Jump to a key chapter
Understanding Planetary Atmospheres
Planetary atmospheres are essential in determining the conditions on the surface of planets, including whether or not they are capable of supporting life. Exploring the composition, the process of light scattering, and the thermodynamics of these atmospheres provides invaluable insights into the workings of various celestial bodies.
Composition of Planetary Atmospheres
The composition of a planetary atmosphere is dictated by its original material, the process of planetary differentiation, and further alterations by geological and biochemical processes. Main constituent gases often include hydrogen, helium, carbon dioxide, and nitrogen, while trace amounts of other elements can provide clues about the planet's history and potential habitability. For instance, Earth's atmosphere is primarily composed of nitrogen (78%) and oxygen (21%), with trace amounts of other gases like argon, carbon dioxide, and neon. This mixture supports life and influences the planet's climate. In contrast, Venus has a dense carbon dioxide atmosphere, exerting significant greenhouse effect that renders its surface extraordinarily hot.
Mars, with its thin, mostly carbon dioxide atmosphere, offers a stark contrast to Earth, showcasing how atmospheric composition affects surface conditions.
Light Scattering in Planetary Atmospheres
Light scattering in planetary atmospheres is a critical process that influences their appearance and the amount of light and heat that reaches the surface. It occurs when sunlight interacts with atmospheric particles, scattering in different directions. Rayleigh scattering, responsible for Earth's blue skies and the reddening of the sun at sunset and sunrise, occurs when particles are much smaller than the wavelength of light hitting them. Conversely, Mie scattering happens with particles about the same size as the light's wavelength, affecting how clouds and hazes appear from space.
The colour of a planet's sky can tell us a lot about the composition and size of its atmospheric particles.
Thermodynamics of Planetary Atmospheres
The thermodynamics of planetary atmospheres involves the study of energy and temperature changes within an atmospheric system. This includes understanding heat transfer processes, atmospheric pressure, volume, and entropy changes. Understanding these mechanisms helps scientists predict weather patterns, climate change, and the potential habitability of exoplanets. Key thermodynamic processes in atmospheres include conduction, where heat is transferred through direct contact; convection, involving the movement of warm and cool air masses; and radiation, the emission of energy from the planet into space. Each of these processes plays a vital role in how energy is distributed across a planet, affecting everything from local weather to global climate patterns.
An interesting aspect of planetary thermodynamics is the greenhouse effect, a natural process that warms a planet's surface. When the Sun's energy reaches the planet, some is reflected back to space and the rest is absorbed, warming the planet. The planet then emits this heat, but certain gases in the atmosphere (greenhouse gases) trap some of this escaping heat, warming the atmosphere. This process is crucial for life on Earth as it keeps our planet at a habitable temperature. However, the balance is delicate, and too much of a greenhouse effect, as seen on Venus, can lead to runaway heating, making a planet inhospitable.
Spectroscopy and Radiative Transfer of Planetary Atmospheres
Spectroscopy and radiative transfer play critical roles in understanding the complex processes of planetary atmospheres. Through spectroscopy, scientists can identify the chemical makeup of a planet's atmosphere, while radiative transfer models help explain how energy moves through these atmospheres. This knowledge is key for uncovering the conditions on other planets and potentially finding signs of life.
Basics of Spectroscopy in Planetary Studies
Spectroscopy is a technique used to measure the light spectrum emitted, absorbed, or scattered by materials, allowing identification of their composition. In planetary atmospheres, spectroscopy examines the light from the Sun that has passed through a planet's atmosphere or the light emitted by the planet itself. This analysis reveals vital clues about atmospheric gases, their concentrations, and even the presence of organic molecules.Light interacts with molecules in the atmosphere, leaving distinctive markers or spectral lines in the light's spectrum, which can be observed with various types of spectrometers. By analysing these lines, scientists can deduce which gases are present in an atmosphere and in what quantities.
Spectroscopy: A scientific measurement technique that explores how light interacts with matter. By studying the spectra of light absorbed, emitted, or scattered by materials, spectroscopy can reveal their composition, physical properties, and chemical state.
For instance, when observing Mars, spectroscopy has detected signatures of carbon dioxide, water vapour, and methane in its atmosphere, providing insights into its climatic conditions and the potential for sustaining life.
Each chemical element or compound emits or absorbs light over characteristic wavelengths, serving as a unique fingerprint for identification.
Radiative Transfer Mechanisms in Planetary Atmospheres
Radiative transfer describes how energy in the form of electromagnetic radiation travels through and interacts with a planetary atmosphere. It involves both the absorption of sunlight by atmospheric gases and the emission of infrared radiation from the planet. These processes are fundamental for heating the atmosphere, determining the planet's temperature profile, and driving weather and climate systems.Key mechanisms in radiative transfer include absorption, emission, and scattering. These mechanisms can vary greatly across different planets, depending on factors such as atmospheric composition, pressure, and temperature.
Radiative Transfer: A process by which electromagnetic radiation is transmitted, absorbed, and emitted by substances, altering the energy balance and thermal structure within an environment, such as a planetary atmosphere.
Absorption and emission of radiation are governed by a planet's atmospheric composition, leading to phenomena like the greenhouse effect. For example, Earth's atmosphere traps heat through the absorption of infrared radiation by greenhouse gases, moderating our planet's temperature. On the other hand, an atmosphere like Venus's, with its thick clouds of sulfuric acid and abundant carbon dioxide, results in a runaway greenhouse effect, showing the dramatic impact of radiative transfer processes on planetary climates.
Radiative transfer models must take into account a planet's geometry and how sunlight angles and path lengths through the atmosphere vary, affecting the overall climate and temperature distribution.
Chemical Processes in Planetary Atmospheres
Understanding the chemical processes within planetary atmospheres allows scientists to uncover the dynamics that govern weather patterns, climate change, and the potential for life on other planets. These processes involve a variety of chemical reactions and physical interactions that contribute to the overall state of the atmosphere.These interactions are crucial for maintaining the balance of gases, contributing to the temperature regulation of planets, and influencing their ability to support life.
Role of Chemical Reactions
Chemical reactions in planetary atmospheres play a pivotal role in shaping the environment of a planet. Processes such as photochemical reactions, where sunlight breaks down or alters chemical compounds, and thermochemical reactions, driven by the planet's internal heat, continuously transform the atmospheric composition.The presence of oxygen in Earth's atmosphere, for instance, is largely the result of photosynthesis—a biochemical reaction where plants and other organisms convert carbon dioxide and water into oxygen and glucose using sunlight.
Photochemical reactions: Chemical reactions initiated by the absorption of light by atoms or molecules, leading to changes in the chemical composition of an atmosphere.
A well-known photochemical reaction in Earth's atmosphere is the formation of ozone. Ultraviolet (UV) light from the Sun splits oxygen molecules (O2), and the resulting oxygen atoms (O) then combine with unbroken O2 molecules to form ozone (O3). This ozone layer plays a crucial role in protecting life on Earth by absorbing the bulk of the Sun's harmful UV radiation.
Many of the colours seen on planets are due to the chemical compositions of their atmospheres and the reactions occurring within them. For example, Jupiter's red spots and bands are attributed to complex organic molecules and compounds such as phosphorus.
Dynamics of the Planetary Boundary Layer in the Atmosphere
The planetary boundary layer (PBL) is the part of the atmosphere that is directly influenced by its contact with a planetary surface and responds to surface forcings, such as evaporation, heat fluxes, and friction, over a timescale of about an hour or less.Its dynamics are crucial for the distribution of particulates, gases, and heat, affecting everything from local weather patterns to global climate systems. The thickness and characteristics of the PBL can vary significantly depending on the time of day, weather conditions, and geography of the planet.
Planetary Boundary Layer (PBL): The lowest part of the atmosphere, where its behaviour is directly influenced by its interaction with the planet's surface. This layer is critical in the transfer of heat, moisture, and momentum between the surface and the atmosphere.
The urban heat island effect can be observed in cities, where structures such as buildings and roads absorb and retain heat, affecting the temperature and dynamics of the local planetary boundary layer. This effect leads to warmer temperatures in urban areas compared to their rural surroundings, demonstrating how human activities can influence the PBL.
In the context of extraterrestrial atmospheres, understanding the PBL is critical for designing missions and interpreting data from planetary landers and rovers. For instance, the density and composition of Mars' PBL can tell scientists a lot about Martian dust storms, air circulation patterns, and even the potential for harvesting water vapour from the Martian atmosphere.Mars' PBL behaves differently from Earth's due to the thinner atmosphere and weaker gravity, showing significant daily temperature swings and playing a key role in the planet's dust storms that can envelope the entire planet.
The dynamics of the PBL are not only influenced by surface conditions but also by the planetary atmosphere's overall composition, which can significantly affect its capacity to transfer heat and moisture.
Practical Applications of Understanding Planetary Atmospheres
The exploration of planetary atmospheres not only deepens our understanding of the cosmos but also has practical applications that affect both space exploration and our understanding of Earth's own weather and climate systems. By studying these extraterrestrial environments, scientists can refine models of Earth's atmosphere, predict changes more accurately, and design efficient methods for exploring other planets.
Exploring Planetary Weather and Climate
Understanding the intricacies of planetary weather and climate plays a significant role in comprehending how planets evolve over time and the potential they hold for supporting life. By examining factors such as atmospheric composition, pressure, and temperature, researchers can make informed predictions about weather patterns and climatic conditions on other planets.
- Comparative climatology offers insights into Earth's climate by studying Venus' greenhouse effect or Mars' dust storms.
- Mapping out seasonal changes and weather phenomena on gas giants like Jupiter helps in understanding atmospheric dynamics in extreme conditions.
For example, the Curiosity Rover's observations of Martian dust devils have contributed to a better understanding of Mars' atmospheric dynamics, which are significantly influenced by the planet's surface features and solar heating patterns.
Observations of the extreme winds on Neptune, which reach speeds of up to 2,100 kilometres per hour, demonstrate how vastly different atmospheric dynamics can be when compared to Earth.
Implications for Planet Exploration and Study
The study of planetary atmospheres extends beyond mere academic curiosity; it is integral to the future of space exploration. Techniques developed for understanding these alien environments can be applied to the search for exoplanets with conditions suitable for life.
- Identifying signatory gases that indicate biological activity can guide astronomers in the search for habitable worlds beyond our Solar System.
- Understanding atmospheric composition is critical for designing spacecraft capable of entering a planet's atmosphere, conducting studies, or landing on its surface.
One fascinating application of planetary atmosphere research is the study of Venus' atmosphere to understand Earth's future. Venus, often called Earth's twin due to its similar size and composition, experienced a runaway greenhouse effect that led to extreme temperatures. By comparing Venus' atmospheric conditions to Earth's, scientists can explore scenarios related to global warming and the long-term impacts on Earth's climate.Such comparative studies are invaluable for developing strategies to mitigate climate change and predicting possible futures for our planet.
Technologies developed for atmospheric entry and exploration, such as heat shields and aerobraking techniques, also enhance our capabilities for returning to Earth safely from space missions.
Planetary Atmospheres - Key takeaways
- Planetary Atmospheres: Layers of gases surrounding a planet, crucial for determining surface conditions and potential to support life.
- Composition of Planetary Atmospheres: Includes main gases like hydrogen, helium, carbon dioxide, and nitrogen; Earth's atmosphere consists of 78% nitrogen and 21% oxygen.
- Light Scattering in Planetary Atmospheres: Rayleigh scattering causes blue skies and red sunsets on Earth; Mie scattering influences how clouds appear from space.
- Thermodynamics of Planetary Atmospheres: Studies energy and temperature changes; key processes include conduction, convection, and radiation, which affect weather and climate.
- Spectroscopy and Radiative Transfer: Essential for identifying atmospheric composition and how energy moves through atmospheres; indicates chemical processes and potential habitability on other planets.
Learn faster with the 12 flashcards about Planetary Atmospheres
Sign up for free to gain access to all our flashcards.
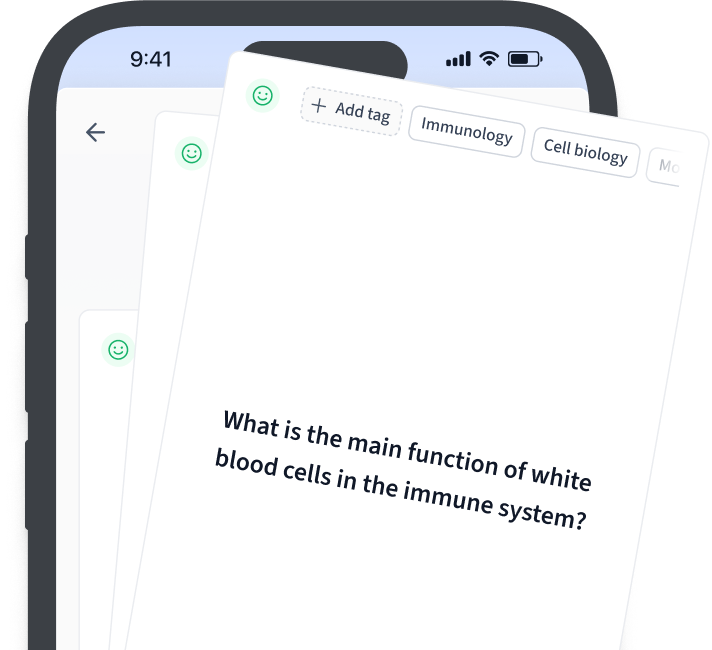
Frequently Asked Questions about Planetary Atmospheres
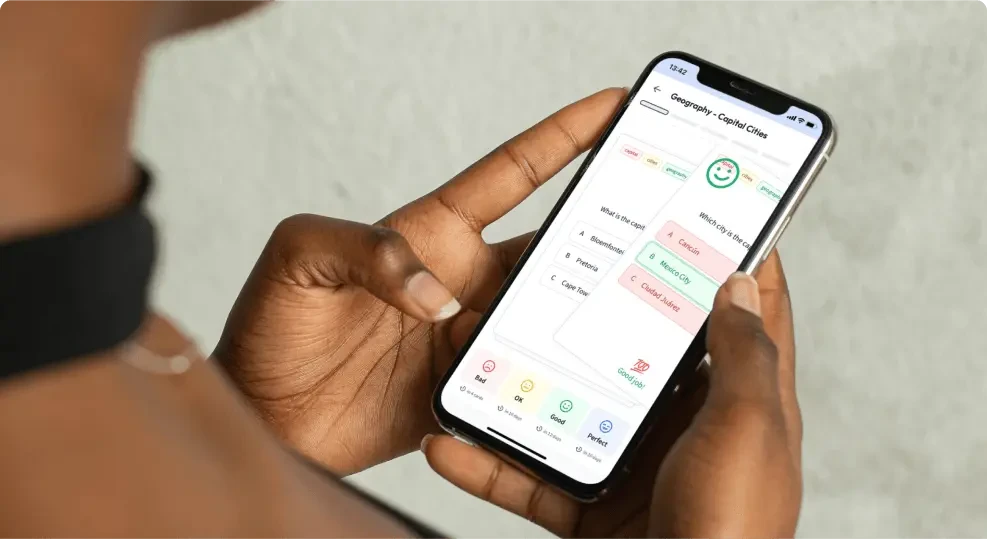
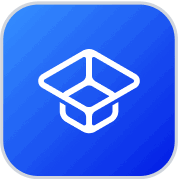
About StudySmarter
StudySmarter is a globally recognized educational technology company, offering a holistic learning platform designed for students of all ages and educational levels. Our platform provides learning support for a wide range of subjects, including STEM, Social Sciences, and Languages and also helps students to successfully master various tests and exams worldwide, such as GCSE, A Level, SAT, ACT, Abitur, and more. We offer an extensive library of learning materials, including interactive flashcards, comprehensive textbook solutions, and detailed explanations. The cutting-edge technology and tools we provide help students create their own learning materials. StudySmarter’s content is not only expert-verified but also regularly updated to ensure accuracy and relevance.
Learn more