Jump to a key chapter
Spacecraft Design: An Overview
Exploring the realm of Spacecraft Design unveils a world where technology, physics, and creativity converge to push the boundaries of what's possible beyond Earth's atmosphere. This field encompasses the theoretical and practical aspects of creating vehicles capable of travelling through space, tackling the unique challenges that come with operating in such an unforgiving environment.
Understanding the Basics of Spacecraft Design
At its core, Spacecraft Design involves a multidisciplinary approach, blending elements of aerospace engineering, materials science, propulsion systems, and more. Success in this domain requires not only an understanding of the scientific principles that govern space and movement but also creativity and innovative thinking to apply these principles in novel ways.The fundamental aspects of spacecraft design include:
- Structural integrity, ensuring the spacecraft can withstand the stresses of launch and space travel.
- Propulsion systems, which are crucial for manoeuvring in space and for initial launch from Earth.
- Communication systems, enabling data transmission to and from Earth.
- Life support systems, for missions involving human crews, ensuring survivability in the vacuum of space.
- Power systems, to provide the necessary energy for operations and instruments.
Did you know that the materials used in spacecraft must not only be lightweight and strong but also resistant to extreme temperatures and radiation?
The Role of Spacecraft in Modern Astronomy
Spacecraft have revolutionised the field of astronomy, allowing humanity to observe celestial bodies and phenomena from perspectives that are simply not possible from Earth's surface. These sophisticated machines have become essential tools in the exploration of our solar system and beyond, offering unique insights into the universe's countless mysteries.Key contributions of spacecraft to modern astronomy include:
- Direct exploration of planets, moons, and asteroids, providing invaluable data on their composition, atmospheres, and potential for life.
- Telescopes and observatories placed in orbit, free from Earth's atmospheric distortions, offer clearer and more detailed observations of distant galaxies, stars, and planetary systems.
- Solar system missions that have mapped other planets and moons in unprecedented detail, revolutionising our understanding of them.
The Hubble Space Telescope, sent into space in 1990, has been one of the most productive and vital tools in the field of modern astronomy, bringing the universe closer to home.
A Glimpse into Future Spacecraft Design
The future of Spacecraft Design holds incredible promise, with advancements in technology and materials science paving the way for even more ambitious missions. As we aim further into the cosmos, the next generation of spacecraft will likely incorporate breakthroughs in propulsion, artificial intelligence (AI), and sustainability to traverse vast distances and survive harsher conditions.Emerging trends expected to influence future spacecraft design include:
- Improved propulsion systems, such as electric or nuclear propulsion, offering faster travel times and higher efficiency.
- Autonomous systems powered by AI, enabling spacecraft to make real-time decisions without direct human input.
- Reusability and sustainability, with a focus on spacecraft that can be refurbished and relaunched, reducing waste and costs.
Innovations in material sciences are creating opportunities for lighter, stronger, and more adaptive materials to be used in spacecraft design, which could revolutionize the way we explore space.
Elements of Spacecraft Design
Delving into the elements of spacecraft design uncovers the intricate details and complex engineering behind vehicles destined for space. This area requires a harmonious blend of various scientific and technological disciplines, ensuring the spacecraft meets its mission specifications while withstanding the harsh conditions of space travel.
Key Components in Spacecraft Construction
Spacecraft are comprised of multiple key components, each serving a distinct purpose but working together to ensure mission success. These components include:
- Structural Framework: Provides the necessary strength and integrity to withstand the launch process and the space environment.
- Propulsion Systems: Allows the spacecraft to navigate and manoeuvre through space, crucial for reaching and maintaining orbit, as well as for interplanetary travel.
- Power Sources: Supplies energy to the spacecraft's systems and instruments, often through solar panels or nuclear power.
- Thermal Control: Maintains operational temperatures within safe limits to protect onboard systems and instruments.
- Communication Systems: Enables data transmission between the spacecraft and mission control on Earth.
- Payload: Comprises the scientific instruments or human crew, depending on the mission's objectives.
Structural Framework: The physical body of a spacecraft, designed to provide a sturdy base for attaching all other systems and payloads, while also ensuring the structural integrity is maintained under the stresses of launch and space travel.
An iconic example of successful spacecraft design is the Apollo Lunar Module, which was specifically engineered for lunar landings during the Apollo missions. Its unique structure and propulsion systems enabled astronauts to land on and take off from the Moon's surface.
The Interplay Between Design Elements in Spacecraft
In spacecraft design, the interplay between different components is critical to the vehicle's overall performance and safety. For instance, the propulsion system's requirements can influence the design of the structural framework, ensuring it can withstand the forces generated during manoeuvres. Similarly, the thermal control system must be designed with consideration for the spacecraft's orientation relative to the Sun and its operational environment in space. This cohesive integration of components ensures that the spacecraft can carry out its mission effectively.Examples of this integration include:
- Design considerations for thermal expansion and contraction in materials used in the structure and power systems.
- Strategic placement and protection of communication systems to maintain connectivity with Earth.
- Balance between propulsion capabilities and fuel storage, impacting the spacecraft's mass and mission duration.
Effective thermal management is crucial for spacecraft, as temperature variations in space can be extreme, potentially disrupting onboard systems and instruments.
How Elements of Spacecraft Design Influence Mission Outcomes
The effectiveness of a spacecraft's design is ultimately measured by its mission outcomes. For example, the choice of propulsion system affects the spacecraft's speed and how quickly it can reach its destination, impacting mission duration and data collection opportunities. Additionally, the robustness of the communication system determines the quality and quantity of data transmitted back to Earth, a crucial factor for scientific missions. Consequently, each design element, from structural integrity to power sourcing, plays a vital role in the mission's success or failure, highlighting the importance of a comprehensive and integrated approach to space vehicle design.Understanding the subtleties of each design element enables engineers to optimise spacecraft for specific missions, whether it’s exploring distant planets or observing Earth from orbit.
The Fundamentals of Spacecraft Mission Analysis and Design
Spacecraft mission analysis and design is a meticulous process that involves the careful consideration of various factors to ensure a mission's success. This process is fundamental for the strategic planning and execution of space missions, from satellites orbiting Earth to probes venturing into deep space.
Steps in Spacecraft Mission Design
The design and launch of a spacecraft follow a series of step-by-step procedures, each critical to the overall viability of the mission. These steps include:
- Defining mission objectives and goals.
- Conducting preliminary studies and feasibility analysis.
- Designing the spacecraft, focusing on its systems and components.
- Developing and testing prototypes.
- Executing detailed design and assembly.
- Performing pre-launch tests and final preparations.
- Launching the spacecraft.
- Conducting mission operations and data analysis.
Mission objectives: The desired outcomes or tasks the spacecraft is designed to accomplish during its mission, such as Earth observation, scientific research in space, or interplanetary exploration.
A notable example illustrating the crucial steps in spacecraft design is the Mars Rover, Curiosity. Its mission objectives focused on exploring Mars' Gale Crater as a potential environment for past life. The design, development, and deployment stages encapsulated years of planning, rigorous testing, and high-precision engineering to ensure the rover could withstand Mars' harsh conditions.
Case Studies: Successful Spacecraft Missions
The history of space exploration is filled with missions that have significantly advanced our understanding of the universe, demonstrating the potential of human ingenuity and technological prowess. Key case studies include:
- The Apollo 11 moon landing, which marked the first time humans set foot on the moon.
- The Voyager probes, which have provided invaluable data on the outer planets and are now traversing interstellar space.
- The Hubble Space Telescope, offering unprecedented views of space and contributing to major astronomical discoveries.
The Voyager Probes: Launched in 1977, Voyager 1 and Voyager 2 were designed for the primary mission of exploring Jupiter and Saturn. However, their mission was extended, and they went on to explore Uranus and Neptune, providing mankind with the first close-up images of these planets. Their journey continues, now as part of an interstellar mission, sending back data from beyond our solar system's outermost planets. Their longevity and continued contribution to science exemplify the success that can be achieved with proficient mission design and planning.
Challenges in Spacecraft Mission Analysis and Design
Designing a spacecraft mission involves navigating an array of challenges, both technical and operational. Key challenges include:
- Minimising the risk of mission failure.
- Adhering to budget constraints and project deadlines.
- Ensuring the spacecraft can withstand the harsh environments of space.
- Developing reliable communication systems for data transmission back to Earth.
- Choosing the right propulsion systems for the mission's specific needs.
One of the biggest challenges in spacecraft design is creating a thermal control system capable of maintaining operational temperatures across the extreme conditions of space.
Innovations in Spacecraft Propulsion Systems
The exploration of space has always hinged on the advancements in spacecraft propulsion systems. These systems are crucial for the launch, manoeuvrability, and overall success of missions beyond Earth's atmosphere. As technology progresses, so does the capability to explore further and more efficiently into space.
The Evolution of Spacecraft Propulsion
The history of spacecraft propulsion is a testament to human ingenuity, evolving from simple chemical rockets to complex systems harnessing atomic and even solar power. Each innovation has marked a leap forward in our space exploration capabilities.Starting with the V-2 rocket, the first object to reach the boundaries of space, propulsion technology has undergone significant transformations. Advancements have led to the Saturn V, which powered the Apollo missions, and further to the development of the Space Shuttle's reusable booster system. Today, ion thrusters and solar sails propose even less conventional means of space travel, highlighting the progression from explosive, chemical-based systems to more refined and efficient technologies.
Chemical propulsion, relying on the combustion of rocket fuel to generate thrust, was the first method used to send spacecraft beyond Earth's atmosphere.
Comparing Propulsion Systems: Traditional vs. Modern Approaches
The comparison between traditional and modern spacecraft propulsion systems reveals a dramatic shift in technology and efficiency. Traditional systems primarily utilised chemical reactions, which, while powerful, are limited by the need to carry large quantities of fuel, reducing payload capacity and efficiency.In contrast, modern propulsion technologies, such as electric and nuclear thermal propulsion, offer significant advantages:
- Efficiency: Modern systems provide a higher specific impulse, meaning they can produce more thrust per unit of fuel.
- Sustainability: Technologies like electric propulsion use less propellant, reducing mission costs and environmental impact.
- Duration: With modern propulsion, spacecraft can remain operational for longer durations, opening up pathways to deep space exploration.
Specific impulse (Isp) is a measure of the efficiency of rocket and jet engines, defined as the total thrust per rate of propellant consumption. It indicates how much thrust can be produced with a given amount of propellant, and is critical in determining a propulsion system's performance.
An example of modern propulsion technology is NASA's Dawn spacecraft, which utilised ion propulsion to explore the asteroid Vesta and the dwarf planet Ceres. Ion propulsion, which accelerates ions to generate thrust, represents a significant leap in efficiency compared to traditional chemical propulsion.
The Future of Spacecraft Propulsion: What's Next?
The future of spacecraft propulsion teems with possibilities, from theoretical concepts to technologies nearing practical application. Anticipated advancements include:
- Nuclear Thermal Propulsion (NTP): Offering vastly superior efficiency compared to chemical rockets, paving the way for manned missions to Mars.
- Electric Propulsion: With developments in solar electric propulsion being geared towards more sustainable and long-term space travel.
- Antimatter and Fusion Propulsion: While still in the realm of science fiction, research into these areas promises almost unimaginable speeds, potentially reducing travel time within our solar system from years to months or even weeks.
Nuclear Thermal Propulsion (NTP) is particularly exciting for its potential to revolutionise space travel. NTP systems use a nuclear reactor to heat a propellant like hydrogen, which then expands and is expelled to produce thrust. This method can theoretically provide a much higher specific impulse compared to chemical rockets, making it perfect for deep space missions where efficiency is paramount. Although challenges related to safety, cost, and technology remain, the prospect of NTP signifies a bold step towards faster and further space exploration.
Exploring Interplanetary Spacecraft Design
Interplanetary spacecraft design encompasses a complex blend of engineering, physics, and creativity to develop vehicles capable of traversing the vast distances between planets within our solar system. These missions, aimed at uncovering the mysteries of our planetary neighbours, require innovative solutions to address the unique challenges of interplanetary travel.
Design Considerations for Interplanetary Missions
Designing a spacecraft for an interplanetary mission involves careful consideration of several critical factors that influence both the design and performance of the spacecraft. Key considerations include:
- Propulsion and fuel efficiency to ensure the spacecraft can complete its journey and, if necessary, return to Earth.
- Navigation and control systems for accurate travel and manoeuvring in space.
- Communication systems capable of transmitting data across vast distances between planets.
- Life support systems for manned missions, ensuring the safety and well-being of the crew.
- Thermal protection to withstand extreme temperatures encountered in space.
Interplanetary spacecraft: A vehicle designed for space travel between planets within our solar system, capable of operating for extended periods in the harsh conditions of space.
The choice of propulsion system plays a pivotal role in determining the feasibility and duration of an interplanetary mission.
Iconic Interplanetary Spacecraft and Their Design Secrets
Several interplanetary spacecraft have made significant contributions to our understanding of space, each with unique design features that have enabled their success. Notable examples include:
- Voyager 1 and 2: Launched in 1977, these spacecraft utilised gravity assists to extend their mission far beyond the outer planets, into interstellar space.
- Cassini-Huygens: This mission to Saturn showcased advanced imaging and navigation technologies, along with a landing probe for Titan, Saturn's largest moon.
- Mars Rover Curiosity: Landing on Mars in 2012, Curiosity has explored the Martian surface with a suite of scientific instruments, powered by a radioisotope thermoelectric generator.
An image showcasing Mars Rovers on the Martian surface, highlighting advanced robotic technology and design innovation.
Cassini-Huygens's journey to Saturn involved the spacecraft entering the planet's orbit in 2004 and deploying the Huygens probe to Titan in 2005. This mission's design incorporated a shield to protect against Saturn's ring particles and precision instruments for detailed study of the planet's atmosphere and rings.
Overcoming Challenges in Interplanetary Spacecraft Design
Designing spacecraft for interplanetary missions poses a unique set of challenges that must be overcome to ensure mission success. Primary challenges include:
- Long-distance communication delays, requiring autonomous systems and operations.
- Extreme and variable thermal environments, demanding robust thermal management systems.
- High-energy radiation from the sun and cosmic rays, necessitating protective shielding for both electronics and human passengers.
- Limited ability to repair or service spacecraft once launched, emphasizing the need for reliability and redundancy in design.
Gravity assist, also known as a slingshot manoeuvre, plays a crucial role in interplanetary missions. By carefully plotting a spacecraft's trajectory so it passes near a planet, the spacecraft can gain significant speed using the planet's gravity, thereby conserving fuel. This technique has enabled missions like Voyager and Cassini to extend their operational range and explore multiple destinations within a single mission. Mastery of gravity assists symbolises the intricate planning and precision required in space mission analysis and design.
Akin's Laws of Spacecraft Design Explained
Delving into Akin's Laws of Spacecraft Design provides invaluable insights into the principles guiding spacecraft engineers and designers. Developed by Dr. David Akin, these laws encapsulate lessons learned from decades of experience in aerospace engineering and space exploration. They serve as a pragmatic guide, offering wisdom that transcends theoretical knowledge.
Understanding Akin's Laws in Spacecraft Design
Akin's Laws of Spacecraft Design are a series of insights and aphorisms that address the inherent complexities and challenges of designing vehicles for space. They highlight the importance of practical design decisions, the inevitability of trade-offs, and the critical nature of thorough testing.These laws cover a broad range of topics, including the importance of simplicity in design, the impact of system integration, the necessity of considering the operational environment, and the imperative of learning from past mistakes. Akin's Laws effectively synthesise hard-earned wisdom into concise statements that are readily applicable across various stages of spacecraft development.
An example of Akin's practical wisdom is that no design survives first contact with reality, underscoring the importance of testing and adaptation.
How Akin's Laws Guide Spacecraft Engineers
Akin's Laws serve as a cornerstone for spacecraft engineers by framing critical aspects of spacecraft design within the context of practical experience. These laws remind engineers to balance innovation with feasibility, emphasise extensive simulation and testing, and to always be prepared for the unexpected.For instance, Akin’s emphasis on redundancy (“Make it redundant”) and the significance of testing (“Test what you fly, fly what you test”) guide engineers in creating spacecraft that are both reliable and capable of fulfilling their mission objectives. By adhering to these principles, engineers can mitigate risks and enhance the overall mission success rate.
System Integration: A process in spacecraft design that entails bringing together various components and subsystems into a single, functioning whole, ensuring compatibility and performance across all systems.
A practical application of Akin’s Law that "Experience is directly proportional to the amount of equipment ruined" is the iterative development and testing of the Mars Rovers. With each mission, lessons learned from previous designs inform improvements, leading to more robust and capable rovers.
Applying Akin's Laws to Future Spacecraft Design Initiatives
As space exploration ventures further and aims higher, Akin's Laws will continue to be a guiding light for engineers and designers. These laws advocate for a design philosophy that prioritises adaptability, thorough testing, and learning from both success and failures.Future spacecraft design initiatives can benefit from these principles by incorporating advanced materials and technologies while maintaining a grounding in the practical realities of spacecraft engineering. For example, the push for more sustainable and reusable spacecraft calls for designs that balance innovation with proven engineering practices. Embracing Akin's Laws helps engineers navigate the complexities of developing spacecraft for new missions, ensuring designs are both forward-thinking and rooted in empirical wisdom.
One notable law, "Design is an iterative process," underscores the evolutionary nature of spacecraft development. This principle is particularly relevant in the context of rapidly advancing technology and changing mission requirements. By accepting that the first design attempt will likely not be perfect, engineers are encouraged to embrace a process of continual refinement. This iterative approach has been instrumental in the success of pioneering missions, such as the development of commercial crew spacecraft, which have undergone extensive revisions and testing to meet the stringent demands of human spaceflight.
Spacecraft Design - Key takeaways
- Spacecraft Design involves multidisciplinary elements, such as aerospace engineering, materials science, and propulsion systems, essential for successful space exploration.
- Key components of spacecraft include structural integrity, propulsion systems, communication systems, life support (for crewed missions), and power systems, each critical for mission success.
- Spacecraft Mission Analysis and Design is a process that includes defining objectives, designing the spacecraft, testing prototypes, detailed assembly, and conducting mission operations.
- Advancements in Spacecraft Propulsion Systems, like electric and nuclear propulsion, improve efficiency and mission duration, enabling more ambitious space exploration.
- Akin's Laws of Spacecraft design offer practical guidelines to engineers, emphasising simplicity, the need for redundancy, and the iterative nature of design.
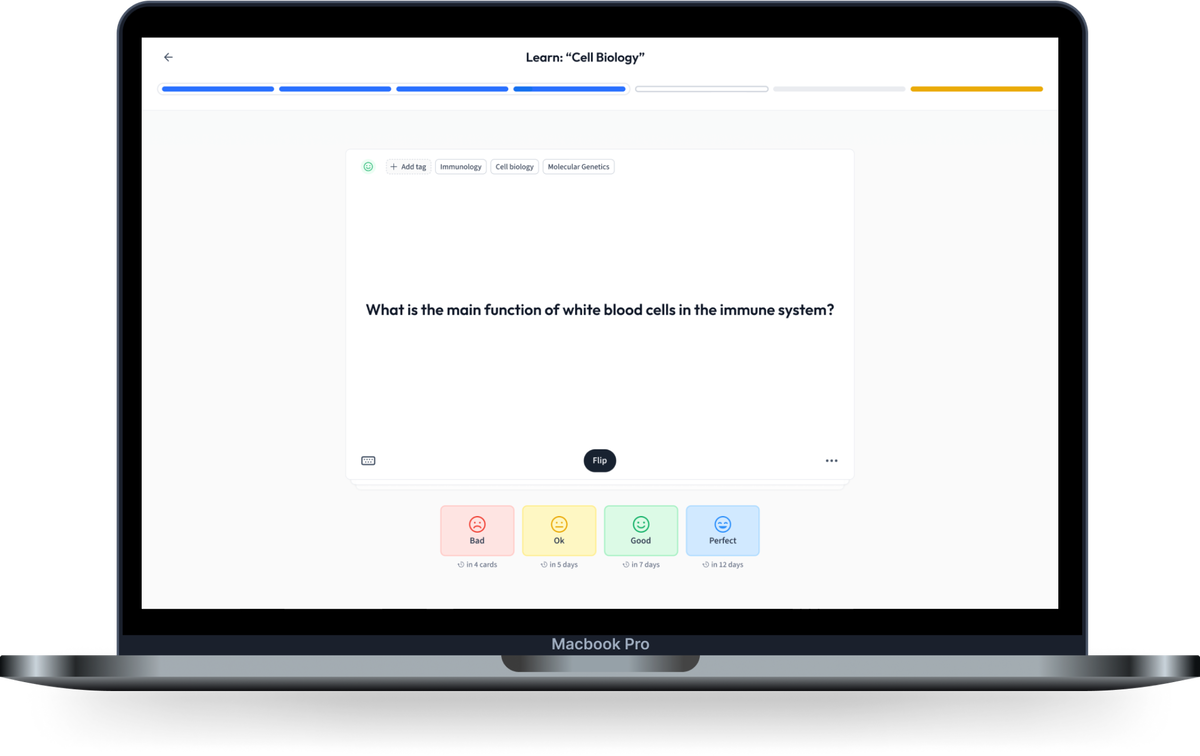
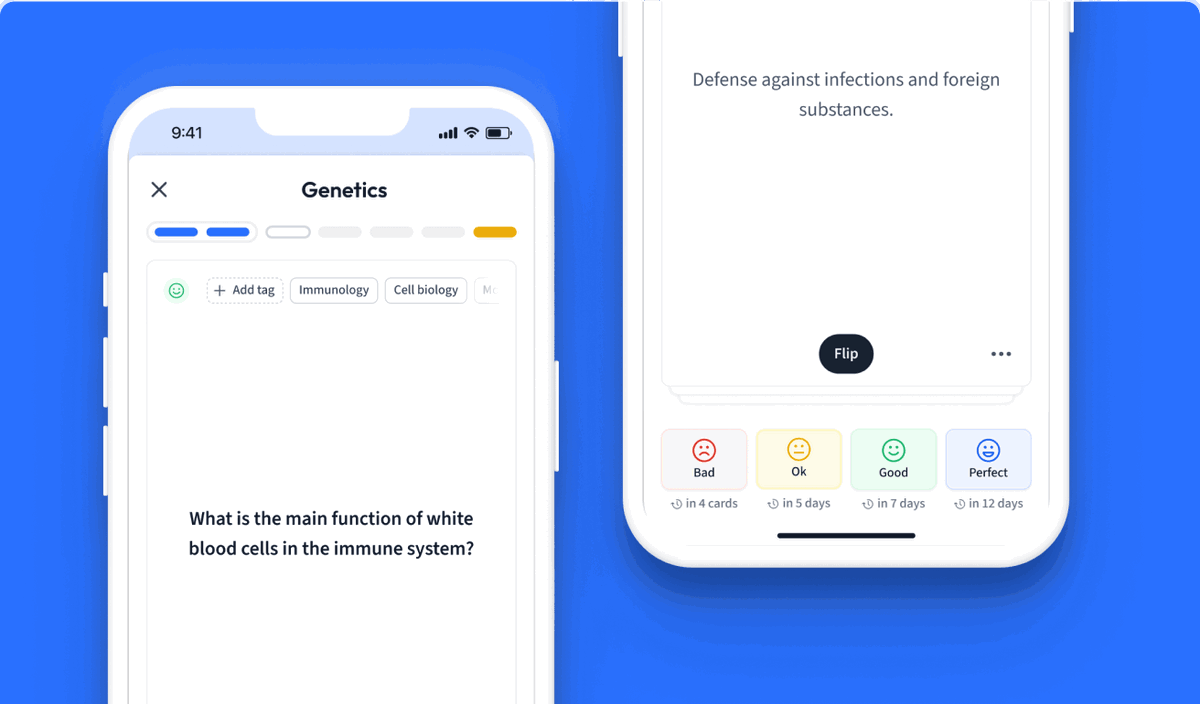
Learn with 12 Spacecraft Design flashcards in the free StudySmarter app
Already have an account? Log in
Frequently Asked Questions about Spacecraft Design
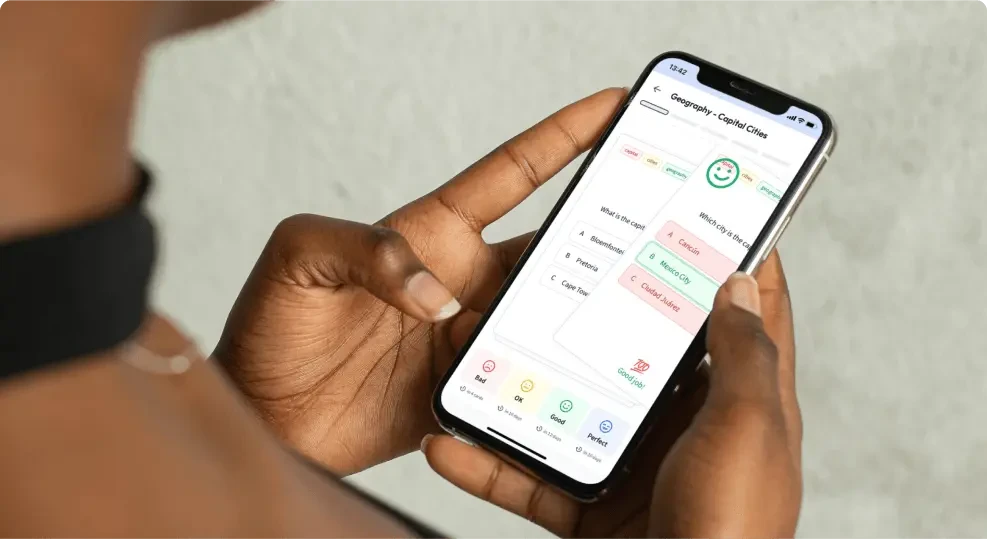
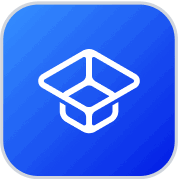
About StudySmarter
StudySmarter is a globally recognized educational technology company, offering a holistic learning platform designed for students of all ages and educational levels. Our platform provides learning support for a wide range of subjects, including STEM, Social Sciences, and Languages and also helps students to successfully master various tests and exams worldwide, such as GCSE, A Level, SAT, ACT, Abitur, and more. We offer an extensive library of learning materials, including interactive flashcards, comprehensive textbook solutions, and detailed explanations. The cutting-edge technology and tools we provide help students create their own learning materials. StudySmarter’s content is not only expert-verified but also regularly updated to ensure accuracy and relevance.
Learn more