Jump to a key chapter
Understanding Thermodynamic Cycles
Thermodynamic cycles are fundamental to understanding how energy is converted and used in various systems, from car engines to power plants. These cycles are the backbone of engineering principles that govern the operation of machines and devices that we rely on daily.
The Basics of Thermodynamic Cycles
At its core, a thermodynamic cycle consists of a series of processes that involve heat and work, leading to the conversion of energy from one form to another. During a cycle, a working fluid, such as air, water, or refrigerant, undergoes phases of compression, heating, expansion, and cooling, returning to its original state by the end of the cycle.
There are several key principles that define these cycles:
- The First Law of Thermodynamics, which states that energy cannot be created or destroyed, only transformed.
- The Second Law of Thermodynamics, which indicates that entropy, or disorder, always increases in an isolated system.
These principles ensure that despite energy transformations, the overall efficiency and possibility of a cycle are constrained by natural laws.
Thermodynamic Cycle: A path of transformation that a working fluid takes through a series of thermodynamic processes, returning to its initial state, enabling the continuous conversion of heat energy into mechanical work and vice versa.
A classic example of a thermodynamic cycle is the Carnot cycle, which consists of two isothermal (constant temperature) processes and two adiabatic (no heat transfer) processes. It is considered an ideal cycle since it defines the maximum possible efficiency any heat engine can achieve operating between two temperatures.
While all thermodynamic cycles aim for high efficiency, real-world applications often face efficiency losses due to friction and unintended heat transfer.
Key Components in Thermodynamic Cycles
Understanding the components that make up thermodynamic cycles is crucial for grasping how they work. Here are some of the key components:
- Working Fluid: The substance that moves through the cycle, absorbing and releasing energy.
- Heat Source: Provides the necessary heat to the working fluid, causing it to expand.
- Heat Sink: Absorbs the excess heat from the working fluid, allowing it to cool and contract.
- Compressor or Pump: Increases the pressure of the working fluid, preparing it for the heat addition process.
- Turbine or Engine: The component where the working fluid does work on, producing mechanical or electrical energy.
Here is a simple table summarising the role of each component:
Component | Function |
Working Fluid | Moves through the cycle, absorbing and releasing energy |
Heat Source | Heats the working fluid |
Heat Sink | Cools the working fluid |
Compressor/Pump | Increases fluid pressure |
Turbine/Engine | Converts fluid's energy into mechanical or electrical energy |
Efficient design and the choice of components dramatically affect the performance and efficiency of a thermodynamic cycle.
One of the most influential factors in the efficiency of thermodynamic cycles, particularly in power generation and refrigeration, is the working fluid's thermodynamic properties. The choice of fluid, such as ammonia for refrigeration cycles or water for steam cycles, plays a crucial role in determining the system's efficiency and capability. Advances in materials science and thermodynamics continue to explore new substances and composites that can withstand higher pressures and temperatures, potentially unlocking higher efficiencies and more sustainable energy solutions.
Rankine Thermodynamic Cycle Explained
The Rankine cycle is a pivotal concept in thermodynamics and engineering, playing a critical role in the generation of electricity in power plants. This cycle is specifically designed for steam turbines, enabling the conversion of heat energy into mechanical work, which can then be used to generate electricity.
How the Rankine Cycle Powers Steam Turbines
The Rankine cycle describes the process by which water is turned into steam and then used to drive a steam turbine. This cycle involves four main stages: boiling or evaporation, expansion, condensation, and finally, compression (or pumping). It starts with the boiling of water into steam at high pressure, which is then expanded through a turbine to create mechanical work. After expansion, the steam is cooled and condensed back into water, which is then pumped back into the boiler to restart the cycle.
Here's a brief overview of each step in the Rankine cycle:
- Boiling/Evaporation: Water is heated in a boiler until it becomes high-pressure steam.
- Expansion: The high-pressure steam expands through a turbine, creating mechanical work.
- Condensation: Expanded steam is then condensed back into water in a condenser.
- Compression: A pump returns the condensed water to the boiler, increasing its pressure and completing the cycle.
The Rankine cycle's efficiency can be significantly enhanced by superheating the steam before it enters the turbine and by reheating it between the high- and low-pressure stages of the turbine.
Efficiency Improvements in the Rankine Cycle
Improving the efficiency of the Rankine cycle involves several strategies, focusing primarily on minimising energy losses and maximising the conversion of heat into work. One approach is to increase the temperature and pressure at which the water boils, known as superheating. Additionally, reheat and regenerative cycles can be incorporated to further improve efficiency.
Key strategies include:
- Superheating: Increasing the steam temperature above its boiling point at a given pressure to increase the turbine's power output.
- Reheating: After partial expansion in the turbine, steam is sent back to the boiler to be reheated before it goes through additional expansion stages, reducing moisture content in the later stages and improving efficiency.
- Regenerative feedwater heating: Utilising steam extracted from the turbine to preheat the boiler feedwater, which reduces the amount of fuel needed to reach the boiling point.
These improvements not only boost efficiency but also extend the life of the steam turbine by reducing thermal stress and erosion caused by moisture in the steam.
An advanced efficiency improvement is the combined cycle, where a Rankine cycle is used in conjunction with a Brayton cycle (gas turbine cycle). In this setup, the waste heat from the gas turbine is used to generate steam for a steam turbine, significantly enhancing the overall efficiency of power generation. This combined cycle concept is key in modern high-efficiency power plants, especially those using natural gas.
Rankine Cycle: A thermodynamic cycle which converts heat into mechanical work, used primarily in steam turbines at power plants. The cycle includes stages of boiling (or evaporation), expansion, condensation, and compression (or pumping).
Consider a power plant utilising the Rankine cycle where water is heated to become steam with a temperature of 600°C and pressure of 15 MPa. The steam then expands through a turbine, dropping to a pressure of 0.008 MPa, and is finally condensed at this low pressure. Improvements such as superheating and reheating can result in efficiency gains, making the cycle more effective for power generation.
Diesel Cycle in Thermodynamics
The Diesel cycle is a cornerstone of engineering, central to the operation of diesel engines found in everything from small generators to large ship engines. Unlike petrol engines that operate on the Otto cycle, diesel engines compress air at a higher ratio before fuel injection and combustion, contributing to their efficiency and power.
The Process of the Diesel Cycle
The Diesel cycle entails a series of steps that convert the chemical energy of fuel into mechanical work. This process is characterised by four main stages:
- Intake: Air is drawn into the cylinder.
- Compression: The air is compressed, resulting in a significant temperature rise.
- Combustion: Fuel is injected into the highly compressed air, igniting and expanding the air-fuel mixture.
- Exhaust: The combustion gases are expelled from the cylinder, preparing it for the next cycle.
This cycle's efficiency comes from the high compression ratio, which leads to higher temperatures and pressures, allowing the engine to extract more work from a given amount of fuel.
Diesel Cycle: A thermodynamic cycle utilised by diesel engines that involves air compression, fuel injection at high pressure, combustion, and exhaust. It is distinguished from other cycles by its high compression ratio and combustion process, which does not require an external ignition source like a spark plug.
Imagine a diesel engine in a large lorry. As the engine runs, air is pulled into the cylinders during the intake phase. The engine then compresses this air to a high degree, significantly raising its temperature. When the air is sufficiently compressed, diesel fuel is injected into the cylinder; it spontaneously ignites due to the high air temperature. The combustion forces the piston down, generating the power needed to move the lorry. Finally, the exhaust valves open, releasing the spent gases and readying the cylinder for the next intake of air.
The absence of spark plugs or ignition systems in diesel engines highlights the importance of high air compression, making the cycle distinct and efficient for heavy-duty applications.
Comparing Diesel and Otto Cycles in Thermodynamics
While both the Diesel and Otto cycles are fundamental to engine operation, they exhibit key differences impacting efficiency, performance, and application areas. Here's a comparison:
Diesel Cycle | Otto Cycle |
Utilises air compression for combustion | Uses a spark for combustion |
Higher compression ratio | Lower compression ratio compared to Diesel |
Better efficiency at converting fuel to energy | Typically faster response but less fuel-efficient |
Used in heavy machinery and transport | Common in passenger vehicles and petrol engines |
This contrast underpins why diesel engines, governed by the Diesel cycle, are preferred for applications requiring longevity and high torque, such as in trucks and buses, whereas the Otto cycle's swift response and lighter weight benefits personal cars and light-duty vehicles.
An interesting detail of diesel engines is their adaptability to different fuel grades, including biodiesel or vegetable oil, without significant modifications. This versatility, combined with the diesel cycle's inherent efficiency, paves the way for sustainable alternatives to traditional diesel fuel. Moreover, innovations in diesel engine technology continue to reduce emissions and improve air quality, addressing one of the main concerns associated with diesel engines.
Despite their differences, both cycles play integral roles in modern transportation, pushing the boundaries of efficiency, performance, and environmental friendliness.
Exploring Other Thermodynamic Cycles
Otto Cycle in Thermodynamics: A Comprehensive Overview
The Otto cycle is a cornerstone of thermodynamics that powers the majority of the world's petrol engines, from the small engines in motorcycles to the larger ones in cars. This cycle consists of four theoretical stages: intake, compression, combustion (or power stroke), and exhaust. By converting heat into work, the Otto cycle drives the pistons within an engine, converting fuel into motion.
The process begins with the intake of a fuel-air mixture, followed by compression, which makes the mixture more combustible. After compression, the spark plug ignites the mixture, resulting in combustion that pushes the piston down in the power stroke. Finally, the exhaust valve opens to expel the combustion gases, completing the cycle.
Otto Cycle: A thermodynamic cycle that describes the functioning of a typical spark-ignition piston engine. It is characterised by a high thermal efficiency and is used primarily in internal combustion engines that power most petrol vehicles.
Imagine a typical four-stroke petrol engine in a car. During the first stroke (intake), the piston moves down and draws in a mixture of petrol and air. The second stroke (compression) sees the piston compress this mixture. A spark then ignites it, causing the third stroke (combustion), where the piston is pushed down. Finally, during the fourth stroke (exhaust), the piston moves up, pushing out the burnt gases.
The Otto cycle not only powers most automotive engines but also serves as a fundamental example in engineering studies, highlighting the practical application of thermodynamic principles. Innovations in engine technology, such as variable valve timing and cylinder deactivation, continue to augment the Otto cycle's efficiency and environmental friendliness in modern internal combustion engines.
Power Cycle Thermodynamics in Everyday Applications
Power cycle thermodynamics underpin the functioning of a diverse array of systems and machines beyond traditional engines, including electrical power stations and heating systems. These cycles are critical for the conversion of energy from sources such as nuclear, solar, or chemical forms into electricity or mechanical work. The Rankine cycle, for instance, is widely used in power generation through steam turbines.
Everyday applications of power cycle thermodynamics can be found in refrigerators, air conditioners, and even in the emerging technology of electric vehicle regenerative braking systems, which convert the vehicle's kinetic energy back into electrical energy to recharge the battery.
The efficiency and environmental impact of power cycles are a significant area of research, leading to innovations like cogeneration or combined heat and power (CHP), which aims to use the waste heat from electricity generation for heating purposes, drastically increasing overall efficiency.
Heat Pump Cycle Thermodynamics: How Heat Pumps Work
Heat pump cycle thermodynamics illustrate how heat can be transferred from a cooler space to a warmer space, contrary to the natural flow of heat. Using a cycle that involves the compression and expansion of refrigerant, heat pumps can provide heating or cooling to buildings without generating heat directly through combustion.
The cycle starts with the refrigerant at a low pressure and temperature; it then absorbs heat from the environment (for heating applications) or from inside the premises (for cooling). The refrigerant is then compressed, increasing its temperature, and moved to the area where heat is needed, releasing the absorbed heat to warm up the space. For cooling, this process is reversed.
Heat Pump Cycle: A thermodynamic cycle that moves heat from one location to another, rather than generating heat directly. It can be used both for heating and cooling purposes, making it highly versatile and efficient for climate control in buildings.
In a residential heating scenario, a heat pump extracts heat from the outdoor air—even in winter—and compresses the refrigerant to increase its temperature. This high-temperature refrigerant then releases its heat inside the house, warming it. The cycle then repeats, continually moving heat from outside to inside the home.
Despite their efficiency, the performance of heat pumps can vary with the external temperature, making them more effective in moderate climates.
Refrigeration Cycle Thermodynamics: From Theory to Application
Refrigeration cycle thermodynamics are crucial to understanding how refrigerators, freezers, and air conditioners keep our food fresh and our environments comfortable. This cycle operates in a manner similar to the heat pump cycle but is typically used for cooling applications. It involves the evaporation of a refrigerant to absorb heat from the interior of a fridge or a room, and then the compression and condensation of the refrigerant to expel that heat to the exterior.
The refrigeration cycle's ability to move heat against its natural direction—from a cooler to a warmer location—makes it an essential technology for modern living, allowing for the preservation of food, medications, and the creation of comfortable living and working spaces.
Refrigeration Cycle: A thermodynamic cycle used in cooling applications, working by compressing refrigerant to expel heat outside the system and then evaporating it to absorb heat from within, thereby cooling the interior space.
A common application of the refrigeration cycle is in household refrigerators. The refrigerator extracts heat from its interior, keeping the food inside cool. The absorbed heat is then expelled to the room outside, ensuring that the internal temperature remains lower than the room temperature.
Recently, environmental and efficiency concerns have prompted significant research into refrigeration cycles, focusing on alternative refrigerants with lower global warming potential and the enhancement of cycle efficiency through innovative technologies like magnetic cooling, which represents a potential future avenue for refrigeration technology that could offer greater environmental benefits and energy savings.
Thermodynamic Cycles - Key takeaways
- Thermodynamic Cycles: Involve a series of processes that include heat and work, converting energy from one form to another through a working fluid in a cyclical process.
- First Law of Thermodynamics: Energy cannot be created or destroyed.
- Second Law of Thermodynamics: Entropy always increases in an isolated system.
- Rankine Thermodynamic Cycle: Used in steam turbines at power plants, involving stages of boiling, expansion, condensation, and compression.
- Diesel Cycle in Thermodynamics: Used by diesel engines with high compression ratios leading to higher temperatures and pressures for improved efficiency.
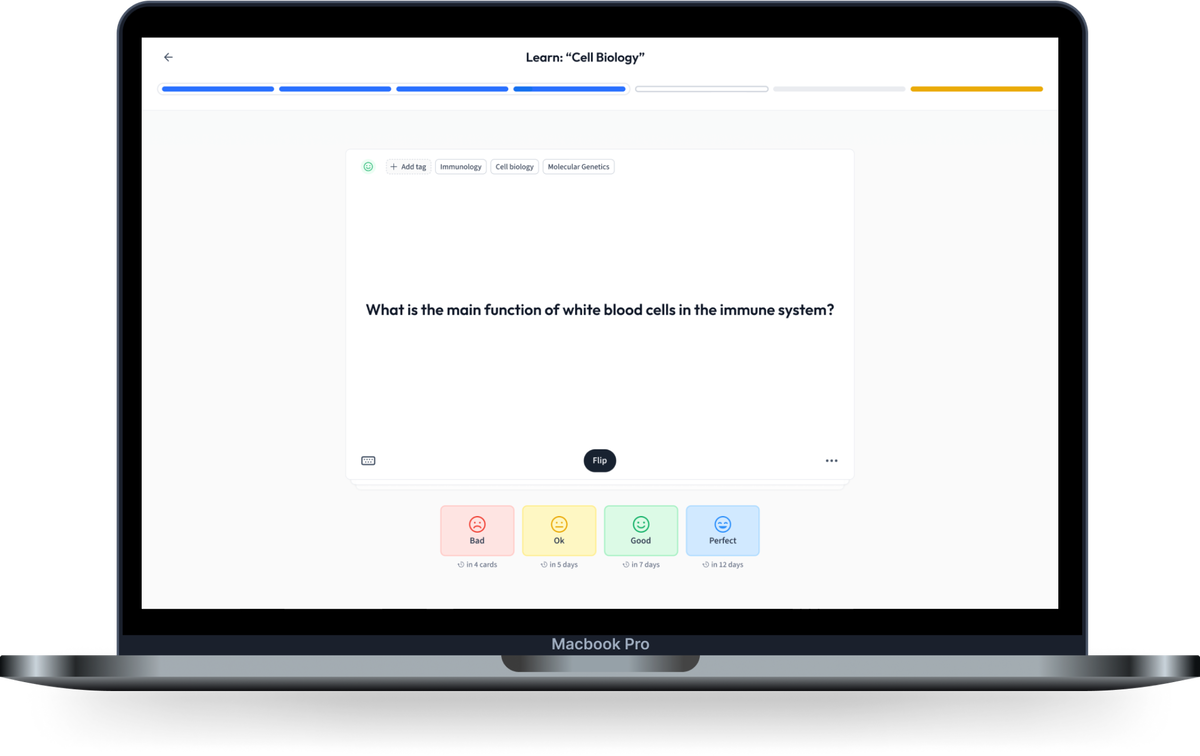
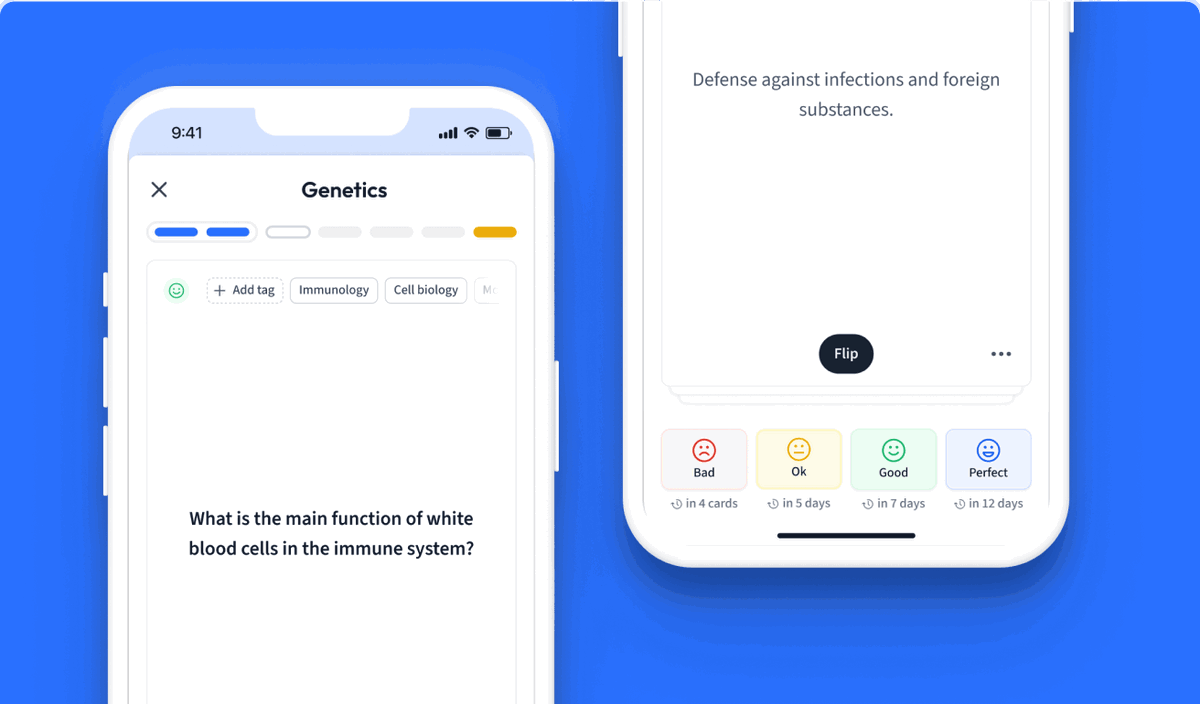
Learn with 12 Thermodynamic Cycles flashcards in the free StudySmarter app
Already have an account? Log in
Frequently Asked Questions about Thermodynamic Cycles
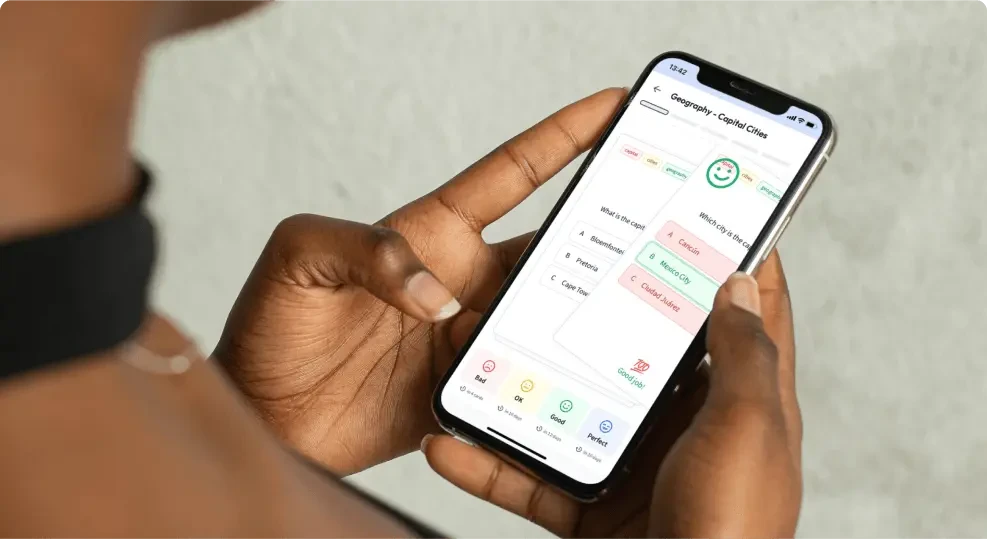
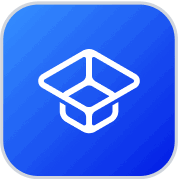
About StudySmarter
StudySmarter is a globally recognized educational technology company, offering a holistic learning platform designed for students of all ages and educational levels. Our platform provides learning support for a wide range of subjects, including STEM, Social Sciences, and Languages and also helps students to successfully master various tests and exams worldwide, such as GCSE, A Level, SAT, ACT, Abitur, and more. We offer an extensive library of learning materials, including interactive flashcards, comprehensive textbook solutions, and detailed explanations. The cutting-edge technology and tools we provide help students create their own learning materials. StudySmarter’s content is not only expert-verified but also regularly updated to ensure accuracy and relevance.
Learn more