Jump to a key chapter
Understanding Spacecraft Systems
Exploring space demands advanced technology and understanding the sophisticated systems that propel and support spacecraft is fascinating. In this journey through spacecraft systems, you will unlock the basics and delve into the components that make interstellar exploration possible.
The Basics of Spacecraft Systems
Spacecraft systems are at the heart of all space exploration missions. They encompass all the technologies required to support the spacecraft's journey, including launch, navigation, communication, and re-entry into Earth's atmosphere. Each system within a spacecraft has a unique function, designed to work in the harsh conditions of space.
A spacecraft is essentially a vehicle designed for space travel or operation in outer space. Spacecraft systems are fundamental to meeting mission objectives, whether for Earth observation, communication, navigation, or deep-space exploration. Understanding these systems is the first step towards comprehending how humans have managed to extend their reach beyond Earth.
Components of a Spacecraft System
The components of a spacecraft system can be divided into several categories, each critical for the spacecraft's operation and survival in space. These components are engineered to perform under extreme conditions, showcasing the pinnacle of human technological advancement.
Propulsion System: This system is responsible for moving the spacecraft and changing its trajectory in space. It includes engines that can work in the vacuum of space and the fuels that power them.
The fundamental components of a spacecraft system include, but are not limited to:
- Structural components, which provide the framework and stability for the spacecraft.
- Thermal control systems, which manage the temperature aboard the spacecraft, protecting it and its instruments from the extreme temperatures of space.
- Power systems, crucial for supplying energy to the spacecraft's instruments, often through solar panels or nuclear reactors.
- Avionics, which encompass the onboard computers, navigational aids, and data processing units that facilitate communication and control.
- Life support systems (in manned spacecraft), ensuring the crew's survival by managing air, water, and food supplies.
Each component is an engineering marvel, representing a field of study within aerospace engineering. These systems work in concert to ensure the spacecraft's functionality throughout its journey.
A remarkable example of spacecraft system engineering is the propulsion system used in rocket launches. Rockets use a combination of high-powered engines and fuel to escape Earth's gravitational pull. This involves a careful balancing of thrust, weight, fuel efficiency, and trajectory calculation. Technologies such as reusable rocket boosters have revolutionized space travel, drastically reducing the cost and increasing the feasibility of space exploration.
Remember, while astronauts get the spotlight, it's the spacecraft systems that ensure their safe journey and return.
Propulsion Systems for Spacecraft
Propulsion systems are crucial for spacecraft, enabling them to launch from Earth, manoeuvre in space, and sometimes return. These systems vary greatly, from traditional chemical rockets to advanced electric propulsion technologies.
Traditional vs. Ion Propulsion Systems for Spacecraft
Traditional propulsion systems rely on chemical reactions to produce thrust. These systems burn fuel, which generates gases expelled at high speeds to propel the spacecraft. In contrast, ion propulsion, a type of electric propulsion, uses electrical energy to accelerate ions out of the engine. This creates thrust in accordance with Newton's third law of motion.
Ion Propulsion: A propulsion technology that uses beams of ions (charged particles) as the reaction mass, creating thrust in space.
One real-world example of ion propulsion is NASA's Dawn spacecraft. It used an ion engine to travel to and study the asteroid belt, showcasing the long-duration operation ability of such systems.
Ion propulsion systems, despite their lower thrust compared to traditional systems, excel in efficiency. They can operate for years on a fraction of the fuel, making them ideal for long-duration, deep-space missions. This is because ion engines produce thrust through the acceleration of ions obtained by ionizing a propellant, typically xenon, using electric power.
Ion propulsion systems are far more efficient than traditional chemical rockets, allowing spacecraft to carry less propellant for the same mission parameters.
Electric Propulsion System Spacecraft: A Deep Dive
Electric propulsion systems for spacecraft represent a broad category that includes ion propulsion. These systems use electrical energy (often from solar panels) to generate thrust, vastly improving efficiency over chemical systems.
Another form of electric propulsion is Hall effect thrusters, which operate by accelerating ions through a magnetic field. Electric propulsion technologies demonstrate the potential for reducing payload weight, increasing mission lifespan, and expanding the capabilities of spacecraft.
The efficiency of electric propulsion systems is often measured in terms of specific impulse, which is significantly higher in electric systems compared to chemical propulsion. This efficiency makes electric propulsion particularly suited for missions requiring sustained thrust over long periods, such as station keeping, orbit transfers, and deep-space exploration. The reduced need for propellant can substantially decrease launch costs and allow for more scientific instruments or other payloads to be included in missions.
Specific impulse is a measure of how effectively a rocket uses its propellant or mass, with electric propulsion offering values several times that of chemical rockets.
Future Spacecraft Propulsion Systems and Integration
The future of spacecraft propulsion systems looks towards even greater efficiency and the integration of new technologies. Developments like nuclear thermal propulsion (NTP) and solar sails are being investigated for their potential to further reduce reliance on traditional chemical propellants.
NTP, for instance, could offer a balance between the high thrust of chemical systems and the high efficiency of electric propulsion. Meanwhile, solar sails rely on the momentum of photons from the sun to propel spacecraft, eliminating the need for propellant altogether. The integration of these systems, alongside advancements in materials and energy storage, will define the next generation of space exploration.
As propulsion technology evolves, so too does the potential for human and robotic exploration of space. Integration techniques, including modular spacecraft designs and adaptable propulsion systems, will be key in navigating the complex environment of outer space. These technological advancements will expand humanity's reach in the solar system, opening up new possibilities for discovery and exploration.
The integration of new propulsion technologies could revolutionize travel within our solar system, dramatically reducing transit times and opening new frontiers for exploration.
Spacecraft Attitude Control System
The Spacecraft Attitude Control System plays a crucial role in ensuring that a spacecraft maintains the correct orientation during its journey through space. This system allows a spacecraft to know and control its position relative to Earth, the sun, or any other celestial bodies it might be studying or using for navigation.
How Spacecraft Attitude Control Works
At the heart of spacecraft attitude control is the principle of maintaining a spacecraft's orientation or 'attitude' in space. This is achieved through a combination of sensors and actuators that work together to detect and adjust the spacecraft's position. Sensors, such as star trackers and sun sensors, provide data on the spacecraft's current orientation. Actuators, including reaction wheels and thrusters, then make the necessary adjustments to align the spacecraft as required.
The process involves:
- Monitoring the spacecraft's current orientation with regard to a defined frame of reference.
- Determining the desired orientation for mission objectives.
- Executing manoeuvres to correct any deviation from the desired orientation.
Attitude Control System (ACS): A system within a spacecraft designed to control the orientation of the vehicle, based on the desired position relative to the Earth, other celestial objects, or an inertial space frame.
Attitude control is particularly critical for missions that require precise positioning to collect data, such as Earth observation satellites, interplanetary vehicles, and telescopes. The accuracy of this system affects not only the mission's success but also the quality of the data collected. Advanced algorithms and control mechanisms are employed to ensure the spacecraft can autonomously maintain its attitude, even in the unpredictable environment of space.
The Role of Gyroscopes in Spacecraft Attitude Control
Gyroscopes play a pivotal role in spacecraft attitude control systems by continuously measuring the rate of rotation around the spacecraft's three axes. This information is vital for determining the spacecraft's orientation in space.
A typical application involves:
- Using gyroscopic data to identify unintentional drifts or rotations.
- Feeding this information into the spacecraft's control system.
- Activating thrusters or reaction wheels to counteract these movements and stabilise the spacecraft's attitude.
A notable application of gyroscopes was on the Hubble Space Telescope. Its high-precision gyroscopes allowed it to remain focused on celestial targets for extended periods, ensuring the acquisition of high-quality observations and images. The failure of these gyroscopes can lead to significant challenges in maintaining attitude control, underscoring their importance in space missions.
Challenges in Spacecraft Attitude Control
Spacecraft attitude control is fraught with challenges, stemming from both the inherent unpredictability of the space environment and the limitations of current technology. Some of the key challenges include:
- External Disturbances: Solar radiation pressure, gravitational forces from celestial bodies, and magnetic fields can alter a spacecraft's orientation unexpectedly.
- Hardware Limitations: The finite life of actuators and sensors, along with potential failures, poses a risk to sustained attitude control.
- Fuel Consumption: For spacecraft relying on thrusters for attitude adjustment, efficient use of fuel is a constant concern, as running out of fuel can end a mission prematurely.
One of the greatest tests of spacecraft attitude control was encountered by the Kepler Space Telescope. Kepler's mission was extended using an innovative technique called 'the Kepler Hack,' which involved using the pressure of sunlight as a virtual thruster to help maintain its pointing ability, despite having lost two of its reaction wheels. This solution exemplifies the ingenuity required to overcome the challenges associated with spacecraft attitude control.
Innovations such as the deployment of CubeSats for scientific missions showcase the evolving sophistication of attitude control systems in minuscule platforms, where traditional systems are impracticable.
The Science of Spacecrafts and Launch Systems
The journey of a spacecraft from concept to orbit encompasses a wide array of scientific disciplines and engineering feats. This exploration delves into the intricacies of spacecraft design and the innovative launch systems that propel them into space.
From Theory to Launch: The Science Behind Spacecrafts
The process of creating a spacecraft begins long before any physical construction takes place. It starts with theoretical groundwork in physics, mathematics, and material science. Understanding the demanding environment of space is paramount, including temperature extremes, vacuum pressure, and cosmic radiation.
Designers must also consider the spacecraft's mission objectives. Whether it's to observe Earth, explore distant planets, or carry humans into space, each mission requires custom solutions to propulsion, navigation, and survival in the harsh conditions of outer space.
Key Technologies in Modern Launch Systems
Modern launch systems leverage cutting-edge technologies to boost efficiency, reliability, and safety. Innovations such as reusable rocket components and advanced materials have revolutionised this field.
Reusable rockets, for example, can land back on Earth after launching their payloads, dramatically reducing the cost of access to space. Similarly, the integration of stronger, lighter materials improves the payload capacity and reduces fuel consumption.
Reusable Rockets: Launch vehicles designed to be recovered and re-launched multiple times, a key advance in reducing the cost of space exploration.
SpaceX's Falcon 9 is a prime example of a reusable rocket, capable of launching payloads to orbit and then landing vertically either on a drone ship at sea or back at the launch site.
The advent of ion propulsion systems represents a leap in spacecraft efficiency. Unlike traditional chemical rockets that burn fuel for a short, powerful thrust, ion thrusters emit ions to generate continuous, albeit weaker, thrust over long periods. This enables spacecraft to achieve greater speeds with significantly less propellant, opening new horizons for deep space exploration.
Advanced materials, including composites and superalloys, are essential for withstanding the extreme conditions of launch and the space environment, thus enabling the creation of more resilient spacecraft.
Evolution of Spacecraft Launch Systems
The evolution of spacecraft launch systems is a testament to human ingenuity and the relentless pursuit of exploration. Historically, these systems have progressed from rudimentary, single-use rockets to sophisticated launch vehicles equipped with stages, allowing them to reach higher altitudes with greater payloads.
In recent years, the focus has shifted towards sustainability and efficiency. The development of partially and fully reusable rockets represents a significant leap forward, promising a future where access to space becomes more routine and affordable.
The shift from government-led space missions to commercial space activities has been pivotal in driving innovation in launch systems. Commercial entities are not only refining existing technologies for greater reliability and cost-effectiveness but are also exploring novel concepts like air-breathing rocket engines, which could revolutionise how we access space by using atmospheric oxygen to burn fuel, thus carrying less onboard.
Spacecraft Systems - Key takeaways
- Spacecraft Systems: Encompass all technologies required for a spacecraft's journey, including launch, navigation, and communication.
- Propulsion Systems: Key for spacecraft to launch, manoeuvre, and return to Earth; includes traditional chemical rockets and advanced electric propulsion systems such as ion propulsion.
- Ion Propulsion: A technology that accelerates ions to create thrust, characterized by high efficiency and suitability for long-duration deep-space missions.
- Spacecraft Attitude Control System: Ensures correct spacecraft orientation using a combination of sensors and actuators; critical for missions that require precise positioning.
- The Science of Spacecrafts and Launch Systems: Covers the journey from spacecraft concept to orbit and includes advancements like reusable rockets and the development of new materials.
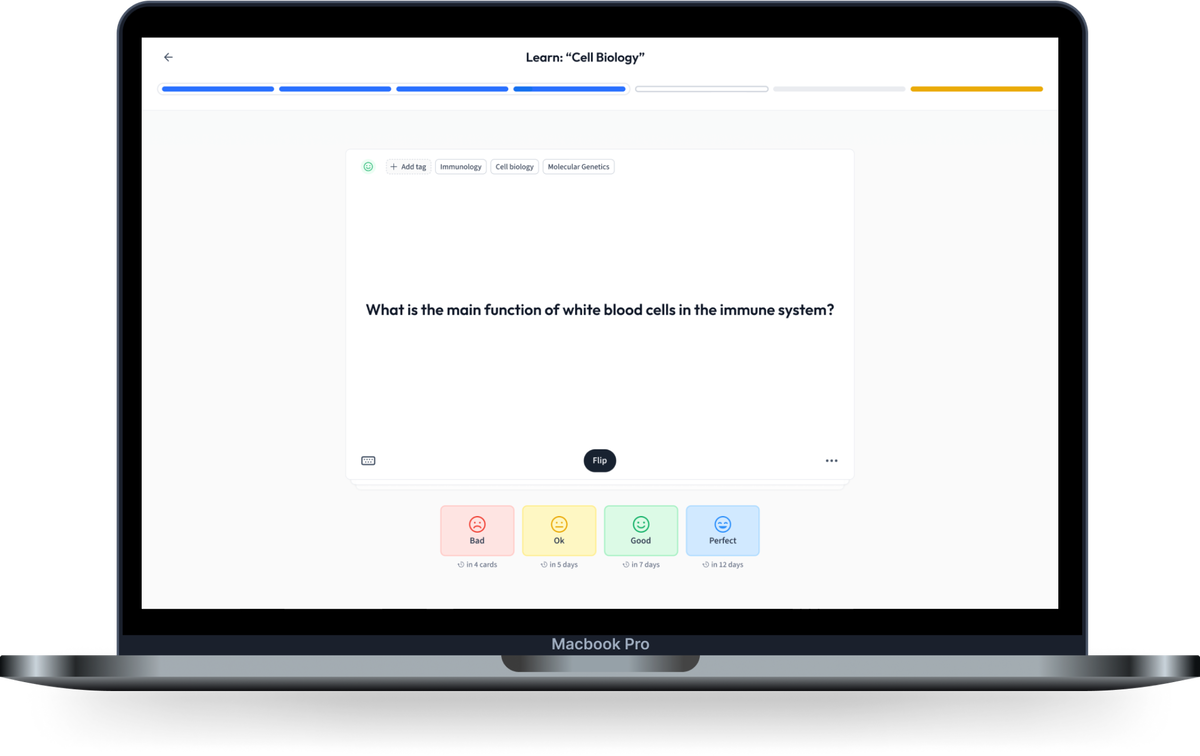
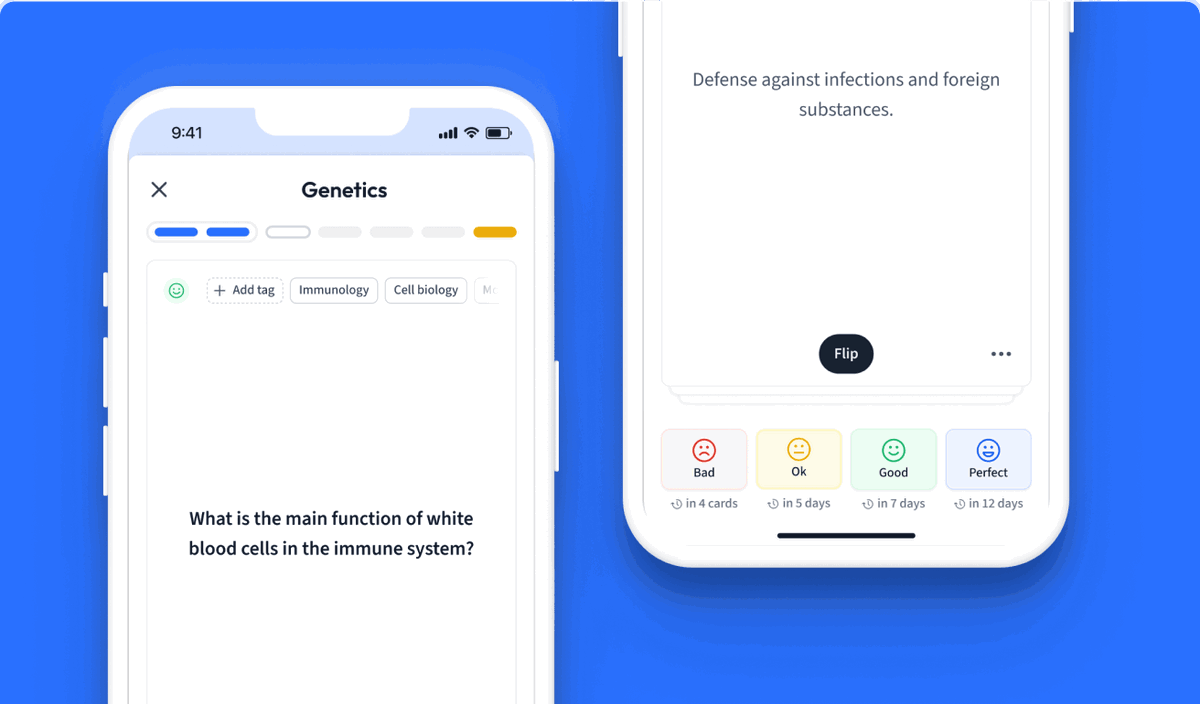
Learn with 12 Spacecraft Systems flashcards in the free StudySmarter app
Already have an account? Log in
Frequently Asked Questions about Spacecraft Systems
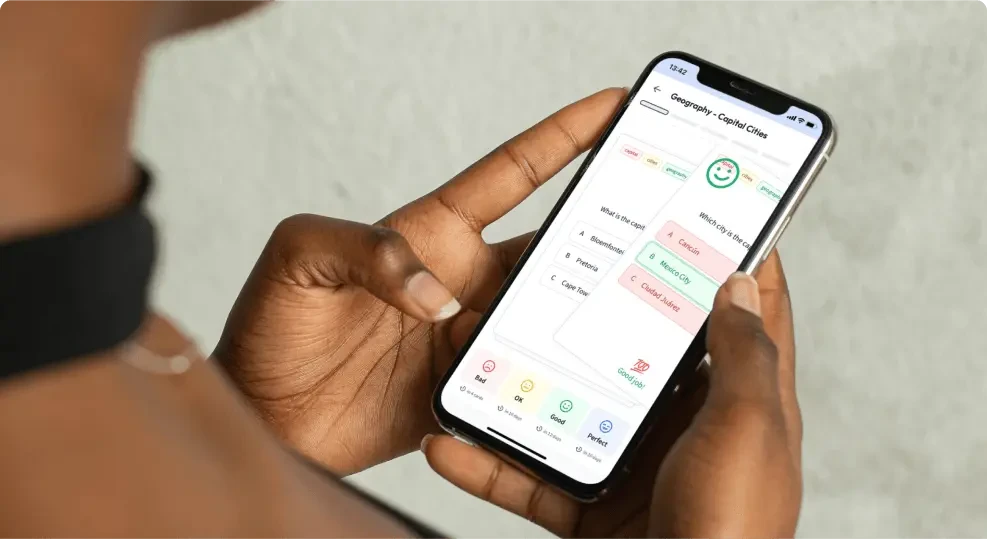
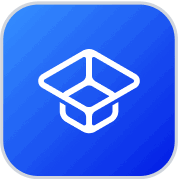
About StudySmarter
StudySmarter is a globally recognized educational technology company, offering a holistic learning platform designed for students of all ages and educational levels. Our platform provides learning support for a wide range of subjects, including STEM, Social Sciences, and Languages and also helps students to successfully master various tests and exams worldwide, such as GCSE, A Level, SAT, ACT, Abitur, and more. We offer an extensive library of learning materials, including interactive flashcards, comprehensive textbook solutions, and detailed explanations. The cutting-edge technology and tools we provide help students create their own learning materials. StudySmarter’s content is not only expert-verified but also regularly updated to ensure accuracy and relevance.
Learn more