Jump to a key chapter
What is Thermal Cycling?
Thermal cycling refers to the process through which materials are exposed to alternate cycles of heating and cooling. This testing method is crucial in understanding how materials behave under the fluctuating temperatures they might experience in real-world conditions. Whether for industrial components, electronic devices, or aerospace engineering, thermal cycling helps predict the longevity and reliability of materials and components.
Thermal Cycling Definition
Thermal Cycling: A repeated process of heating and cooling materials to evaluate their thermal endurance, stability, and to simulate real-world temperature fluctuations they will undergo during their operational life.
In the realm of engineering and materials science, this process is indispensable. Understanding how materials expand, contract, or potentially degrade under these conditions is crucial for designing reliable products.
While most materials are subjected to this process to ensure durability, certain materials, like polymers and metals, exhibit very distinct behaviours under thermal stress, making the analysis even more critical.
Importance of Thermal Cycling in Aerospace Engineering
In aerospace engineering, thermal cycling testing is paramount due to the extreme temperature ranges encountered in flight operations, from the intense cold of outer space to the friction-induced heat of re-entry into the Earth's atmosphere. Thus, materials and components used in spacecraft and aircraft must withstand these conditions without failing.
Consider the case of spacecraft materials that are subjected to temperatures ranging from below -150°C in space to over 1,200°C during re-entry into Earth's atmosphere. The ability of a material to withstand such drastic changes without compromising its integrity is what makes thermal cycling tests a cornerstone in the aerospace industry.
Example: The thermal protection system of the Space Shuttle was designed to withstand the thermal cycling experienced during its missions. Materials such as reinforced carbon-carbon on the wing leading edges and silica fibre tiles across the fuselage were subjected to rigorous thermal cycling tests to ensure their performance and safety.
Moreover, the reliability of electronic systems used in aerospace is tested via thermal cycling to ensure that components like circuit boards, sensors, and chips can endure the swift temperature transitions. The adherence to stringent thermal cycling tests ensures the safety, functionality, and success of aerospace missions.
Understanding the Thermal Cycling Process
The thermal cycling process is a critical aspect of material testing and product development. It helps researchers and engineers understand how materials and components behave under the stress of repeated thermal variation. This information is pivotal in determining the durability and lifetime of materials in various applications, from consumer electronics to aerospace engineering.
Steps in the Thermal Cycling Process Explained
Thermal cycling involves several key steps to accurately assess material performance under thermal stress. It starts with the selection of temperature ranges that the material will be exposed to, based on its expected operating conditions. The process proceeds through several stages, each critical to obtaining reliable data. Here are the primary steps involved:
- Initial temperature assessment to establish a baseline.
- Ramp up to the maximum temperature at a controlled rate.
- Hold at the maximum temperature for a specified period.
- Cool down to the minimum temperature at a controlled rate.
- Hold at the minimum temperature for a certain duration.
- Repeat the cycle for the desired number of times.
Temperature ramp rates and hold times are crucial variables in thermal cycling tests, directly influencing the stress levels materials experience.
Role of Thermal Cycling in Material Testing
Thermal cycling plays a pivotal role in material testing, offering insights into a material's thermal fatigue resistance, reliability, and lifecycle under fluctuating temperatures. By simulating the conditions a material faces in its actual application environment, engineers can predict its performance and identify potential failure points before they occur. The outcomes of thermal cycling tests are indispensable for several reasons:
- Identifying Material Defects: Thermal cycling can expose existing weaknesses in materials, such as cracks, delamination, or debonding, that aren't visible under constant temperatures.
- Evaluating Thermal Expansion Coefficient: The test helps measure how much a material expands and contracts with temperature changes, which is vital for designs requiring precise dimensional tolerances.
- Assessing Durability and Reliability: The ability of a material to withstand repeated thermal cycling without degradation is a good indicator of its durability and reliability in real-world applications.
- Safety and Compliance: For many products, particularly in sectors such as aerospace, automotive, and electronics, passing thermal cycling tests is a regulatory requirement, ensuring the safety and reliability of the final product.
Exploring Thermal Cycling Tests
Thermal cycling tests are essential to evaluate how different materials and components perform under repetitive heating and cooling. This kind of testing simulates the conditions that products may face during their lifecycle, providing vital information on their durability and reliability.Understanding the process involved in conducting a thermal cycling test, as well as the key components of a thermal cycling chamber, is crucial for engineers and researchers. These insights help in designing products that are more resilient and have a longer lifespan.
How a Thermal Cycling Test is Conducted
A thermal cycling test involves subjecting a material or component to several rounds of heating and cooling to assess its thermal fatigue resistance. The process is meticulously planned to replicate the actual conditions the material will face. Here's a typical sequence in the thermal cycling process:
- Determining the temperature extremes that the product will encounter.
- Setting up the test to cycle between these extremes.
- Controlling the rate of temperature change and the dwell time at each extreme.
- Repeating the cycles for the number of times required to gain significant data.
The number of cycles in a thermal cycling test can vary significantly, from just a few cycles for preliminary testing to thousands for comprehensive analysis.
Components of a Thermal Cycling Chamber
Understanding the components that make up a thermal cycling chamber is key to appreciating how these sophisticated devices can simulate various environmental conditions. A thermal cycling chamber is made up of several critical parts:
Component | Function |
Temperature Controller | Manages the temperature settings, including ramp rates and dwell times, to accurately simulate conditions. |
Heating and Cooling System | Generates the required temperatures, often using electrical heaters for heating and refrigerants or liquid nitrogen for cooling. |
Insulation | Maintains the temperature integrity of the chamber, minimizing energy loss and ensuring uniform temperature distribution. |
Air Circulation System | Ensures consistent temperature throughout the chamber by evenly distributing heat and cold. |
Data Logging and Monitoring System | Records temperature data, cycle times, and other relevant test conditions for analysis. |
Thermal Efficiency in Engineering Cycles
Thermal efficiency plays a pivotal role in engineering cycles, providing insights into how well these systems convert heat into work or other forms of useful energy. In the world of thermodynamics, thermal efficiency is a measure of performance that engineers strive to maximise in order to reduce waste, enhance sustainability, and optimise the operation of machines and power plants.
Carnot Cycle Thermal Efficiency
Carnot Cycle Thermal Efficiency: The theoretical maximum efficiency that a heat engine can achieve in converting heat into work, operating between two temperatures.
The Carnot cycle is a principle in thermodynamics that offers a benchmark for the efficiency of heat engines. With no losses in heat to friction or other processes, it represents an idealised process. The efficiency of a Carnot cycle is determined by the temperatures of the heat source and the heat sink. The formula for calculating the thermal efficiency ( heta) of a Carnot engine is given by: heta = 1 - (T_c/T_h)where T_c is the absolute temperature of the cool reservoir, and T_h is the absolute temperature of the hot reservoir, both measured in Kelvin (K).
Example: Imagine a Carnot engine working between a heat source at 500 K and a heat sink at 300 K. The thermal efficiency would be calculated as follows:1 - (300/500) = 0.4This means 40% of the heat energy is converted into work, while the remaining 60% is rejected to the cool reservoir. It serves as an ideal, demonstrating the limitations imposed by thermodynamics on real-world engines.
No real engine can achieve the Carnot efficiency due to inevitable losses, but it serves as a gold standard for comparing the performance of thermal machines.
Thermal Efficiency of Rankine Cycle
Rankine Cycle Thermal Efficiency: A measure of performance for steam power plants and heat engines that operate on a liquid-vapour cycle, primarily used in electricity generation.
The Rankine cycle is a fundamental model for steam-based thermal power systems, which includes most fossil fuel and nuclear power plants. The cycle involves four main processes: pumping the working fluid to high pressure, heating and vaporising the fluid, expanding the vapour through a turbine to generate power, and finally condensing the vapour and repeating the cycle.The efficiency of a Rankine cycle is affected by various factors, including the temperatures and pressures at which the system operates. Improving thermal efficiency in Rankine cycles often involves increasing the temperature and pressure of the steam, but this is limited by material strength and safety considerations.
To enhance the efficiency of Rankine cycles, modern power plants employ techniques such as reheat and regeneration. Reheat involves taking exhaust steam from the turbine and reheating it before sending it back into the turbine. Regeneration utilises steam extracted from the turbine to preheat the boiler feedwater. These methods contribute to efficiency gains by reducing the thermal energy rejected to the environment and making better use of the input heat energy.
Example: A Rankine cycle with a steam turbine operates between a high temperature of 823 K (550°C) and a low temperature of 293 K (20°C). While it might not reach the efficiency of a theoretical Carnot engine, modern enhancements like superheating, reheating, and regeneration can push real-world efficiencies closer to this theoretical limit.
The efficiency of real-world Rankine cycles typically ranges between 30% to 40%, significantly influenced by operational parameters and the technology applied to reduce energy losses.
Thermal Cycling - Key takeaways
- Thermal Cycling Definition: A process of heating and cooling materials repeatedly to test their thermal endurance and behaviour under real-world temperature fluctuations.
- Thermal Cycling Test: A method used to evaluate how materials and components perform under repetitive thermal stress, informing durability, reliability, and regulatory compliance.
- Thermal Cycling Chamber Components: Includes a temperature controller, heating and cooling system, insulation, air circulation system, and data logging and monitoring for precise environment simulation.
- Carnot Cycle Thermal Efficiency: Represents the maximum theoretical efficiency of a heat engine, calculated using the temperatures of the heat source and the heat sink in Kelvin.
- Thermal Efficiency of Rankine Cycle: A measure of performance in steam power plants, with efficiency improvements through techniques such as reheat and regeneration.
Learn faster with the 12 flashcards about Thermal Cycling
Sign up for free to gain access to all our flashcards.
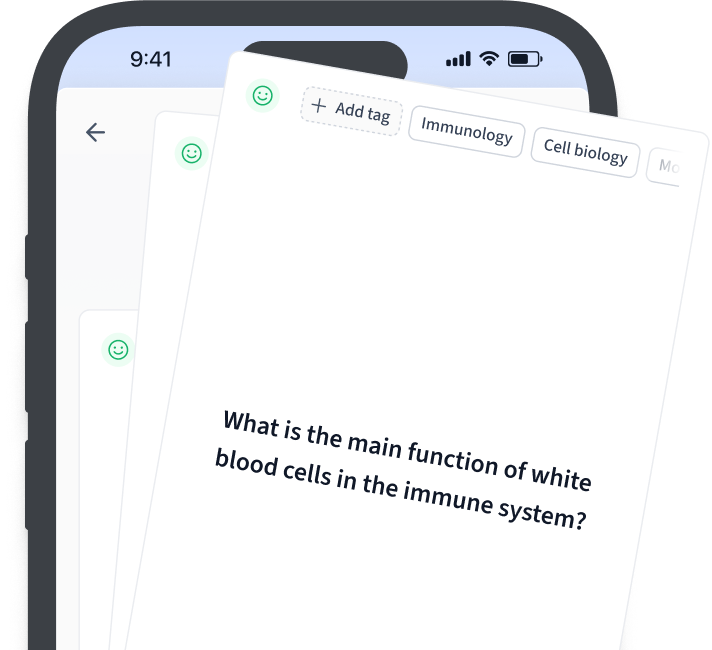
Frequently Asked Questions about Thermal Cycling
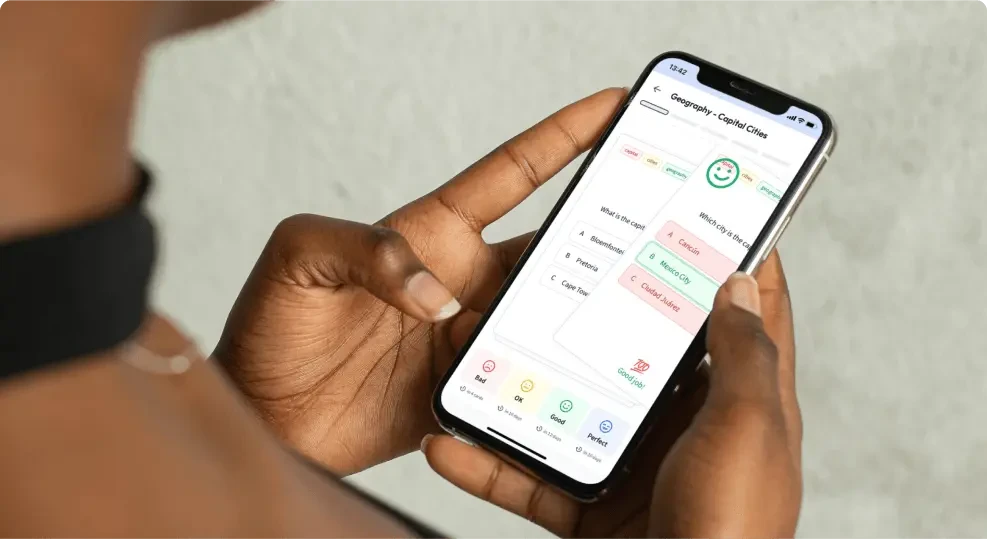
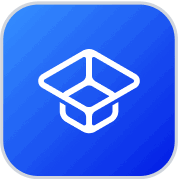
About StudySmarter
StudySmarter is a globally recognized educational technology company, offering a holistic learning platform designed for students of all ages and educational levels. Our platform provides learning support for a wide range of subjects, including STEM, Social Sciences, and Languages and also helps students to successfully master various tests and exams worldwide, such as GCSE, A Level, SAT, ACT, Abitur, and more. We offer an extensive library of learning materials, including interactive flashcards, comprehensive textbook solutions, and detailed explanations. The cutting-edge technology and tools we provide help students create their own learning materials. StudySmarter’s content is not only expert-verified but also regularly updated to ensure accuracy and relevance.
Learn more