Jump to a key chapter
What is Sonic Boom Analysis?
Sonic boom analysis is the study of the shock waves produced when an object moves through the air faster than the speed of sound. It encompasses a range of disciplines, including physics, fluid dynamics, and aerospace engineering, to understand and mitigate the impacts of these shock waves on the environment, structures, and human wellbeing.
Key Concepts in Sonic Boom Physics Principles
- Speed of Sound: The speed at which sound waves travel through a medium, such as air, which is approximately 343 meters per second (1,235 km/h) at sea level and at 20°C.
- Supersonic Speed: Any speed that exceeds the speed of sound in the medium through which the object is moving.
- Shock Waves: Rapid pressure increases that occur when an object moves through a medium at a speed faster than the speed of sound, creating a conical wavefront known as a Mach cone.
- Mach Number: A dimensionless quantity representing the ratio of the speed of an object to the speed of sound in the surrounding medium. For instance, Mach 1 is the speed of sound, Mach 2 is twice the speed of sound, and so forth.
Sonic Boom: A sonic boom occurs when an object moves through the air at a speed greater than the speed of sound, causing a high-energy shock wave. This shock wave produces a loud, thunder-like noise that can be heard over large areas and can cause damage to structures and distress to wildlife and humans.
A classic example of a sonic boom can be observed in military aircraft, such as fighter jets, which fly at supersonic speeds. As the aircraft exceeds the speed of sound, it produces a sonic boom that can be heard on the ground as a loud explosion.
Did you know? The Concorde, a British-French turbojet-powered supersonic passenger airliner, was famous not just for its speed but also for the sonic booms it generated while cruising at supersonic speeds over the ocean.
The Role of Sonic Boom Analysis in Aerospace Engineering
Sonic boom analysis plays a critical role in aerospace engineering, particularly in the design and development of supersonic aircraft and spacecraft. Engineers employ this analysis to:
- Minimize the impact of shock waves on the environment and built structures.
- Improve public perception and acceptance of supersonic travel by reducing the noise and vibration felt on the ground.
- Comply with regulations regarding noise pollution and protect wildlife affected by sonic booms.
- Enhance the comfort and safety of aircraft passengers and crew by minimizing the effects of shock waves on aircraft structural integrity.
Low-Boom Flight Demonstration: One of the most interesting advancements in sonic boom mitigation comes from the Low-Boom Flight Demonstration (LBFD) project by NASA. This project aims to design, build, and fly a piloted, supersonic X-plane that produces sonic booms so quiet they hardly register on the ground. The success of the LBFD could revolutionize supersonic travel, making it more acceptable and widespread by significantly lowering the noise pollution associated with sonic booms.
Mathematical Modelling of Sonic Booms
Mathematical modelling of sonic booms offers a systematic approach to understanding and predicting the impact of supersonic travel on the environment and human structures. Utilising advanced mathematics and computational fluid dynamics, it encompasses the creation of theoretical models to simulate the complex interactions between shock waves and various mediums.This branch of analysis is pivotal for the development of quieter supersonic aircraft, aiming to mitigate the negative effects of sonic booms through design optimisation and technological innovations.
The Process Behind Mathematical Modelling of Sonic Booms
The process of mathematical modelling of sonic booms involves several key steps:
- Definition of the problem space, including the geometry of the object causing the sonic boom and the characteristics of the medium through which the shock wave propagates.
- Derivation of governing equations based on fluid dynamics and acoustics principles to describe the behaviour of shock waves.
- Application of numerical methods to solve these complex equations, often involving differential equations that describe the pressure and density changes in the shock wave.
- Simulation of various flight scenarios to predict sonic boom patterns under different conditions.
Computational Fluid Dynamics (CFD): A crucial tool in the mathematical modelling of sonic booms, CFD allows engineers and scientists to visualise and predict the behaviour of shock waves as they propagate through different media. Advanced CFD software uses millions of data points to simulate the interaction between the shock wave and the atmosphere, offering insights into the potential impact of sonic booms on the environment and human structures. These simulations are pivotal in designing aircraft that minimise sonic boom impact.
Applying Mathematical Models to Predict Sonic Boom Effects
The use of mathematical models to predict sonic boom effects involves the application of theoretical models to real-world scenarios:
- Assessing the environmental impact of supersonic flight, particularly on regions overflown by supersonic aircraft.
- Evaluating potential structural damage to buildings and other infrastructures due to the intensity and frequency of sonic booms.
- Designing flight paths that minimise sonic boom exposure to populated areas, using predictive models to simulate sonic boom footprints.
- Informing policy and regulatory frameworks by providing quantifiable data on the impact of sonic booms, thus supporting the development of sustainable supersonic travel.
Innovations in aircraft design, such as variable geometry wings and optimised fuselage shapes, are largely driven by insights gained from mathematical models of sonic booms.
Sonic Boom Reduction Techniques
Exploring sonic boom reduction techniques is a crucial aspect of contemporary aerospace engineering. This focus seeks to mitigate the environmental and societal impacts of supersonic flights by employing advanced technologies and innovative aircraft designs.
Innovations in Sonic Boom Reduction Technologies
Innovations in sonic boom reduction technologies have led to remarkable advancements in aerospace engineering. Key developments include:
- Adaptive Materials: Incorporation of materials that can change shape in response to environmental conditions to minimise shockwaves.
- Quiet Supersonic Technology (QueSST): NASA's initiative to design aircraft with significantly lower sonic boom levels, aiming for a soft 'thump' rather than a disruptive boom.
- Advanced Aerodynamic Designs: Aircraft shapes that distribute shock waves more evenly, reducing the intensity of sonic booms on the ground. These designs include elongated noses and optimised wing configurations.
Application of Metamaterials: A cutting-edge approach involves the use of metamaterials in aircraft design. These artificially engineered materials are structured to control, direct, and manipulate wave fronts of sound, potentially redirecting or significantly weakening the strength of sonic booms. The application of these materials in critical parts of an aircraft, such as the nose and wings, offers a promising route to drastically reduce the impact of sonic booms.
The Impact of Aircraft Design on Sonic Boom Intensity
The design of an aircraft significantly influences the intensity of the sonic boom it generates. Factors such as the aircraft's shape, weight, and speed play a crucial role in determining the characteristics of the sonic boom produced:
- Aircraft Shape: Sleek, streamlined designs with longer noses can help mitigate boom intensity by influencing the way shock waves coalesce.
- Weight Distribution: Optimising the placement of mass within the aircraft can affect how shock waves propagate and interact, potentially reducing ground-level impact.
- Flight Altitude and Speed: Adjusting the cruising altitude and speed can minimise the boom's intensity felt on the ground, with higher altitudes generally causing less disruption.
Recent developments have seen aircraft designs that aim to 'smooth out' or 'stretch' the shock wave, converting the traditional loud boom into a series of softer, less intrusive sounds.
The Environmental Impact of Sonic Booms
Sonic booms, the powerful shockwaves produced when an object travels through the air faster than the speed of sound, have significant environmental impacts. From disturbing wildlife to affecting human communities, understanding and mitigating these effects are vital for sustainable supersonic travel.This exploration delves into the fundamental causes behind sonic booms, their observed effects on the environment, and the strategies currently being developed to reduce their impact.
Analysing Sonic Boom Causes and Effects on the Environment
Sonic booms result from the rapid compression of air molecules ahead of a supersonic object, creating a shockwave that travels to the ground as a loud, disruptive boom. The environmental impact of these phenomena is considerable, influencing both terrestrial and aquatic ecosystems.Effects on the environment include:
- Disturbance to wildlife, potentially altering migration patterns, breeding activities, and feeding behaviours.
- Damage to structures, including buildings and historical landmarks, from the intense vibrations caused by shockwaves.
- Impact on human health and well-being, through noise pollution that can lead to stress-related illnesses and decreased quality of life in affected areas.
Impact on Marine Life: Sonic booms are not just limited to terrestrial environments; they can also affect marine ecosystems. Studies have shown that shockwaves can propagate into water bodies, causing potential harm to marine species. Sudden changes in water pressure can disorient fish, disturb marine mammal communication, and even lead to physical harm in extreme cases.
The effects of sonic booms are more pronounced in areas with frequent supersonic flights, making it a significant concern for communities near military bases or supersonic test flight paths.
Strategies to Mitigate the Environmental Effects of Sonic Booms
Mitigating the environmental impact of sonic booms is an evolving field, combining technology, policy, and community engagement. Effective strategies include:
- Advancements in Aircraft Design: Developing aircraft with aerodynamic shapes that disperse shockwaves more evenly across the sky, lessening the intensity of sonic booms when they reach the ground.
- Operational Restrictions: Implementing flight altitude and route restrictions to avoid supersonic travel over sensitive areas, such as national parks or residential communities.
- Regulatory Policies: Establishing clear guidelines and noise limits for supersonic aircraft, encouraging the industry to invest in quieter technologies.
One notable success story in this area is NASA's X-59 QueSST aircraft, designed to fly faster than the speed of sound with significantly reduced noise. By carefully shaping the aircraft's body, NASA aims to transform the disruptive boom into a gentle sonic 'thump', minimising its environmental footprint.
Sonic Boom Analysis - Key takeaways
- Sonic Boom Analysis Definition: The examination of shock waves created when an object travels through air faster than sound, involving physics, fluid dynamics, and aerospace engineering to lessen environmental and structural impacts.
- Sonic Boom Physics Principles: Fundamental concepts include the speed of sound (343 m/s at sea level and 20°C), supersonic speed (any speed above the speed of sound), shock waves (rapid pressure increases forming a Mach cone), and the Mach number (speed ratio of an object to the speed of sound).
- Mathematical Modelling of Sonic Booms: Utilises advanced mathematics and computational fluid dynamics to predict sonic boom impacts, leading to quieter aircraft design through optimisation and technological innovation.
- Sonic Boom Reduction Techniques: Progress in aerospace engineering has yielded adaptive materials, Quiet Supersonic Technology (QueSST), and advanced aerodynamic designs, including the use of metamaterials to control and weaken sonic booms.
- Environmental Impact of Sonic Booms: Addresses the causes and effects on ecosystems and human communities, advocating for strategies to reduce shockwave impacts through improved aircraft designs, operational restrictions, and regulatory policies.
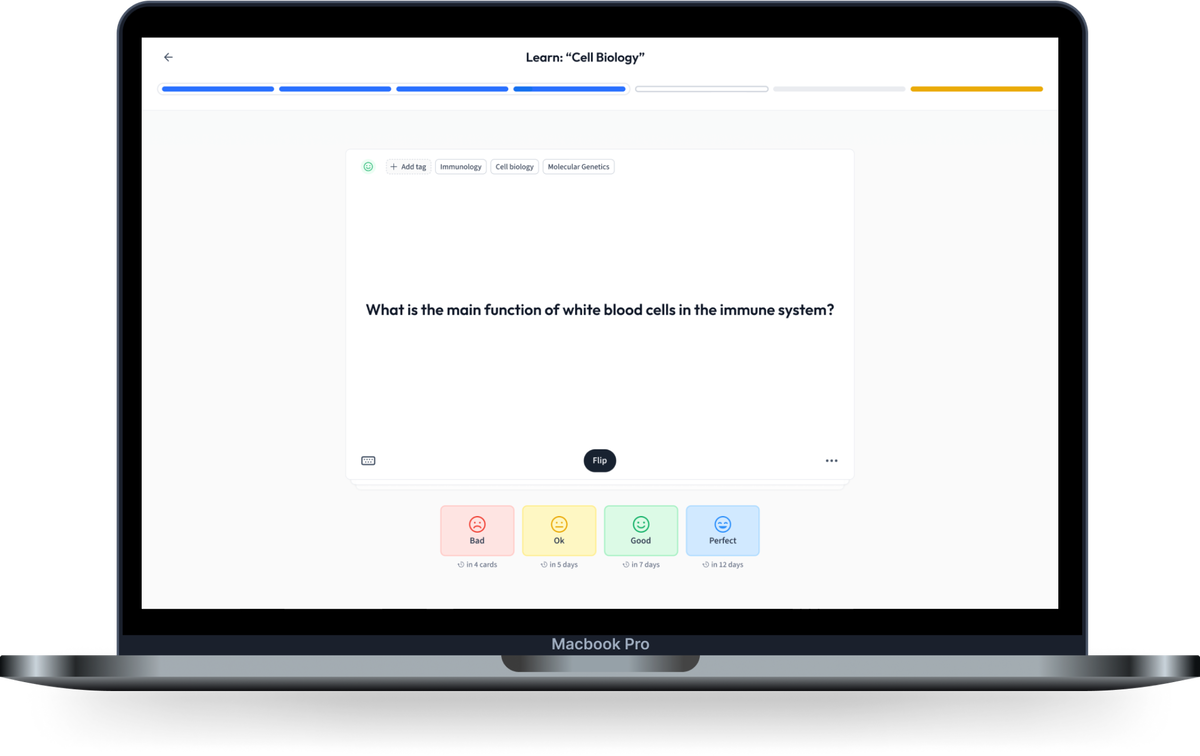
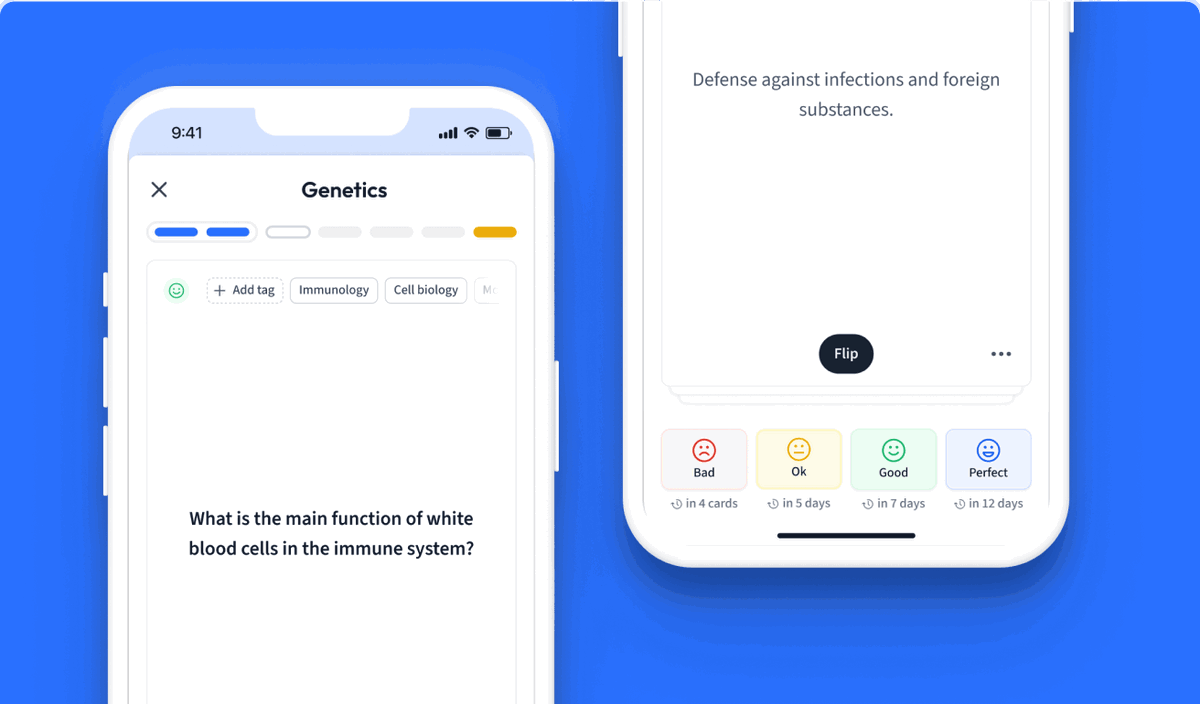
Learn with 12 Sonic Boom Analysis flashcards in the free StudySmarter app
Already have an account? Log in
Frequently Asked Questions about Sonic Boom Analysis
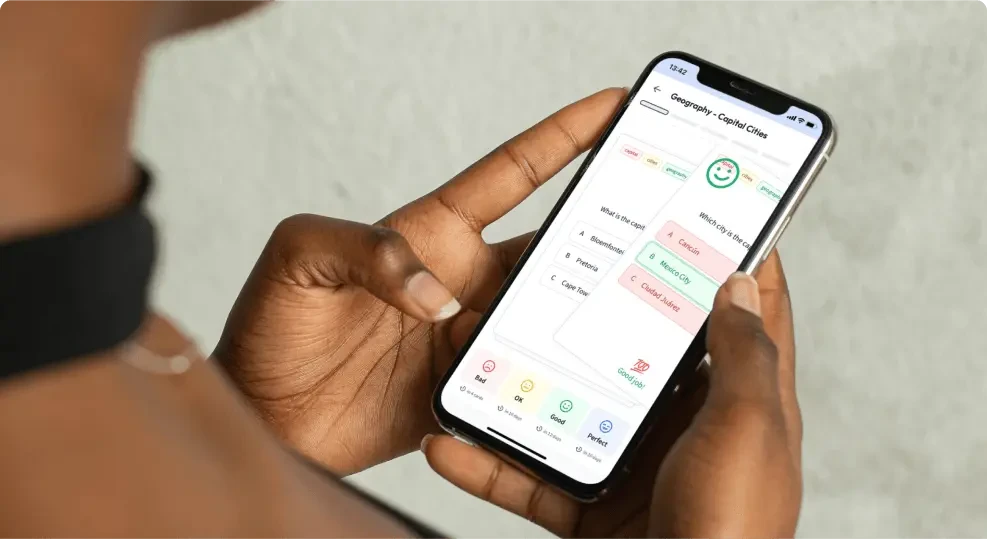
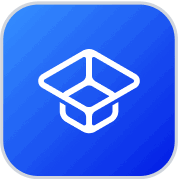
About StudySmarter
StudySmarter is a globally recognized educational technology company, offering a holistic learning platform designed for students of all ages and educational levels. Our platform provides learning support for a wide range of subjects, including STEM, Social Sciences, and Languages and also helps students to successfully master various tests and exams worldwide, such as GCSE, A Level, SAT, ACT, Abitur, and more. We offer an extensive library of learning materials, including interactive flashcards, comprehensive textbook solutions, and detailed explanations. The cutting-edge technology and tools we provide help students create their own learning materials. StudySmarter’s content is not only expert-verified but also regularly updated to ensure accuracy and relevance.
Learn more