Jump to a key chapter
What is Rocket Science?
Rocket Science, often perceived as a complex field, involves the study and application of physics, engineering, and mathematics to create and operate rockets. It plays a pivotal role in space exploration, satellite deployment, and even in certain military applications. Understanding rocket science opens up a world of innovation and exploration beyond our planet.
The Fascinating World of Rocket Science Explained
The realm of rocket science encompasses more than just launching spacecraft into orbit. It involves understanding the principles that make space travel possible. From the physics of propulsion to the engineering challenges of spacecraft design, rocket science is at the forefront of human exploration and technological advancement. It's about overcoming the Earth's gravity and venturing into the unknown depths of space.At its core, rocket science applies fundamental physics laws, such as Newton's third law of motion, to propel vehicles through the atmosphere and into space. The field is interdisciplinary, merging principles from aerodynamics, astrodynamics, and material science to develop vehicles capable of withstanding the harsh conditions of space travel.
Did you know? Rocket science isn't just for rockets. Its principles are applied in designing jet engines, fireworks, and even some types of automotive technologies.
Rocket Science Fundamentals Explained
Rocket science can be broken down into several fundamental concepts that provide the foundation for this field. Understanding these basics is essential for grasping how rockets break free from Earth's gravitational pull and navigate through space:
- Propulsion: This involves generating thrust to lift and accelerate the rocket. It's achieved through the expulsion of mass (fuel) at high speed, adhering to Newton's third law.
- Aerodynamics: The study of how gases interact with moving bodies. Aerodynamics is crucial for designing a rocket's shape, allowing it to efficiently pass through the Earth's atmosphere.
- Orbit mechanics: Understanding the paths that objects follow in space is vital for placing and keeping satellites in orbit around Earth or sending spacecraft to other planets.
- Structural integrity: Rockets must be designed to withstand immense forces during launch, flight, and re-entry into the Earth's atmosphere without breaking apart.
Rocket: A vehicle designed to propel itself by ejecting exhaust from one end, based on conservation of momentum, capable of travelling through Earth's atmosphere or in the vacuum of space.
One notable example of rocket science in action is the Saturn V rocket, used during the Apollo missions to send humans to the moon. It demonstrated key rocket science principles, such as staging, to effectively break free of Earth's gravitational pull and navigate to the Moon and back.
The challenge of reusability is a current focus in rocket science, aiming to reduce the cost of access to space. Traditionally, rockets were used only once. However, companies like SpaceX and Blue Origin have pioneered reusable rocket technologies, marking a significant shift towards more sustainable and cost-effective space exploration. These efforts showcase not only the technical complexities involved in rocket science but also the ongoing innovation driving the field forward.
Rocket Science Equation
The Rocket Science Equation, often symbolized as the rocket equation, is a fundamental piece of understanding how rockets achieve motion. This equation is crucial for anyone delving into the world of rocket science and space exploration.
Unlocking the Mystery of Rocket Propusion Fundamentals
At the heart of rocket propulsion fundamentals is the principle of action and reaction, as stated in Newton's third law. Rockets move forward by expelling their exhaust in the opposite direction at high speed. This core principle is mathematically captured in the rocket equation, which provides the theoretical basis for rocket design and performance analysis.Understanding this fundamental concept helps in comprehending how rockets can travel from the Earth's surface to outer space. Despite the apparent complexity, the basics of rocket propulsion are rooted in straightforward physical principles that govern motion and force.
Think of rocket propulsion like blowing up a balloon and letting it go. The air rushing out of the balloon propels it in the opposite direction.
Key Equations in Rocket Science
Rocket Science heavily relies on several key equations to predict and enhance the performance of rockets. The most significant among these is the Tsiolkovsky rocket equation, named after the Russian scientist Konstantin Tsiolkovsky, who first proposed it. This equation precisely relates the change in velocity of the rocket to the characteristics of the propellant used. Other key equations include those governing orbital dynamics, such as Kepler's laws for planetary motion and Newton's law of universal gravitation.Beyond these, equations relating to fluid dynamics, thermodynamics, and structural mechanics also play essential roles in the design and analysis of rockets.
Tsiolkovsky Rocket Equation: A fundamental equation that describes the motion of vehicles that follow the basic principle of a rocket: \(\Delta v = I_{sp} \cdot g_0 \cdot \ln\left(\frac{m_0}{m_f}\right), where \(\Delta v\) is the change in velocity, \(I_{sp}\) is the specific impulse of the propellant, \(g_0\) is the acceleration due to gravity at the Earth's surface, \(\ln\) is the natural logarithm, \(m_0\) is the initial total mass, and \(m_f\) is the final total mass.
An example of the Tsiolkovsky rocket equation in action is the Saturn V's first stage: with a specific impulse of about 260 seconds, and a mass ratio (the ratio of its initial fully fueled mass to its mass after burning its fuel) of around 10, it achieved a \(\Delta v\) of about 2.4 km/s, contributing significantly to reaching the required velocity for Earth orbit.
One intriguing aspect of the Tsiolkovsky rocket equation is its implication for interplanetary travel. It illustrates the exponential nature of the fuel requirement relative to the \(\Delta v\) needed for missions beyond Earth's orbit. This explains why multi-stage rockets are necessary for space exploration, as each stage can significantly contribute to the overall \(\Delta v\) while shedding the dead weight of used-up fuel tanks, making the journey more efficient.
Rocket Aerodynamics Principles
Rocket aerodynamics principles are fundamental to understanding how rockets navigate through the Earth's atmosphere and into outer space. These principles govern the behaviour of rockets under the forces of air and are critical for the successful design and operation of any spacecraft.
Understanding the Basics of Rocket Aerodynamics
Aerodynamics refers to the way air moves around things. For rockets, which are designed to travel at high speeds through the atmosphere before reaching space, understanding aerodynamics is crucial. The main aerodynamic forces acting on a rocket during flight are drag, which acts in the opposite direction to the motion; lift, which can act in any direction; and weight, which acts downwards due to gravity. Effective rocket design aims to minimise drag while maximising lift and controlling weight.Rocket aerodynamics are also significantly influenced by the rocket's shape, angle of attack (the angle between the direction of the rocket's velocity and its longitudinal axis), and its speed. Under the umbrella of these responsibilities, engineers use fluid dynamics, a branch of physics that describes the behaviour of fluids (liquids and gases), to predict and manage these forces effectively.
Drag: The aerodynamic force opposing an object's motion through a fluid (such as air). For rockets, drag is a critical factor that affects fuel consumption and trajectory.
The shape of a rocket is streamlined to reduce drag, allowing it to move more efficiently through the atmosphere.
How Aerodynamics Influence Rocket Design
The influence of aerodynamics on rocket design cannot be overstated. Beyond affecting speed and fuel efficiency, aerodynamics can significantly impact a rocket's stability and control systems. To ensure a successful mission, rocket engineers must carefully consider several aerodynamic factors during the design phase. These include:
- Shape and Size: The rocket's body and fins are designed to minimise drag and optimise lift. This is why most rockets have a streamlined shape, tapering towards the top.
- Materials: The choice of materials affects the rocket's ability to withstand aerodynamic heating and pressure during flight.
- Control Surfaces: Fins and other control surfaces aid in steering the rocket and maintaining its stability during ascent.
Furthermore, the exact positioning of a rocket's centre of gravity and centre of pressure is crucial for stability. These two points must be carefully calculated and designed to prevent the rocket from tumbling or spinning uncontrollably.
A notable example of aerodynamics in rocket design is the distinct shape of the NASA Space Shuttle's external tank and solid rocket boosters. Their design minimised air resistance during ascent, proving the importance of aerodynamics in efficient space vehicle design.
Supersonic and hypersonic speeds introduce complex aerodynamic challenges due to the formation of shockwaves. Rockets and spacecraft entering or exiting the atmosphere at these speeds undergo severe aerodynamic heating, necessitating the development of heat-resistant materials and innovative cooling mechanisms. The study of aerodynamics at these extreme conditions continues to push the boundaries of material science and engineering, highlighting the intricate relationship between rocket design and its aerodynamic performance.
Rocket Engineering Basics
Rocket engineering combines the principles of physics, mathematics, and engineering to develop, test, and launch vehicles that can travel through Earth's atmosphere and into space. This field is vital for space exploration, satellite communication, and in some cases, defence. By understanding the basics of rocket engineering, you unlock insights into how humanity reaches beyond our planet.
Introduction to Rocket Engineering
Rocket engineering is not just about building rockets; it's about solving the complex challenges of propelling a vehicle out of Earth's atmosphere and into space. It involves a multifaceted approach that combines various scientific disciplines to design rockets that can withstand the immense forces encountered during launch, orbit, and re-entry.At its core, rocket engineering requires a deep understanding of propulsion, aerodynamics, material science, and structural integrity. Engineers must also consider factors such as fuel efficiency, safety, and the environmental impact of rocket launches. As space exploration evolves, rocket engineering continues to push the boundaries of what's possible, leveraging technological advances to achieve greater successes.
Rocket engineering is as much about overcoming earthly challenges as it is about exploring space. The laws of physics that dictate how things move on Earth also govern motion in the cosmos.
The Essential Elements of Rocket Science
The field of rocket science is built on several foundational elements that ensure the successful design, launch, and operation of rockets. These elements include:
- Propulsion: The mechanism by which a rocket moves through space, typically achieved by the expulsion of exhaust gases.
- Aerodynamics: The study of how gases interact with moving objects, crucial for designing a rocket's shape and structure to minimise drag and maximise efficiency.
- Structural engineering: Ensuring the integrity of a rocket's structure to withstand the forces experienced during launch and spaceflight.
- Navigation and control systems: Systems that guide a rocket on its course, including during liftoff, in orbit, and upon re-entry.
- Thermal management: Managing the extreme temperatures encountered during different phases of a mission to protect the rocket and its payload.
Rocket Propulsion: A means of moving a rocket or spacecraft by expelling part of its mass, in the form of a high-speed exhaust, to take advantage of the reaction force produced in accordance with Newton's third law of motion.
The Falcon Heavy, developed by SpaceX, is an example of advanced rocket engineering leveraging multiple elements of rocket science. Featuring a two-stage launch vehicle design, it utilises liquid oxygen and RP-1 fuel for its propulsion system, demonstrating high efficiency and reusability in modern rocketry.
One of the fascinating challenges in rocket engineering is achieving orbital velocity. This requires not only powerful propulsion systems but also meticulously designed trajectories that leverage the Earth's rotation and gravitational pull. Achieving and maintaining the precise speed and altitude necessary for an orbit involves complex calculations and constant adjustments from navigation and control systems. This intricacy embodies the marriage of theoretical physics and practical engineering that defines the field of rocket science.
Rocket Science - Key takeaways
- Rocket Science is an interdisciplinary field involving physics, engineering, and mathematics to develop rockets for space exploration, satellite deployment, and military applications.
- The Rocket Science Equation, or Tsiolkovsky rocket equation, is key to understanding rocket motion, relating the change in velocity to the propellant's characteristics.
- Rocket propulsion fundamentals are based on Newton's third law, with thrust generated by expelling mass to lift and accelerate the rocket.
- Rocket aerodynamics principles include the study of drag, lift, and weight, and their impacts, which are critical for efficient rocket design and navigation through Earth's atmosphere.
- Rocket engineering basics encompass propulsion, aerodynamics, structural engineering, navigation and control systems, and thermal management for successful space travel.
Learn faster with the 12 flashcards about Rocket Science
Sign up for free to gain access to all our flashcards.
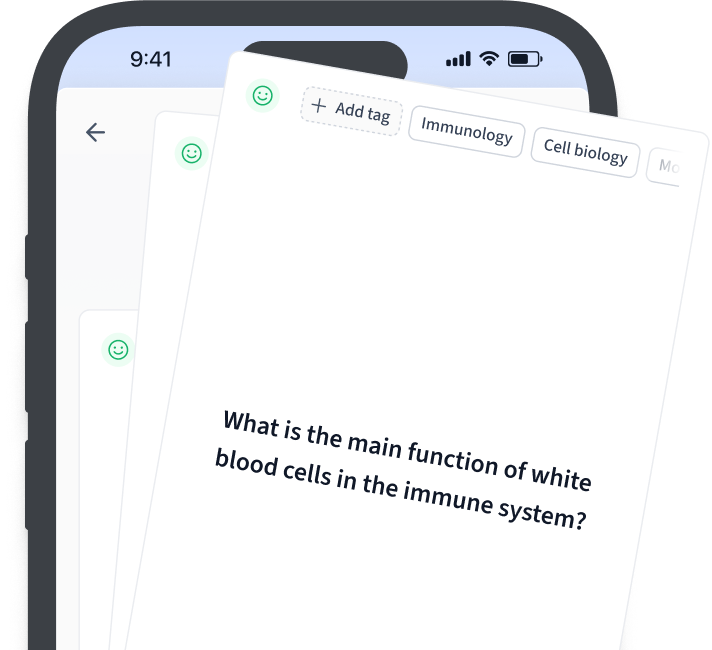
Frequently Asked Questions about Rocket Science
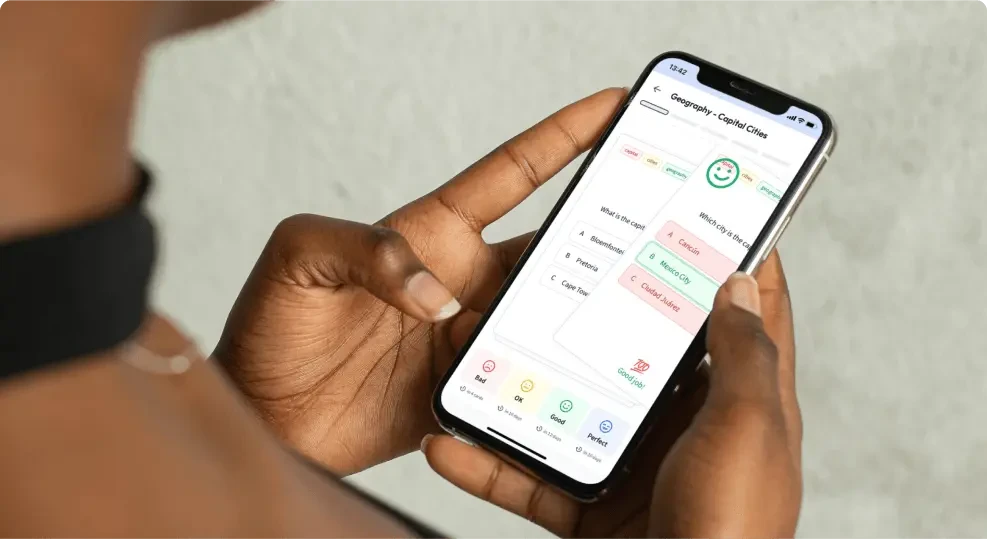
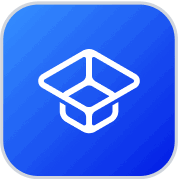
About StudySmarter
StudySmarter is a globally recognized educational technology company, offering a holistic learning platform designed for students of all ages and educational levels. Our platform provides learning support for a wide range of subjects, including STEM, Social Sciences, and Languages and also helps students to successfully master various tests and exams worldwide, such as GCSE, A Level, SAT, ACT, Abitur, and more. We offer an extensive library of learning materials, including interactive flashcards, comprehensive textbook solutions, and detailed explanations. The cutting-edge technology and tools we provide help students create their own learning materials. StudySmarter’s content is not only expert-verified but also regularly updated to ensure accuracy and relevance.
Learn more