Jump to a key chapter
Introduction to Pulse Detonation Engine
Pulse Detonation Engines (PDEs) represent a fascinating area of engineering that merges principles from various disciplines to create highly efficient propulsion systems. These engines are notable for their unique operation mode, which involves detonation waves to produce thrust, offering potential improvements over conventional propulsion technologies.
Pulse Detonation Engine: A propulsion technology that generates thrust by using a series of explosions or detonations within a tube. These detonations create shock waves that push against the back end of the engine, propelling the vehicle forward.
Unlike conventional jet engines that rely on continuous combustion, PDEs operate through the intermittent ignition of fuel-air mixtures, leading to pulses of high-speed exhaust gases. This method maximises the energy derived from each fuel unit and can significantly enhance engine efficiency and performance.
The concept of using detonation waves for propulsion might seem similar to how fireworks propel themselves into the sky, yet on a much more controlled and powerful scale.
History and Evolution of Pulse Detonation Engines
The development of Pulse Detonation Engines has evolved significantly since its initial conceptualisation. Early interest in PDE technology dates back to the first half of the 20th century, although tangible progress only emerged decades later due to technological limitations.
Important milestones in the development of PDEs:
- 1940s: Theoretical foundations laid out, but practical application was hindered due to the lack of advanced materials and understanding.
- 1980s: Resurgence of interest in PDE technology, spurred by advances in computational simulation and materials science.
- Early 2000s: Successful demonstrations of PDE-powered flight, marking a significant proof of concept for this propulsion method.
- Present: Research and development continue, focusing on refining the technology for commercial and military applications, with an emphasis on improving efficiency, reliability, and scalability.
PDEs are a prime example of how persistent engineering challenges can incubate groundbreaking solutions over time.
How Does a Pulse Detonation Engine Work?
Understanding the workings of a Pulse Detonation Engine (PDE) requires diving into the realms of physics and engineering. Unlike traditional jet engines, PDEs utilise the rapid, successive detonations of a fuel-air mixture to produce thrust. This approach offers potential benefits in terms of efficiency and simplicity.The key to the effectiveness of a PDE lies in its ability to convert chemical energy from fuel into mechanical energy (thrust) more directly and powerfully than conventional propulsion methods.
The Science Behind Pulse Detonation Wave Engine
The Pulse Detonation Engine operates on the principle of detonation rather than deflagration, which is used in most engines today. Detonation represents a supersonic combustion process in which a shock wave precedes the flame front, compressing the air-fuel mixture and leading to more efficient combustion.A critical aspect of PDE technology is the ability to generate and control these detonation waves, which travel faster than the speed of sound and create a series of high-energy pulses. By harnessing these pulses, PDEs can propel vehicles at high speeds.
Detonation vs. Deflagration: Detonation involves a supersonic combustion process characterised by a shockwave that pushes ahead of the combustion front, leading to high-efficiency combustion. Deflagration, in contrast, is a subsonic combustion wave, much slower and less efficient.
The mechanics of detonation are complex and involve precise dynamics between fuel, oxidiser, and initial energy input to achieve the transition from deflagration to detonation. This transition is critical and requires exact conditions of pressure, temperature, and mixture concentration.
Stages of Operation in a Pulse Detonation Jet Engine
The operation of a Pulse Detonation Jet Engine can be broken down into distinct stages, each critical for the engine's overall performance. These stages create a cyclical process that allows for the repetitive generation of thrust through detonation waves.Here’s a summary of the operational stages:
- Filling: The combustion chamber is filled with a mixture of fuel and air.
- Detonation: The mixture is then ignited to initiate a detonation wave.
- Expansion: High-temperature and high-pressure gases produced during detonation expand rapidly, creating thrust.
- Exhaust: The exhaust gases are expelled, and the cycle repeats.
Imagine filling a balloon and then suddenly releasing the air. The rapid exit of air propels the balloon in the opposite direction. Similarly, in a PDE, the rapid expansion of gases during the detonation and exhaust stages propels the engine forward.
The challenge in the efficient operation of a PDE lies in optimising each stage of the cycle. Factors such as the timing of fuel injection, the composition of the fuel-air mixture, and the method of initiating detonation are pivotal. Advanced materials and control systems are crucial to handle the extreme conditions generated during detonation, ensuring the engine's structural integrity and operational efficiency.
Pulse Detonation Engine Design
The design of a Pulse Detonation Engine (PDE) is central to its innovative approach to propulsion. By leveraging the power of controlled detonations, these engines achieve remarkable efficiency and power output compared to traditional propulsion systems. This efficiency comes from the PDE's unique components and the way they work together to generate thrust.
Key Components of a Pulse Detonation Engine
The design and functionality of a Pulse Detonation Engine are based on several key components. Each plays a crucial role in the engine's operation, enabling the unique detonation-based propulsion method that sets PDEs apart from other engine types.The following are the primary components involved in a PDE:
- Combustion chamber: Where the fuel-air mixture is detonated, generating high-pressure and high-velocity gases.
- Fuel injection system: Delivers fuel into the combustion chamber. The design of this system is critical for achieving the ideal fuel-air mixture.
- Detonation initiation system: Responsible for igniting the fuel-air mixture to start the detonation process. This can be achieved through various means, including electrical discharge.
- Exhaust system: Allows the high-pressure gases produced by the detonation to exit, creating thrust in the process.
The fundamental principle behind the PDE's efficiency relates to how quickly and effectively it can convert the chemical energy of the fuel into mechanical energy (thrust).
In the design of the combustion chamber, material selection becomes paramount due to the extreme temperatures and pressures encountered during detonation. Materials such as ceramics or specially formulated alloys are often used to withstand these conditions. Moreover, the geometry of the chamber is meticulously calculated to support efficient detonation wave propagation and to harness the maximum possible thrust from each detonation cycle.
Innovations in Pulse Detonation Engine Design
Recent years have seen a surge in innovations within Pulse Detonation Engine design, aiming at overcoming the technological barriers that have historically limited their widespread application. These innovations cover a broad spectrum, from advancements in materials technology to sophisticated fuel mixing and delivery systems.Some of the most significant innovations in PDE design include:
- Improved detonation initiation mechanisms, using laser ignition or microwave-enhanced techniques for more reliable and controlled detonation.
- Advanced computational fluid dynamics (CFD) models to better predict and enhance detonation behaviour within the engine.
- Development of adaptive fuel injection systems that can adjust the fuel-air mixture in real-time based on operating conditions.
- Use of high-temperature-resistant materials, such as ceramic matrix composites, to construct components that can withstand the extreme conditions inside a PDE.
For instance, the integration of laser ignition systems into PDE design represents a leap forward in reliability and control over the detonation process. Compared to traditional spark plug ignition, lasers can precisely deliver energy to initiate the detonation at the optimal location and moment, enhancing the engine's overall performance.
One of the frontier areas of innovation in PDE design is the exploration of fuel flexibility. This involves adapting the engine to efficiently burn a variety of fuels, including less conventional, more sustainable options such as biofuels or hydrogen. Achieving such flexibility could drastically reduce the carbon footprint of these engines and broaden their applications in both terrestrial and aerospace propulsion systems.
Types of Pulse Detonation Engines
Pulse Detonation Engines (PDEs) have emerged as a compelling area of research within the field of propulsion technology. Among the various designs explored, each type offers distinct mechanisms and advantages for generating thrust. The innovation in PDE design extends to how these engines initiate and utilise detonation waves for propulsion.
Rotating Pulse Detonation Engine
A Rotating Pulse Detonation Engine (RPDE) represents a unique take on traditional PDE technology. Unlike a conventional PDE, which operates by firing a series of static detonation waves through a linear tube, an RPDE utilises a rotary mechanism to continuously ignite fuel-air mixtures around a circular path.The fundamental structure of an RPDE includes a circular, annular combustion chamber where the detonations occur. This innovation enables the engine to generate continuous propulsion, mimicking a turbine-like operation but with the efficiency and power of detonation waves.
Rotating Pulse Detonation Engine (RPDE): An engine design that combines the principles of pulse detonation with a rotating mechanism. This setup allows for continuous detonation waves to be initiated in a circular pattern, creating a constant thrust output.
Example: Imagine a circular racetrack where explosions occur at specific intervals along the path. Each explosion propels the next phase of ignition, continuously driving the system forward. This is akin to how an RPDE operates, with each detonation wave contributing to the continuous rotation and thrust generation.
The RPDE brings several engineering challenges, particularly in synchronising detonation timing and managing thermal stresses within the rotating system. Innovations in materials science and combustion modelling are critical to overcoming these obstacles, making the RPDE a forefront of research in the quest for more efficient propulsion systems.
Advantages of Pulse Detonation Wave Engine Over Conventional Engines
Pulse Detonation Wave Engines (PDWEs) stand out from conventional engines due to their operational efficiency and potential for higher performance. These advantages are rooted in the fundamental differences in combustion dynamics between PDWEs and traditional jet or internal combustion engines.Here are some of the key advantages PDWEs offer over conventional engines:
- Higher Thermal Efficiency: The use of detonation waves allows for almost instantaneous combustion of the fuel-air mixture, leading to improved thermodynamic efficiency.
- Reduced Mechanical Complexity: PDWEs eliminate the need for many moving parts found in traditional engines, such as turbines and compressors, which can reduce maintenance requirements and improve reliability.
- Better Performance at High Speeds: The nature of detonation combustion makes PDWEs particularly suited for supersonic and hypersonic speeds, where conventional engines lose efficiency.
- Adaptability to Different Fuels: PDWEs can typically handle a broader range of fuels, including alternative and less conventional fuel sources, offering flexibility in operation.
For instance, the adaptability to different fuels means a PDWE could theoretically operate on a blend of traditional aviation fuel and biofuel, reducing its environmental impact while maintaining high performance.
The unique combustion method of PDWEs, harnessing the power of detonation rather than deflagration, is the key to their multiple advantages over traditional engines.
The transition from conventional combustion engines to PDWEs signifies a paradigm shift in propulsion technology. Research and development efforts focus on optimising the operating cycles, understanding the complex flow dynamics of detonation processes, and advancing materials technology to withstand the extreme conditions produced by detonations. Successful implementation of PDWE technology in practical applications could herald a new era in efficient, high-speed travel.
Pulse Detonation Engine - Key takeaways
- Pulse Detonation Engine (PDE): A propulsion technology utilising detonation waves within a tube to produce thrust, offering advancements over traditional engines.
- Detonation Waves: A supersonic combustion process with a shock wave ahead of the flame front, providing efficient combustion compared to subsonic deflagration used in other engines.
- Operational Stages: A PDE operates in cycles: Filling the combustion chamber with fuel-air mix, detonation to produce a wave, rapid gas expansion, and exhaust of gases.
- Design Considerations: PDEs require durable materials like ceramics, controlled fuel-air mixtures, and precise detonation initiation for maximum efficiency and thrust.
Rotating Pulse Detonation Engine (RPDE): A variation of PDE with a rotating mechanism, enabling continuous propulsion similar to turbine engines but leveraging the efficiency of detonation waves.
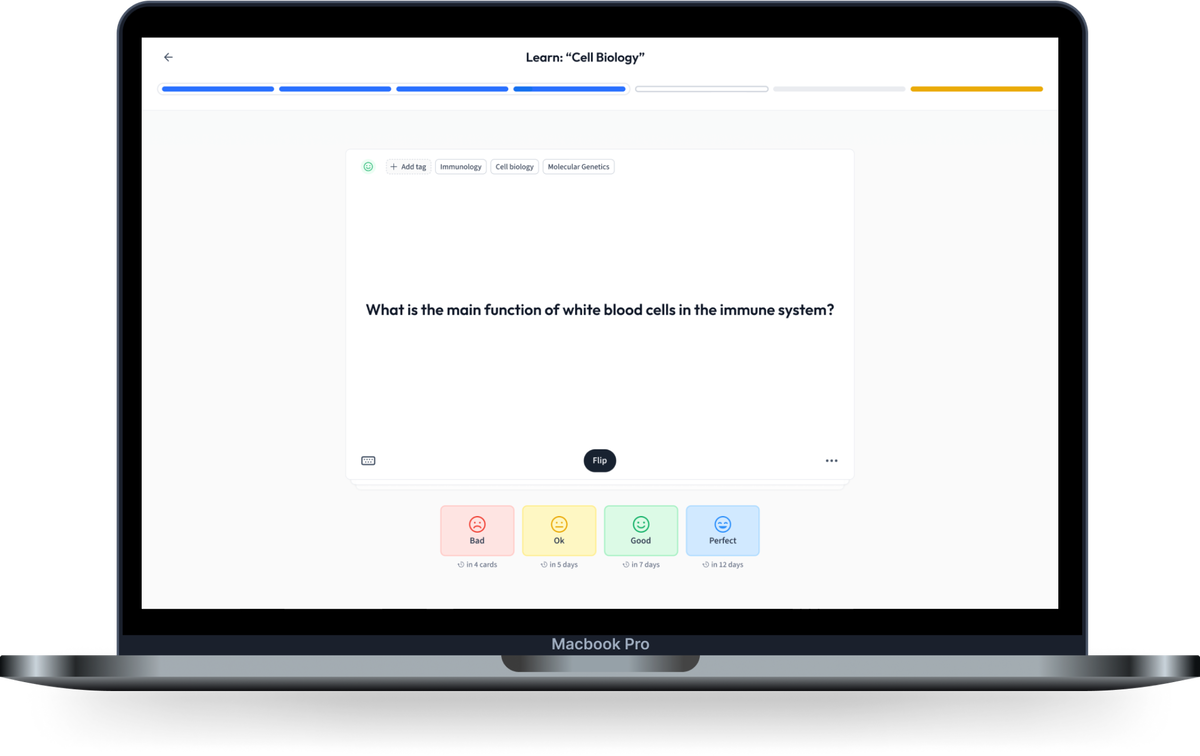
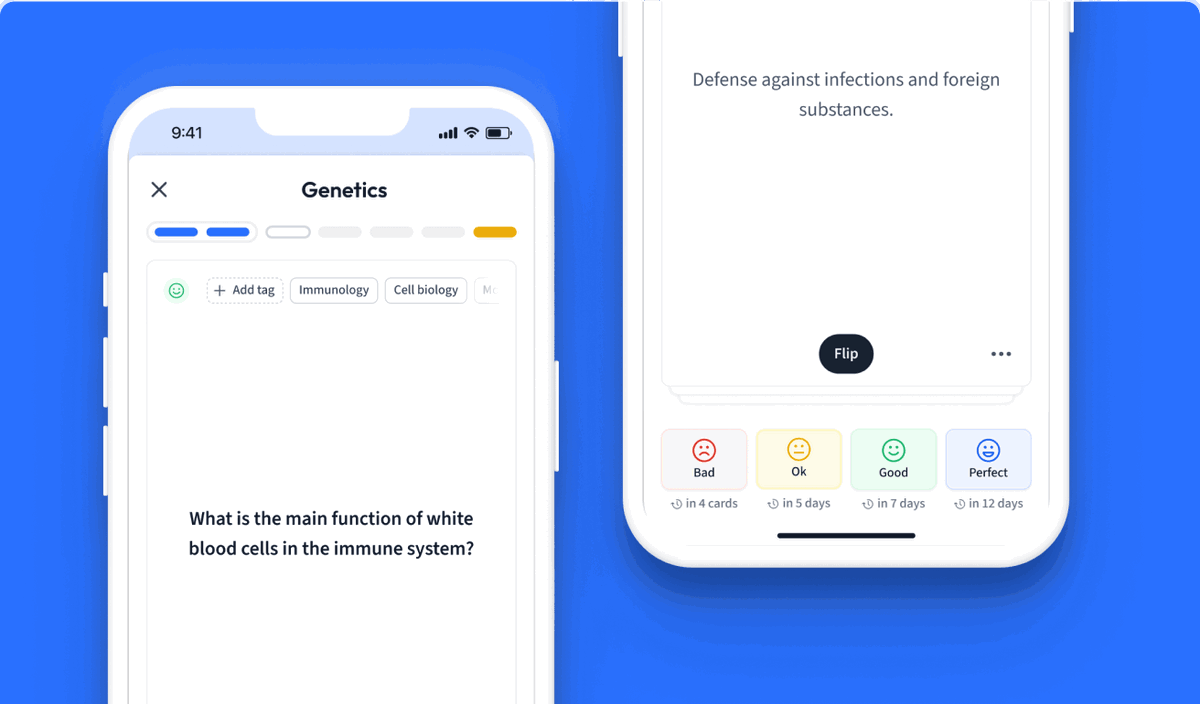
Learn with 12 Pulse Detonation Engine flashcards in the free StudySmarter app
Already have an account? Log in
Frequently Asked Questions about Pulse Detonation Engine
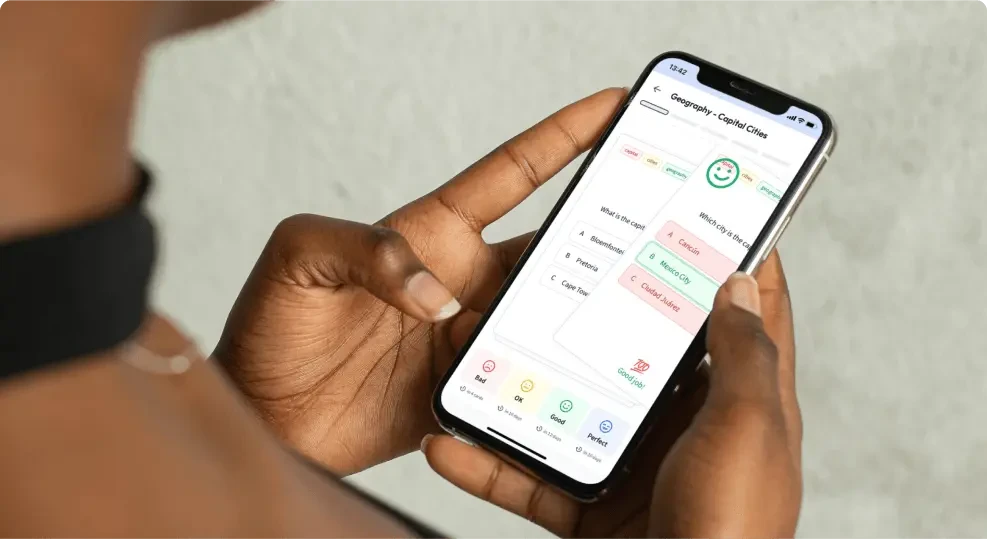
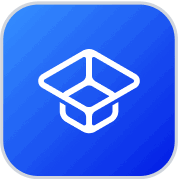
About StudySmarter
StudySmarter is a globally recognized educational technology company, offering a holistic learning platform designed for students of all ages and educational levels. Our platform provides learning support for a wide range of subjects, including STEM, Social Sciences, and Languages and also helps students to successfully master various tests and exams worldwide, such as GCSE, A Level, SAT, ACT, Abitur, and more. We offer an extensive library of learning materials, including interactive flashcards, comprehensive textbook solutions, and detailed explanations. The cutting-edge technology and tools we provide help students create their own learning materials. StudySmarter’s content is not only expert-verified but also regularly updated to ensure accuracy and relevance.
Learn more