Jump to a key chapter
Understanding Robust Control
Robust Control is a critical concept within the field of engineering concerned with ensuring that systems perform reliably under a wide range of conditions. Dive deep into this fascinating area, where precision and adaptability collide to create systems that can withstand uncertainty and variability.
What Is Robust Control? A Simple Explanation
At its core, Robust Control focuses on designing controllers that maintain system performance despite external disturbances or variations in system parameters. This versatility is essential for areas like aerospace, automotive systems, and any field that demands high reliability under unpredictable conditions.
Imagine your home heating system automatically adjusting to keep you comfortable, no matter how wildly the weather outside changes. That's Robust Control in action.
Robust Control: A branch of control theory that deals with the control of systems that are subject to uncertain
The Essentials of Robust Control for Beginners
Embarking on the journey of understanding Robust Control begins with several key concepts that form the foundation. These include the notions of system robustness, control design objectives, and the tools used to achieve robust performance. Grappling with these ideas is the first step towards mastering this indispensable field.
Among the tools leveraged in Robust Control is the H∞ method, which allows engineers to design control systems that can optimally reject disturbances and maintain performance across a broad spectrum of scenario. Likewise, μ-analysis is used for determining the stability of a control system when subject to parameter variations, further cementing the ties between theory and practical application.
For instance, consider an aircraft that must remain stable and controllable at various speeds and altitudes, despite the unpredictable nature of air currents and weather conditions. To ensure this level of robustness, engineers design control systems using principles of Robust Control. This involves creating algorithms that can quickly adjust the aircraft's flight controls in response to changing environmental conditions, maintaining stability and ensuring the safety of all on board.
Types of Robust Control Systems
Delving into the different types of robust control systems unveils how engineers equip various systems to tackle uncertainties, ensuring they perform optimally under a wide array of conditions. Each approach, whether it be linear, adaptive, or optimal, caters to specific scenarios and challenges, showcasing the versatility and depth of robust control as a field.
Exploring Linear Robust Control
Linear Robust Control focuses on systems described by linear equations, making it a foundational approach in control theory. This type involves designing controllers that can handle inaccuracies in the system model and external disturbances effectively. Tools like H∞ methods are commonly used here, optimising system performance across a preset range of conditions.
The beauty of linear robust control lies in its simplicity and the extensive mathematical foundation it is built upon. Algorithms developed under this approach are pivotal in industries where system models are well understood but subject to variable conditions, such as in manufacturing processes or electrical power systems.
Introduction to Adaptive Robust Control
Adaptive Robust Control takes a dynamic approach by allowing the system to adjust its parameters in real-time to cope with uncertainties. This adaptability is particularly valuable in environments where conditions change unpredictably, requiring the system to learn and evolve continuously.
Employing techniques such as model reference adaptive control (MRAC) and adaptive backstepping, this approach ensures the control system can maintain its objectives despite variations in external and internal parameters. Applications range from robotics to aerospace, where adaptability and resilience are paramount.
The Basics of Optimal and Robust Control
Optimal and Robust Control combines the objectives of optimising performance while being resilient to disturbances. It utilises an optimization framework to determine the best control action that minimises a cost function, considering both the performance index and robustness against model uncertainties.
The application of this method is broad, covering systems where efficiency and reliability are critical. Utilizing tools such as LQR (Linear Quadratic Regulator) for designing optimal controllers under linear system assumptions, engineers can carefully balance the trade-offs between performance and robustness. This methodology is integral to designing systems that function reliably in unpredictable environments, like autonomous vehicles and advanced communication networks.
Principles of Robustness Control in Engineering
Robustness control in engineering ensures that systems continue to operate effectively despite changes and uncertainties in their environments. This discipline involves creating control strategies that are resilient to variations, from minor fluctuations to significant disturbances.
Key Concepts in Robust Control Definition
Robust Control: A subset of control theory that deals with designing control systems that can maintain desired performance in the presence of uncertainties.
Robustness in control systems is underpinned by several crucial concepts, including system stability, performance robustness, and uncertain model dynamics. Understanding these elements is essential for designing systems that can adapt and withstand a range of operational scenarios.
- System Stability: Refers to the ability of a system to return to its equilibrium state after a disturbance.
- Performance Robustness: The system's capacity to maintain performance levels in the face of parameter variations or external disturbances.
- Uncertain Model Dynamics: This involves the variations in system models that arise due to unpredictable changes in the system's environment or configuration.
For example, consider an unmanned aerial vehicle (UAV) navigating through varying weather conditions. Robust control methods are applied to ensure that irrespective of unexpected wind gusts or temperature changes, the UAV maintains its flight path and stabilisation, highlighting the critical nature of robust design in practical applications.
The Importance of Robustness in Control Systems
The implementation of robust control principles is vital across numerous engineering disciplines, safeguarding systems against the unpredictable. From aerospace to consumer electronics, the methodology's breadth and adaptability have established it as a cornerstone of modern engineering.
An often-overlooked aspect of robustness is its role in extending the lifespan of systems by reducing the wear and tear caused by oscillatory or unstable operations.
Delving deeper into the subject, the mathematical foundation of robust control involves formulating and solving problems where system parameters are not fixed but vary within known bounds. The approach employs tools such as ext{H}_ ext{ ext{∞}} norms and Lyapunov functions, which provide frameworks for analysing and ensuring stability and performance despite uncertainties. For instance, the ext{H}_ ext{ ext{∞}} method focuses on optimising the worst-case scenario performance of a system, making it highly effective for systems operating under severe uncertain conditions. This methodological rigour underscores why robust control is indispensable in creating resilient and dependable systems.
Implementing Robust Control in Aerospace Engineering
Aerospace engineering constantly challenges the boundaries of what's possible, ensuring aircraft and spacecraft perform safely and efficiently in various unpredictable environments. Implementing robust control in this sector is critical for managing the dynamic and uncertain conditions faced during flight.
Applications of Robust Control in Aerospace
Robust control systems are integral to modern aerospace engineering, offering solutions that enhance the stability, safety, and performance of both aircraft and spacecraft. By ensuring that these complex machines can handle unexpected disturbances, such as turbulent weather or system parameter variations, robust control helps to maintain optimal operational conditions.
- Flight Control: Adaptive robust control systems adjust in real-time to maintain stability and control of the aircraft during flight, compensating for aerodynamic uncertainties.
- Engine Control: Enhancing engine efficiency and reliability under various conditions, robust control systems optimise performance while managing unexpected disturbances.
- Spacecraft Attitude Control: In the vacuum of space, where external disturbances can unpredictably affect trajectory and orientation, robust control is pivotal for maintaining the correct attitude and orbit of spacecraft.
Consider how a spacecraft needs to adjust its course in real-time to counteract unforeseen gravitational forces, showcasing the adaptability of robust control in aerospace applications.
Challenges in Aerospace Robust Control Solutions
While robust control provides numerous benefits to aerospace engineering, its implementation comes with significant challenges. These include the complexity of modeling aerospace systems accurately, ensuring the robustness of control algorithms against extreme environmental conditions, and maintaining system performance over a wide range of operational scenarios.
- Modeling Uncertainties: Accurately predicting how changes in speed, altitude, or atmospheric conditions will affect aircraft performance can be incredibly challenging.
- Environmental Extremes: From the intense cold and vacuum of space to the turbulent atmosphere re-entry conditions, aerospace systems must operate reliably under some of the most harsh and unpredictable environments.
- Algorithm Complexity: Developing and verifying algorithms that can adapt to and manage a vast array of potential disturbances requires extensive testing and validation.
The intricacies of designing robust control systems for aerospace applications highlight the intersection of theoretical knowledge and practical engineering. For example, when formulating control laws for aircraft stability, engineers must account for non-linear dynamics, such as \[x^2 + \frac{1}{x} = y\], representing the aircraft's response to control inputs across different flight regimes. This complexity necessitates a deep understanding of both control theory and the unique dynamics of aerospace vehicles, underscoring the critical role of robust control in advancing aerospace technology.
Robust Control - Key takeaways
- Robust Control Definition: A field of engineering focused on designing controllers that ensure system performance remains stable despite external disturbances and variations in system parameters.
- H∞ Method: A tool in robust control that optimises control systems to reject disturbances and maintain performance across varied scenarios.
- Linear Robust Control: Concerns systems described by linear equations and involves designing controllers to handle inaccuracies and disturbances effectively.
- Adaptive Robust Control: A dynamic control approach allowing real-time parameter adjustment to cope with uncertainties, using techniques like MRAC and adaptive backstepping.
- Optimal and Robust Control: A method that uses an optimisation framework to balance performance with resilience against disturbances, employing tools such as LQR.
Learn faster with the 12 flashcards about Robust Control
Sign up for free to gain access to all our flashcards.
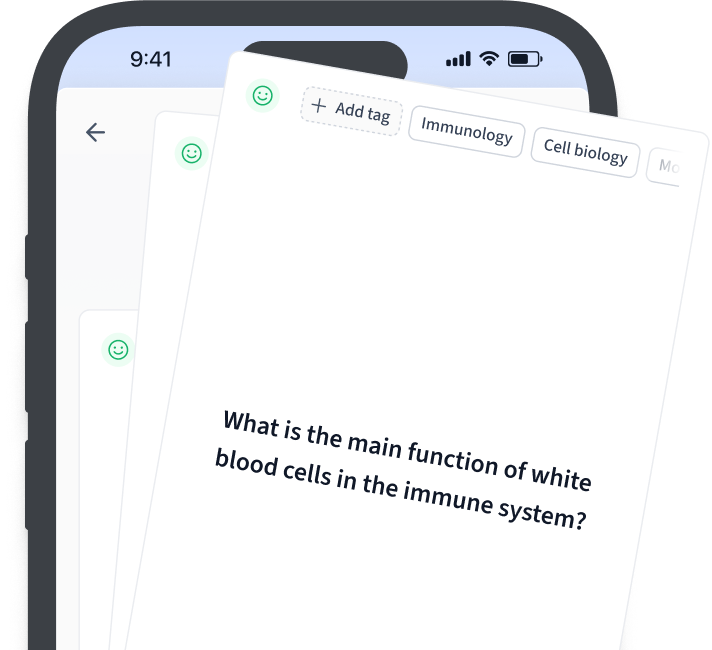
Frequently Asked Questions about Robust Control
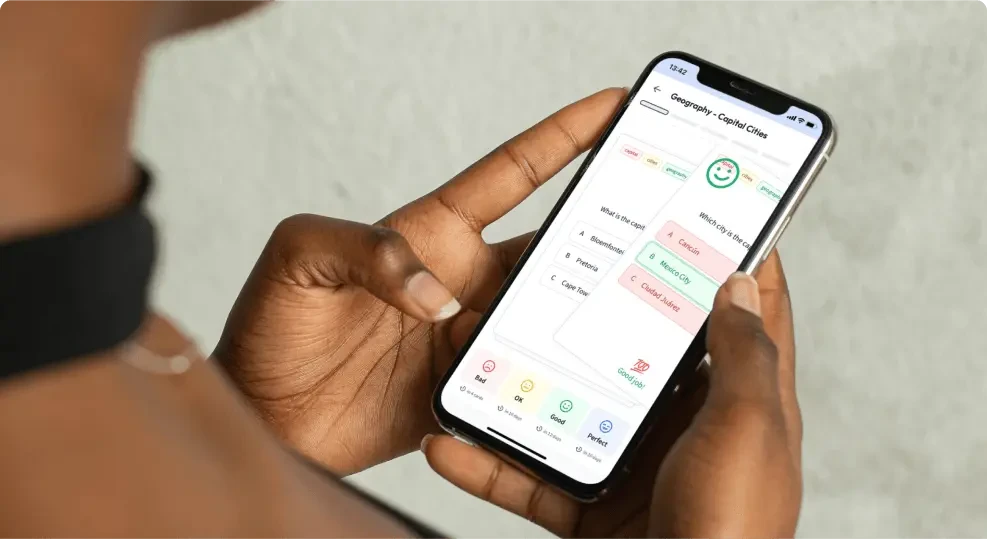
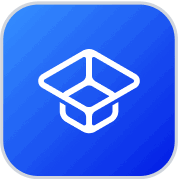
About StudySmarter
StudySmarter is a globally recognized educational technology company, offering a holistic learning platform designed for students of all ages and educational levels. Our platform provides learning support for a wide range of subjects, including STEM, Social Sciences, and Languages and also helps students to successfully master various tests and exams worldwide, such as GCSE, A Level, SAT, ACT, Abitur, and more. We offer an extensive library of learning materials, including interactive flashcards, comprehensive textbook solutions, and detailed explanations. The cutting-edge technology and tools we provide help students create their own learning materials. StudySmarter’s content is not only expert-verified but also regularly updated to ensure accuracy and relevance.
Learn more