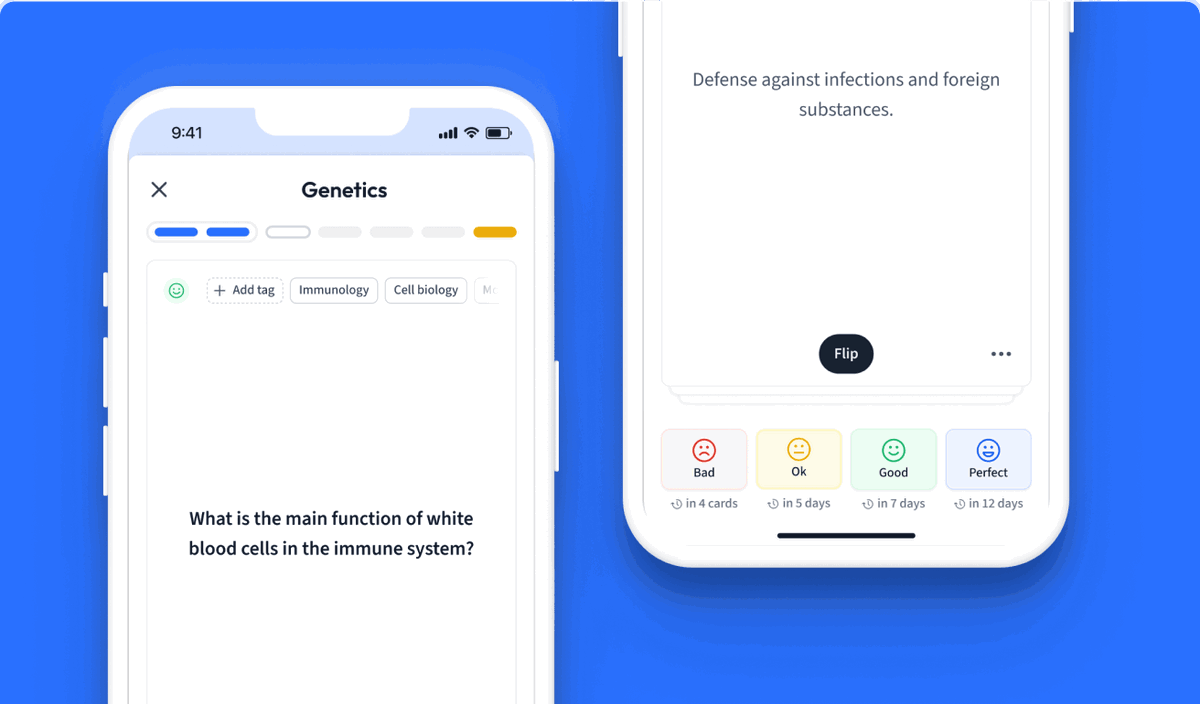
Learn with 12 Orbital Simulation flashcards in the free StudySmarter app
Already have an account? Log in
Frequently Asked Questions about Orbital Simulation
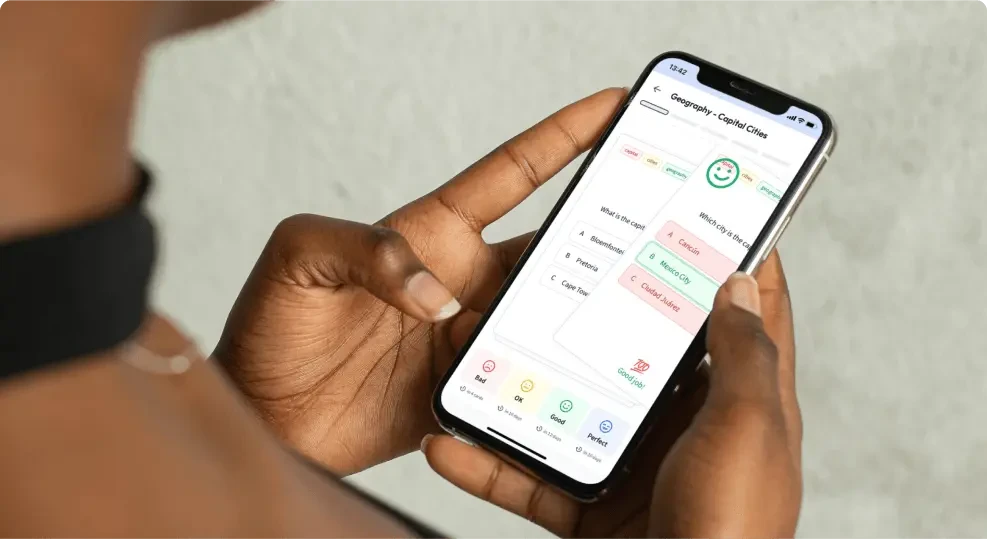
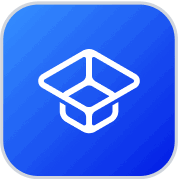
About StudySmarter
StudySmarter is a globally recognized educational technology company, offering a holistic learning platform designed for students of all ages and educational levels. Our platform provides learning support for a wide range of subjects, including STEM, Social Sciences, and Languages and also helps students to successfully master various tests and exams worldwide, such as GCSE, A Level, SAT, ACT, Abitur, and more. We offer an extensive library of learning materials, including interactive flashcards, comprehensive textbook solutions, and detailed explanations. The cutting-edge technology and tools we provide help students create their own learning materials. StudySmarter’s content is not only expert-verified but also regularly updated to ensure accuracy and relevance.
Learn more