Jump to a key chapter
What is Thermoelectric Cooling?
Thermoelectric cooling represents a solid-state cooling method that operates on the Peltier effect. Unlike traditional cooling mechanisms, which rely on fluid dynamics and chemical refrigerants, thermoelectric cooling utilises electricity to move heat from one side of the device to the other, creating a cold surface and a hot surface. This mechanism is widely appreciated for its reliability, lack of moving parts, and the ability to cool objects to below ambient temperature.
Understanding Thermoelectric Cooling Principles
The thermoelectric cooling process is governed by the Peltier effect, a phenomenon discovered in the 19th century. When electric current passes through the junction of two different types of materials, it causes a heat differential. This means, heat is absorbed at one junction and released at another, resulting in a cooling effect. The efficiency and cooling power of thermoelectric coolers (TECs) are influenced by various factors including the materials used, the magnitude of the electric current, and the ambient conditions.
Peltier effect: A thermoelectric phenomenon where heat is absorbed or released when an electric current passes through the junction of two different materials.
Example: Imagine a thermoelectric cooler used in a portable cooler box. When connected to a power source, electricity flows through the thermoelectric module, causing one side to become cold while the other side heats up. This cold side is used to keep the items inside the cooler box at a lower temperature than the surrounding environment.
The Peltier effect not only governs the cooling capacity but also the heating capability of thermoelectric devices. This means, by reversing the direction of the electric current, the same device can be used to heat an object. Such versatility is what makes thermoelectric cooling particularly useful in applications where precise temperature control is critical.
Key Components of a Thermoelectric Cooling Device
A thermoelectric cooling device consists of several key components that enable it to efficiently transfer heat. These include:
- Thermoelectric modules: The core component that facilitates the Peltier effect. It comprises multiple Peltier couples made from semiconductors.
- Heat sinks: Attached to both sides of the thermoelectric modules to facilitate heat absorption and dispersion.
- Electric power source: Supplies the necessary current for the cooling process.
- Cold and hot sides: The physical areas of the device where heat is absorbed and released, respectively.
The effectiveness of a thermoelectric cooler can be greatly influenced by the efficiency of its heat sinks, as these are essential for dissipating heat away from the device.
How Does a Thermoelectric Cooler Work?
Thermoelectric coolers (TECs), based on the Peltier effect, offer an intriguing method of temperature control. By leveraging the relationship between electricity and heat transfer, these solid-state devices provide cooling without using traditional refrigerants or complex mechanical parts. This mechanism of action is both fascinating and elegantly simple, making TECs popular in a variety of applications where reliability and precision are key.
The Mechanics Behind Thermoelectric Cooling
In the heart of thermoelectric cooling lies a process called the Peltier effect. This effect occurs when an electric current is passed through two distinct types of materials connected in series. Due to the difference in electrical conductivity and energy absorption of these materials, heat is absorbed on one side of the junction and released on the other. The result is a cold side, which can be used to cool items or spaces, and a hot side, which is typically fitted with heat sinks to disperse the heat into the surrounding environment. The efficiency of this process is highly dependent on the materials used, the amount of electric current, and the ambient temperature conditions.
Example: Consider a small electronic device that needs to be maintained at a consistent temperature to operate correctly. A thermoelectric cooler can be integrated into the design, with its cold side directed towards the device. When powered on, the TEC absorbs heat from the device, transferring it to the hot side, where it is dispersed into the air by a heat sink.
The efficiency of a thermoelectric cooler is often described in terms of its coefficient of performance (COP). This metric evaluates how effectively the device converts electrical energy into cooling power. High-performance materials such as Bismuth Telluride are commonly used in TEC modules to maximise this efficiency.
Reversing the current's direction in a TEC will invert its function, turning the previously cold side hot, and vice versa. This reversibility allows for both cooling and heating applications, such as in climate-controlled seats in vehicles.
From Theory to Practice: Operating a Thermoelectric Cooling Device
To effectively operate a thermoelectric cooling device, understanding its core components and functionality is crucial. A TEC module acts as the active element in the system, directly converting electrical energy into a temperature gradient. Attached to both sides of the module are heat sinks—critical in managing the device's thermal performance by dissipating the absorbed heat into the environment and maintaining the cold side at a lower temperature. Ensuring efficient heat sink performance is key to achieving effective cooling.
Powering a TEC requires a direct current (DC) power source, the voltage of which will determine the rate of heat transfer. The exact specifications for operating a TEC—such as voltage, current, and maximum temperature differential—are determined by its design and intended application. Here are general steps for operating a typical thermoelectric cooling device:
- Connect the TEC module to a suitable DC power source, observing the correct polarity.
- Apply power to the module, starting at a low voltage and gradually increasing to the desired level based on the cooling needs.
- Monitor the temperature differential across the TEC to ensure it remains within safe operating limits.
- Adjust the power input as necessary to provide the required level of cooling.
- Use thermal insulation around the cooled area to maximise efficiency.
In designing and optimising a system with a thermoelectric cooler, one must consider not just the TEC itself but also the ambient environment and the specific thermal properties of the object or space to be cooled. Factors such as ambient temperature, airflow around the heat sinks, and thermal conductivity of the TEC's surroundings will significantly impact the overall performance. Advanced applications might integrate temperature sensors and adjustable power supplies to dynamically control the cooling process, adapting in real-time to changes in ambient conditions or cooling requirements.
Thermoelectric Cooler Efficiency
Thermoelectric cooler (TEC) efficiency is a measurement of how effectively these devices convert electrical energy into a temperature differential, applying the Peltier effect. It's an essential aspect to consider, especially in applications where energy conservation and cost-effectiveness are prioritised alongside cooling performance.
Factors Affecting Thermoelectric Cooling Element Performance
The performance of a thermoelectric cooling element is influenced by a series of factors, each playing a crucial role in determining the overall efficiency and effectiveness of the cooling process.
- Material Quality: The types of semiconductor materials used in the TEC can significantly affect efficiency. Materials with a higher thermoelectric figure of merit (ZT) offer better performance.
- Temperature Differential: The difference in temperature between the hot and cold sides of the TEC. A greater differential demands more power, reducing efficiency.
- Heat Sink Performance: Effective heat dissipation is crucial. Poor heat sink performance can lead to higher temperatures on the hot side, reducing the cooling capability.
- Electrical Current and Voltage: The amount of current running through the TEC and the voltage applied can impact its operational efficiency. Optimal levels depend on the specific TEC design and application.
- Ambient Conditions: External temperature and airflow can affect heat dissipation from the hot side. Ideally, ambient conditions should support efficient heat removal.
Maintaining an optimal temperature differential across the TEC can help in maximising efficiency, as extreme differentials can significantly increase power consumption.
Maximising Efficiency in Thermoelectric Coolers
To maximise the efficiency of thermoelectric coolers, several strategies can be employed, focusing on optimising component performance and reducing unnecessary power consumption.
- Utilising High-Quality Materials: Selecting TECs made from materials with a high ZT value can enhance efficiency.
- Optimising Heat Sink Design: Improved heat sink designs that promote better heat dispersion can prevent overheating and improve cooling efficiency.
- Electrical Optimisation: Running the TEC at its optimal voltage and current settings according to manufacturer specifications ensures better performance.
- Thermal Insulation: Proper insulation around the cooled area can minimize heat leakage, enhancing the overall efficiency of the thermoelectric cooling system.
- Environmental Control: Maintaining favourable ambient conditions, such as cooler temperatures and good airflow, can improve heat removal from the hot side.
Thermoelectric figure of merit (ZT): A dimensionless unit that measures the efficiency of a thermoelectric material. A higher ZT value indicates better thermoelectric performance.
Example: A thermoelectric cooler operating in an environment with controlled ambient temperature and using materials with a high ZT value can achieve greater efficiency. When paired with an optimised heat sink design, this setup ensures maximum heat dissipation, thereby maximising the cooling effect with reduced energy consumption.
Optimising the design of a thermoelectric system goes beyond merely selecting high-quality components. It involves a comprehensive approach that considers electrical consumption, thermal management, and environmental impact. For instance, integrating thermal insulating materials around the cooled space can significantly reduce thermal exchange with the surroundings, thus lowering the workload on the thermoelectric cooler and leading to energy savings. Moreover, the deployment of smart control systems that dynamically adjust the input power to the TEC based on real-time temperature measurements can further enhance efficiency, ensuring that the device consumes only as much power as needed to maintain the desired temperature.
Advancements in Thermoelectric Cooling
Thermoelectric cooling technology has seen significant advancements in recent years. Driven by demands for more efficient, compact, and environmentally friendly cooling solutions, these innovations have expanded the potential applications of thermoelectric coolers (TECs). The sector continues to evolve, with research and development focusing on enhancing the performance and applicability of thermoelectric elements.
Innovations in Thermoelectric Cooling Elements
The core innovations in thermoelectric cooling elements are centred around material science and system-level integration enhancements. Scientists and engineers have made leaps in identifying and synthesising new materials with higher thermoelectric performance, leading to more efficient and effective cooling solutions.
- Development of materials with higher thermoelectric figures of merit (ZT).
- Improvements in the module manufacturing process to reduce thermal resistance and enhance durability.
- Integration of advanced heat sink designs for better heat dissipation.
Thermoelectric figure of merit (ZT): A dimensionless metric used to assess the efficiency of a thermoelectric material. It combines the material's electrical conductivity, thermal conductivity, and Seebeck coefficient (thermoelectric sensitivity). High ZT materials are more effective at converting temperature differences into electrical voltage and vice versa.
Example: Recent advancements include the discovery of nanostructured materials such as bismuth telluride-based alloys, which have shown significant improvement in thermoelectric efficiency. These nanostructures help to decrease the thermal conductivity while maintaining or enhancing the electrical conductivity and Seebeck coefficient, leading to higher ZT values.
Adoption of computational modelling and machine learning has accelerated the discovery of new thermoelectric materials and the optimisation of existing ones.
The Future of Thermoelectric Cooling Technology
Looking forward, the future of thermoelectric cooling technology holds promising potential. With ongoing advancements in material science and thermal management techniques, the efficiency and applicability of TECs are expected to reach new heights. Anticipated future trends include:
- Increasing use of thermoelectric systems in sustainable and renewable energy applications.
- More compact and efficient thermoelectric modules for consumer electronics cooling, automotive applications, and medical devices.
- Integration of TECs with IoT devices for enhanced environmental control.
One particularly exciting area of development is the potential use of thermoelectric coolers in harvesting waste heat from industrial processes and converting it into usable electricity. This application aligns with global sustainability goals, offering a pathway to increase energy efficiency across industries. Additionally, the miniaturisation of thermoelectric modules could revolutionise cooling and heating in wearable technologies, providing personalised thermal comfort solutions without the bulk and inefficiency of traditional thermal management systems.
Advancements in flexible thermoelectric materials open the door for their incorporation into a wide range of wearable and flexible electronics, marking a significant shift in how thermal management can be achieved in such applications.
Thermoelectric Cooling - Key takeaways
- Thermoelectric Cooling: A solid-state method utilizing electricity to transfer heat from one side of a device to another, based on the Peltier effect, for reliable and precise temperature control.
- Peltier Effect: Heat is absorbed or released when electric current passes through the junction of two different materials, central to the function of thermoelectric coolers (TECs).
- Key TEC Components: Thermoelectric modules comprising Peltier couples, heat sinks for heat dispersal, an electric power source, and distinct cold and hot sides.
- Coefficient of Performance (COP): Used to evaluate a TEC's efficiency in converting electrical energy into cooling power, influenced by materials' quality and environmental conditions.
- Advancements in Thermoelectric Materials: Innovation focusing on higher thermoelectric figures of merit (ZT) materials and system integration for efficient, compact, and environmentally friendly cooling solutions.
Learn faster with the 12 flashcards about Thermoelectric Cooling
Sign up for free to gain access to all our flashcards.
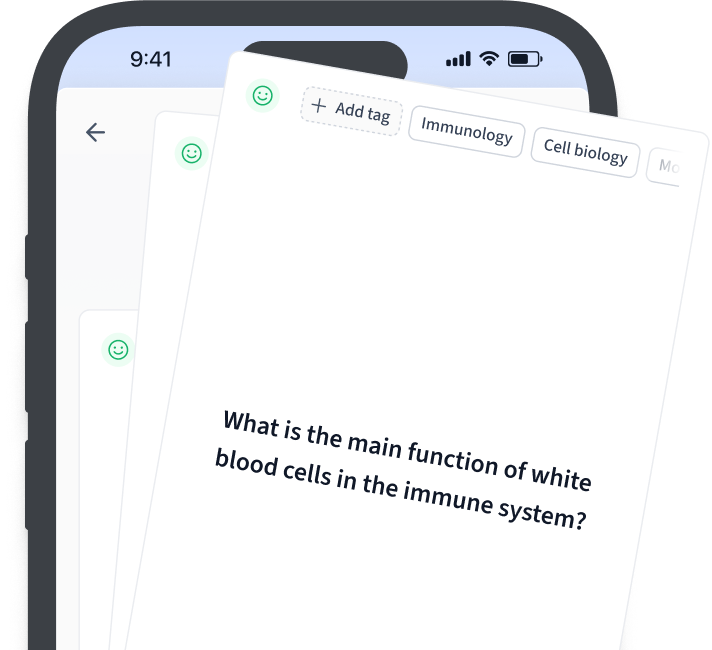
Frequently Asked Questions about Thermoelectric Cooling
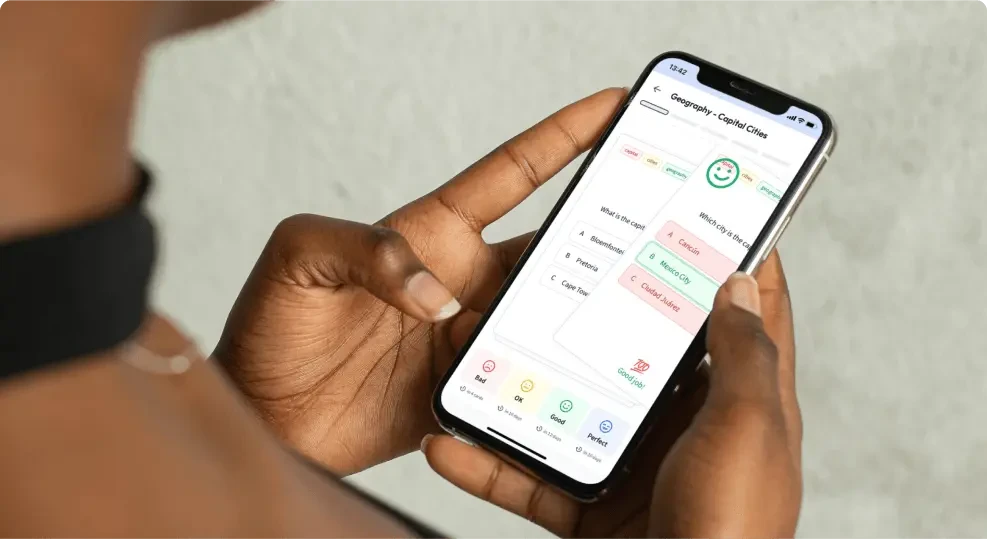
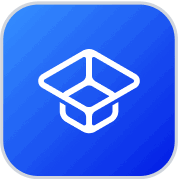
About StudySmarter
StudySmarter is a globally recognized educational technology company, offering a holistic learning platform designed for students of all ages and educational levels. Our platform provides learning support for a wide range of subjects, including STEM, Social Sciences, and Languages and also helps students to successfully master various tests and exams worldwide, such as GCSE, A Level, SAT, ACT, Abitur, and more. We offer an extensive library of learning materials, including interactive flashcards, comprehensive textbook solutions, and detailed explanations. The cutting-edge technology and tools we provide help students create their own learning materials. StudySmarter’s content is not only expert-verified but also regularly updated to ensure accuracy and relevance.
Learn more