Jump to a key chapter
What is Spacecraft Fuel?
Spacecraft fuel constitutes the propellants used to propel a spacecraft. These fuels are crucial for launching, maneuvering, and ensuring that spacecraft reach their intended destinations. Depending on their design and mission requirements, spacecraft might use different types of fuels, each with unique properties and applications.Understanding the types of fuels used and why certain fuels are chosen over others can provide insight into the complexities of space exploration. Whether for launching satellites, manned missions to space, or deep space exploration, the choice of fuel plays a pivotal role in the success of these missions.
Understanding Spacecraft Fuel Types
Spacecraft fuels can be broadly categorized into two major types: chemical propellants and electrical propulsion systems. Chemical propellants are further divided into solid and liquid fuels. Each type has its unique set of characteristics that determines its suitability for specific missions.
- Solid fuels are generally used for their simplicity and reliability. They are used in boosters for launching spacecraft.
- Liquid fuels offer more control and efficiency. They are used in various stages of launch and for in-space maneuvering.
- Electrical propulsion, such as ion drives, provides high efficiency for deep space missions despite low thrust.
Why Liquid Hydrogen is Used as a Fuel in Spacecraft
Liquid hydrogen (LH2) is widely regarded as an efficient spacecraft fuel, especially when paired with liquid oxygen (LOX) as an oxidizer. This combination is known for providing one of the highest efficiencies in terms of thrust per unit of fuel.The primary reasons for using liquid hydrogen in spacecraft include:
- High specific impulse, which means it can produce more thrust per unit mass of fuel than other options.
- Its ability to produce water as a byproduct, which can be crucial for life support in manned missions.
- Relatively abundant and environmentally friendly, with only water vapour as its combustion byproduct.
Specific impulse (Isp) is a measure of the efficiency of rocket and jet engines. It represents the amount of thrust generated per unit of propellant consumed, typically reported in seconds. High Isp values indicate a more efficient engine.
The Space Shuttle's main engines used a combination of liquid hydrogen and liquid oxygen, providing one of the highest specific impulse ratings amongst chemical rockets, greatly contributing to the shuttle's capabilities to reach orbit.
The Role of Hydrazine in Spacecraft
Hydrazine (N2H4) is a liquid fuel used extensively in spacecraft for in-space maneuvering and attitude control. Unlike propulsion systems that use fuels like liquid hydrogen for major thrusts needed during launch, hydrazine is used for precise, small adjustments and orientation control thanks to its ability to be stored as a liquid at room temperature and its rapid decomposition in the presence of a catalyst to produce gas.Why hydrazine is favoured for these applications:
- It does not require cryogenic storage conditions, unlike many other spacecraft fuels.
- Immediate and controlled reaction with a catalyst for efficient thrust control.
- Capability to be repeatedly started and stopped, making it ideal for missions requiring precise maneuverability.
Hydrazine has played a crucial role in numerous space missions, including satellite deployments and interplanetary spacecraft. Its utilization is a testament to the engineering challenges overcome in developing reliable, controllable propulsion systems for the unique environment of space. Despite its toxicity and the precautions required for safe handling, the benefits it brings to spacecraft manoeuvrability and control have made it an indispensable fuel in the arsenal of spacecraft designers.
Innovative Spacecraft Fuel Storage Techniques
Innovative Spacecraft Fuel Storage Techniques are essential to the success and efficiency of space missions. As spacecraft missions evolve, becoming longer and more complex, the need for effective storage solutions becomes increasingly critical. These techniques not only ensure the longevity and reliability of missions but also push the boundaries of what is possible in space exploration.Through advancements in technology and engineering, several innovative solutions have been developed to tackle the unique challenges presented by storing spacecraft fuel.
Challenges in Spacecraft Fuel Storage
Storing fuel in space presents a multitude of challenges that must be meticulously managed to ensure mission success. These challenges include:
- Extreme Temperature Variations: Spacecraft encounter incredibly cold temperatures in space, along with thermal radiation from the sun, requiring fuels to be stored in a way that ensures their stability and efficacy.
- Long Duration Missions: With missions to Mars and beyond on the horizon, fuel must be stored for longer periods, necessitating improved longevity without loss of performance.
- Microgravity: The absence of gravity affects fuel behaviour, including its delivery to engines, requiring specialised storage systems.
- Volume and Weight Constraints: Maximising payload capacity while minimising space and weight taken by fuel storage systems is a constant balancing act.
Revolutionary Techniques in Fuel Storage for Space
To address the significant challenges of fuel storage in space, several revolutionary techniques have been developed and implemented. These techniques are designed to improve efficiency, safety, and reliability of spacecraft missions.
- Cryogenic Fluid Management (CFM): Advances in insulation and refrigeration technologies enable long-term storage of cryogenic fuels, such as liquid hydrogen, by reducing evaporation and leakage rates.
- Microgravity-Compatible Fuel Tanks: Innovative tank designs accommodate the behaviour of liquids in microgravity, ensuring efficient fuel delivery to engines.
- Autonomous On-Orbit Refuelling: Technologies enabling spacecraft to be refuelled in orbit can extend missions, reduce launch weight, and allow for deeper space exploration.
- Solid Hydrogen Storage: Research into solid-state hydrogen storage methods promises a safer and more compact alternative to traditional liquid and gas storage options.
Cryogenic Fluid Management (CFM) refers to the technologies and methodologies used to control and utilise cryogenic substances, such as liquid hydrogen or oxygen, under the extreme conditions of space. The primary focus of CFM is to mitigate the challenges posed by storing these fluids for extended periods, particularly evaporation and boil-off.
NASA's Artemis missions, aimed at returning humans to the Moon, rely heavily on CFM technologies to ensure that the spacecraft's cryogenic fuels are effectively stored and managed. This enables the vehicle to perform lunar landings and returns, showcasing the critical role of advanced fuel storage techniques in modern space exploration.
Exploring the potential of nanomaterials in fuel storage represents a cutting-edge area of research. These materials could allow for the creation of lighter, more efficient fuel tanks that are able to store energy-dense fuels more effectively. Such advancements could significantly enhance the range and capabilities of future spacecraft, opening up new possibilities for exploration and travel within our solar system and beyond.
The choice of spacecraft fuel and its storage technique hinges not just on the destination but also on the mission's duration and specific requirements, such as payload size and weight limitations.
Hydrazine: A Special Fuel for Some Spacecraft
Hydrazine, a liquid chemical compound with the formula N2H4, stands out among spacecraft fuels for its unique properties and applications. It is utilised in various space missions, including satellite station-keeping, attitude control, and propulsion for interplanetary spacecraft. The distinct advantages of hydrazine make it a preferred choice for specific uses in space exploration.Diving into the reasons for its preference and the practices around its handling reveals the complexities and challenges of space mission fuel management.
Why Hydrazine is Chosen Over Other Spacecraft Fuel Types
Hydrazine offers several compelling advantages over other types of spacecraft fuel, leading to its selection for certain mission profiles. The primary benefits include:
- Highly reactive: Hydrazine easily decomposes in the presence of a catalyst to produce hot gases, making it highly efficient for providing thrust in space.
- Storable at room temperature: Unlike many rocket fuels that require cryogenic storage, hydrazine can be stored as a liquid at room temperature, simplifying logistics and storage systems on spacecraft.
- Immediate thrust: It offers the advantage of instant thrust when needed, crucial for timed manoeuvres and emergency adjustments.
Hydrazine (N2H4) is a clear, colourless liquid with an ammonia-like odour, used as a fuel in some spacecraft due to its efficient performance in the vacuum of space and its ability to produce significant thrust per unit mass.
Handling and Safety of Hydrazine in Space Missions
Despite its advantages, hydrazine is toxic and requires careful handling to ensure the safety of those working with it, as well as environmental protection. Safety measures adopted in space missions include:
- Specialized storage tanks: Designed to prevent leakage and to accommodate the expansion and contraction of hydrazine under different temperatures.
- Protective gear for handlers: Engineers and technicians are equipped with protective gear to avoid exposure during handling operations on the ground.
- Rigorous training: Mandatory training programmes for all personnel involved in fuel handling to mitigate risks associated with exposure.
- Automated fuel management systems: Advanced systems to minimize human interaction with hydrazine during flight operations.
The Mars Science Laboratory mission, which delivered the Curiosity rover to Mars, utilises hydrazine fuel for manoeuvring in space and for power generation on the rover itself. This showcases the critical role of hydrazine in enabling the precise navigation and operation of spacecraft in interplanetary missions.
The development of safer alternatives to hydrazine is an ongoing area of research in space propulsion. Compounds like green propellants, which are less toxic and offer similar or improved performance characteristics, are being explored. This shift not only aims at improving the safety of space missions but also at mitigating the environmental impact of space exploration on Earth and beyond. Adopting these innovations could dramatically change the landscape of spacecraft design and mission planning in the future.
The Future of Fuel in Spacecraft
As the boundaries of space exploration expand, so too does the innovation around spacecraft fuel. Advancements in fuel technology are crucial for longer, more complex missions. These innovations promise not only to power the journey but also to shape the future of how humans and machines travel through space.
Role of Fuel Cell Spacecraft in Space Exploration
Fuel cells represent a revolutionary approach to powering spacecraft. Unlike conventional combustion-based propulsion systems, fuel cells convert chemical energy directly into electricity through a reaction between a fuel and an oxidant. This process is efficient, reliable, and produces only water and heat as by-products, making it an eco-friendly option for long-duration space missions.The advantages of fuel cell technology in space exploration include:
- Higher efficiency compared to combustion engines
- Reduced weight and volume of fuel storage
- Capability to provide power for extended periods, ideal for deep space missions
Fuel cells can significantly extend the life of satellites and other spacecraft, reducing the need for frequent refuelling or replacement.
Emerging Trends in Spacecraft Fuel Technology
The landscape of spacecraft fuel technology is rapidly evolving, driven by the need for sustainability, efficiency, and deeper space exploration capabilities. Key emerging trends include:
- Green Propellants: These are less toxic alternatives to traditional chemical propellants, offering safer handling and reduced environmental impact.
- Advanced Electric Propulsion: Developments in electric and ion propulsion technology promise higher efficiency and longer mission durations.
- Nuclear Propulsion: Exploring the potential for nuclear-powered spacecraft offers the promise of vastly increased speed and range.
- In-Situ Resource Utilisation (ISRU): Techniques for using resources found on other planets for fuel could revolutionise interplanetary travel.
One of the most promising areas of research is the development of solar electric propulsion (SEP) systems. These systems use solar panels to generate electricity, which then powers an electric thruster. SEP systems could significantly decrease the amount of propellant required for missions, lower mission costs, and enable spacecraft to travel farther and longer than ever before. This technology is currently being evaluated for use in missions to Mars and beyond, highlighting its potential to be a game-changer in the realm of space exploration.
In-Situ Resource Utilisation (ISRU) refers to the practice of collecting and using materials found on other planets or moons to support space exploration missions. This can include extracting water to produce rocket fuel or using regolith (loose material covering solid rock) to construct habitats.
NASA's Artemis program, aiming to return humans to the Moon, plans to use ISRU to extract water from lunar ice. This water could then be split into hydrogen and oxygen, providing fuel and breathable air for astronauts, showcasing a practical application of emerging fuel technologies in space missions.
Spacecraft Fuel - Key takeaways
- Spacecraft Fuel: Propellants used in spacecraft, crucial for launching and maneuvering, with types including chemical propellants (solid and liquid fuels) and electrical propulsion systems.
- Liquid Hydrogen as Fuel: Offers high specific impulse and efficiency, produces water as byproduct, and is environmentally friendly as it emits only water vapour when combusted with liquid oxygen.
- Hydrazine (N2H4): A commonly used fuel in spacecraft for precision maneuvering, capable of being stored as a liquid at room temperature and offers controlled thrust upon rapid decomposition with a catalyst.
- Spacecraft Fuel Storage Techniques: Includes innovative solutions such as Cryogenic Fluid Management (CFM) and microgravity-compatible fuel tanks to address storage challenges posed by extreme temperatures, long duration missions, and microgravity conditions.
- Fuel Cell Spacecraft: Represent a sustainable and efficient approach to powering spacecraft, where fuel cells convert chemical energy into electricity, producing only water and heat as byproducts.
Learn faster with the 12 flashcards about Spacecraft Fuel
Sign up for free to gain access to all our flashcards.
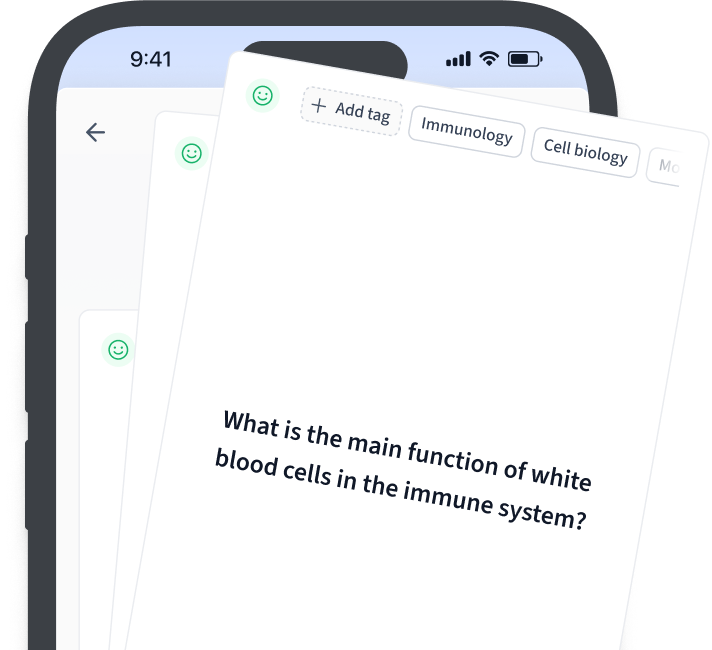
Frequently Asked Questions about Spacecraft Fuel
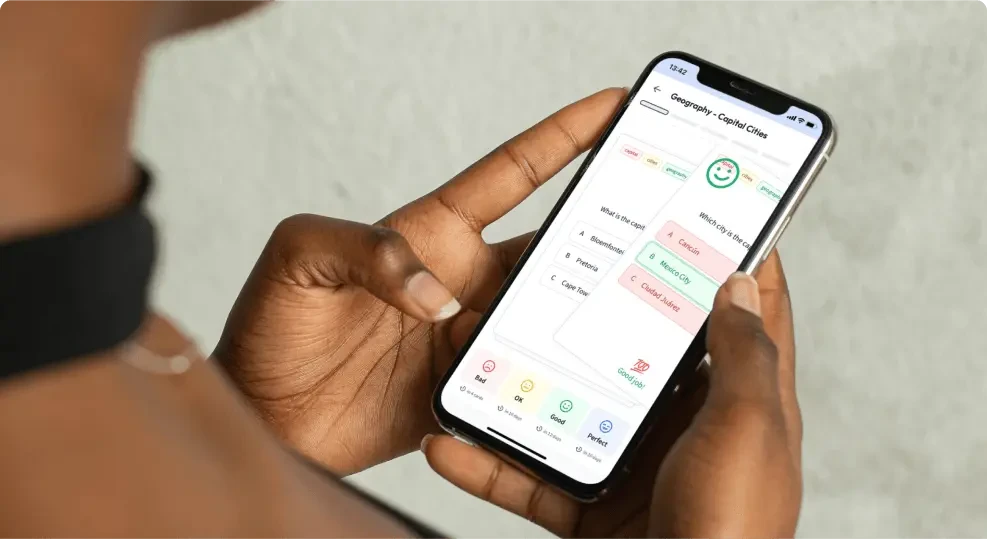
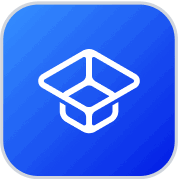
About StudySmarter
StudySmarter is a globally recognized educational technology company, offering a holistic learning platform designed for students of all ages and educational levels. Our platform provides learning support for a wide range of subjects, including STEM, Social Sciences, and Languages and also helps students to successfully master various tests and exams worldwide, such as GCSE, A Level, SAT, ACT, Abitur, and more. We offer an extensive library of learning materials, including interactive flashcards, comprehensive textbook solutions, and detailed explanations. The cutting-edge technology and tools we provide help students create their own learning materials. StudySmarter’s content is not only expert-verified but also regularly updated to ensure accuracy and relevance.
Learn more