Jump to a key chapter
Understanding Turbo Machinery
Turbo machinery occupies a vital space in the landscape of engineering, powering everything from household appliances to massive industrial systems. At its heart, the principles of turbo machinery involve the transfer of energy between a rotor and a fluid, including both liquids and gases. This energy transfer is crucial for the machinery's operation, enabling it to perform tasks such as propulsion, compression, and energy generation.
Introduction to the principles of turbo machinery
The underlying principles of turbo machinery centre around the concepts of fluid dynamics and thermodynamics. These physical principles guide the design and operation of turbo machines, allowing them to efficiently transfer energy. A fundamental aspect to understand is how these machines convert the kinetic and potential energy of fluids into mechanical energy and vice versa.
A core element of this is the impeller or rotor, which is the moving part of the turbo machinery that imparts energy to the fluid. These energy exchanges are governed by two key principles: the Conservation of Mass and the Conservation of Energy. Understanding these principles is essential for grasping the operation of any turbo machinery.
The efficiency of turbo machinery significantly depends on the design of the rotor or impeller, which is tailored to specific fluid properties and operational conditions.
Turbo Machinery: A class of machines that transfer energy between a rotating component and a fluid (including gases and liquids) through dynamic forces.
An example of turbo machinery is a steam turbine used in power plants. Here, steam (fluid) is directed over the blades of the turbine (rotor), causing it to spin and generating electricity.
Exploring turbo machinery design fundamentals
The design of turbo machinery is a complex endeavour that requires a deep understanding of both fluid dynamics and material science. Key considerations include the shape and size of the impeller, the type of fluid it will interact with, and the desired energy transfer outcomes. Computational Fluid Dynamics (CFD) plays a critical role in this process, enabling designers to simulate and optimise machine performance before any physical model is built.
Additional design factors include:
- Thermal properties of the fluids
- Efficiency of the energy conversion process
- Durability and reliability of the components under operational stresses
In the realm of turbo machinery design, material selection stands out as a critical factor. Materials need to withstand the high temperatures and pressures encountered during operation without degrading. Advanced materials like titanium alloys and ceramic matrix composites are increasingly used for their excellent strength-to-weight ratios and resistance to thermal expansion.
Furthermore, the advent of 3D printing technologies has begun to revolutionise turbo machinery design, allowing for the creation of complex components that were previously impossible to manufacture using traditional methods. This innovation not only improves performance but also potentially reduces the cost and time-to-market for new designs.
The role of turbo machinery in aerospace engineering
In aerospace engineering, turbo machinery is at the heart of propulsion systems. Jet engines, for instance, rely on turbo machinery such as compressors to draw in and compress air, mixing it with fuel for combustion to produce thrust. The design and optimisation of these components are crucial for overall engine performance, affecting factors like thrust, fuel efficiency, and environmental emissions.
The role of turbo machinery in aerospace doesn't stop at propulsion; it also extends to systems such as Auxiliary Power Units (APUs), which provide power for aircraft systems when the main engines aren't running. The challenge in aerospace applications often lies in achieving the highest efficiency and reliability while minimising weight and maximising safety.
Advancements in turbo machinery are closely linked to improvements in aerospace engineering, driving developments in faster, safer, and more efficient air travel.
Turbo Machinery Blade Design
The design of blades in turbo machinery is a pivotal aspect that heavily influences the performance, efficiency, and longevity of turbo machines. These blades interact directly with the working fluid, making their design critical for optimal energy transfer.
At the core of turbo machinery blade design are principles that ensure the blades effectively convert kinetic energy from the working fluid into mechanical energy. This involves precise calculations of shape, size, and angle to minimise energy losses due to friction and turbulence. The design process also takes into account the blade's operating environment, including temperature, pressure, and the type of fluid it encounters.
Key considerations in blade design include:
- Blade geometry (e.g., curvature and twist)
- Material selection based on operational stresses
- Manufacturing techniques that can accurately realise the design
- Aerodynamic efficiency to maximise energy transfer and minimise losses
Turbo Machinery Blade: A component within turbo machinery that interacts with a working fluid to convert kinetic and potential energy into mechanical energy. The design of the blade directly impacts the efficiency and effectiveness of this energy conversion.
For example, in a gas turbine engine, the blades in the compressor section are designed to compress air as it enters the engine, increasing its pressure and temperature before it mixes with fuel for combustion. The specific design of these blades is critical for achieving the desired compression ratio and efficiency.
Materials and manufacturing techniques for blades
The materials used for turbo machinery blades must withstand the high-stress operating conditions such as extreme temperatures, pressure fluctuations, and corrosion. Advanced materials such as titanium alloys, nickel-based superalloys, and ceramic matrix composites are commonly used due to their favourable properties. Manufacturing techniques have also evolved, with precision casting, forging, and additive manufacturing (3D printing) being widely employed to achieve complex blade geometries with high accuracy and strength.
Common materials and their key properties include:
Material | Properties |
Titanium Alloys | Lightweight, high strength, excellent corrosion resistance |
Nickel-based Superalloys | High-temperature strength, creep resistance, oxidation resistance |
Ceramic Matrix Composites | High-temperature resistance, low weight, wear resistance |
Advancements in additive manufacturing have revolutionised the production of turbo machinery blades, allowing for the creation of designs that were previously deemed impractical or impossible. This technology facilitates the manufacture of blades with complex internal cooling passages that improve thermal efficiency and prevent overheating, thereby extending their operational lifespan.
Aerodynamics of blades in turbo machinery
The aerodynamics of turbo machinery blades is fundamental to their design, affecting how effectively they can convert the energy of the working fluid into mechanical energy. An optimised blade design addresses factors such as lift and drag forces, angle of attack, and the boundary layer characteristics to enhance performance. The goal is to achieve smooth, laminar flow over the blade surface, minimising turbulence and ensuring efficient energy transfer.
Key aerodynamic considerations in blade design include:
- Flow path geometry to ensure optimal fluid dynamics
- Surface finish to reduce drag and minimise flow separation
- Blade profile selection to maximise lift-to-drag ratios
- Use of computational fluid dynamics (CFD) software for design optimisation and testing
The inclusion of winglets or serrations at the blade tips can further enhance the aerodynamic efficiency by reducing vortices that cause drag and energy loss.
Turbo Machinery Thermodynamics
Turbo machinery thermodynamics involves the study and application of energy and work interactions between fluids and rotating machinery. This area of engineering plays a crucial role in designing systems that are both efficient and effective, underpinning the operation of a wide range of machines, from household fans to advanced jet engines.
Key concepts in turbo machinery thermodynamics
In the realm of turbo machinery thermodynamics, several key concepts are foundational to understanding and designing efficient systems. First among these is the First Law of Thermodynamics, which focuses on the conservation of energy within a system. Additionally, the Second Law of Thermodynamics, which deals with entropy and the irreversibility of real-world processes, is paramount for optimizing turbo machinery performance.
Other essential concepts include:
- The concept of specific speed, which influences the design and selection of turbo machinery for specific applications
- Adiabatic and polytropic efficiency determinations, critical for estimating the performance of compressors and turbines
Understanding the relationship between temperature, pressure, and volume in fluids helps in optimizing the thermodynamic cycles for turbo machinery.
Entropy: A measure of the amount of energy in a physical system that cannot be used to do work. In turbo machinery, minimizing entropy production is key to maximizing efficiency.
Considering a gas turbine engine, the First Law of Thermodynamics is applied to ensure energy balance across the system, while the Second Law is used to evaluate and minimize the engine's inefficiencies, such as heat loss.
Heat transfer and efficiency in turbo machinery
Heat transfer plays a vital role in the efficiency of turbo machinery. Understanding how heat is absorbed, converted, and dissipated in these systems is key to optimising their performance. Heat exchange can occur through different means such as conduction, convection, and radiation, with each mechanism having its specific implications for the design and operation of turbo machinery.
Efficiency, on the other hand, is typically quantified in terms of adiabatic efficiency for compressors and turbines, and thermal efficiency for cycles. Achieving high efficiency in turbo machinery not only improves performance but also reduces fuel consumption and emissions, making it a critical area of focus in design and operation.
One interesting aspect of heat transfer in turbo machinery is the use of cooling techniques in gas turbine blades. To withstand high operating temperatures, these blades may employ internal cooling passages, external cooling films, or combinations of both. The design and effectiveness of these cooling strategies directly impact the turbine's thermal efficiency and longevity.
Turbo machinery efficiency calculation explained
Calculating turbo machinery efficiency involves comparing the actual performance of the machinery to its ideal performance under the same conditions. For turbines and compressors, this is often done using adiabatic or isentropic efficiency, which measures how closely a machine approaches an ideal, reversible process.
The general formula for calculating efficiency ( extit{ exteta}) can be simplified as:
extit{ exteta} = (Actual Work Output) / (Ideal Work Output)
However, the specific calculation can vary depending on the type of machinery and the process it performs. For instance, thermal efficiency is crucial for evaluating the performance of cycles, like in jet engines or power plants, and involves measuring the ratio of the output work to the heat input.
When calculating efficiency, factors such as friction, heat losses, and aerodynamic drag are considered as they can significantly impact the actual performance of turbo machinery.
Maintaining Turbo Machinery
Maintaining turbo machinery is critical to ensuring the longevity, efficiency, and reliability of these complex systems. It involves routine inspections, vibration analysis, timely diagnosis of common issues, and adherence to best maintenance practices.
Fundamentals of turbo machinery vibration analysis
Vibration analysis stands as a fundamental part of maintaining turbo machinery. It helps in identifying early signs of wear and tear, misalignment, or imbalance that could lead to breakdowns if unaddressed. This type of analysis utilises sophisticated equipment to measure vibration levels and patterns while the machinery is in operation, allowing for real-time monitoring and diagnosis.
Key concepts in vibration analysis include:
- Natural frequency
- Amplitude of vibration
- Harmonic frequencies
- Resonance
Vibration Analysis: A technique used in the maintenance of turbo machinery that involves measuring vibrations to identify abnormalities and predict possible failures in machinery.
Proper alignment and balance of rotating parts are crucial in minimising vibrations and extending the life of turbo machinery.
Diagnosing common issues in turbo machinery
Common issues in turbo machinery can range from simple mechanical wear to more complex thermal or fluid dynamic problems. Diagnosing these issues accurately and promptly is crucial for maintaining operational efficiency and preventing catastrophic failures.
Common issues include:
- Bearing failures
- Blade erosion or corrosion
- Cooling system malfunctions
- Seal leaks
- Imbalance or misalignment
A common issue diagnosed in turbo machinery is blade erosion, which can occur due to the high-speed impact of particles within the fluid. This erosion reduces the efficiency of the blades and, consequently, the overall efficiency of the machinery. Timely detection and repair of eroded blades are essential for preventing further damage.
Bearing failures often signal misalignment or balance issues within turbo machinery. Advanced diagnostic tools, such as thermal imaging cameras and ultrasonic detectors, can be used for early detection of bearing overheating or wear, allowing for proactive maintenance steps to be taken before severe damage occurs.
Best practices for turbo machinery maintenance
Adhering to best practices for turbo machinery maintenance is crucial for ensuring the machinery's reliability and performance. These practices include routine inspections, proper lubrication, vibration analysis, and operating within the machinery’s design limits. Additionally, training personnel on the specifics of the turbo machinery in use and contemporary diagnostic techniques is critical.
Best practices include:
- Regular monitoring of vibration and temperature levels
- Keeping detailed maintenance logs for future reference
- Using the correct grade of lubricants
- Replacing worn parts before they fail
- Calibrating sensors and diagnostic equipment regularly
Implementing a predictive maintenance strategy, using data from vibration analysis and other diagnostics, can significantly increase the uptime and efficiency of turbo machinery.
Turbo Machinery - Key takeaways
- Turbo Machinery: Machines that transfer energy between a rotating component and a fluid, used in applications ranging from household appliances to propulsion systems.
- Principles of Turbo Machinery: Governed by fluid dynamics and thermodynamics, focusing on the energy transfer between the rotor/impeller and the fluid, abiding by the Conservation of Mass and Energy.
- Turbo Machinery Design Fundamentals: Involves complex considerations such as impeller shape and size, fluid thermal properties, and computational fluid dynamics (CFD) for simulation and optimisation.
- Turbo Machinery Blade Design: Critical for machine performance, efficiency, and longevity, with key considerations in blade geometry, material selection, and aerodynamic efficiency to reduce energy losses.
- Turbo Machinery Efficiency Calculation: Assessed using metrics like adiabatic or isentropic efficiency for compressors and turbines, and involves the balance of actual work output versus ideal work output.
Learn faster with the 12 flashcards about Turbo Machinery
Sign up for free to gain access to all our flashcards.
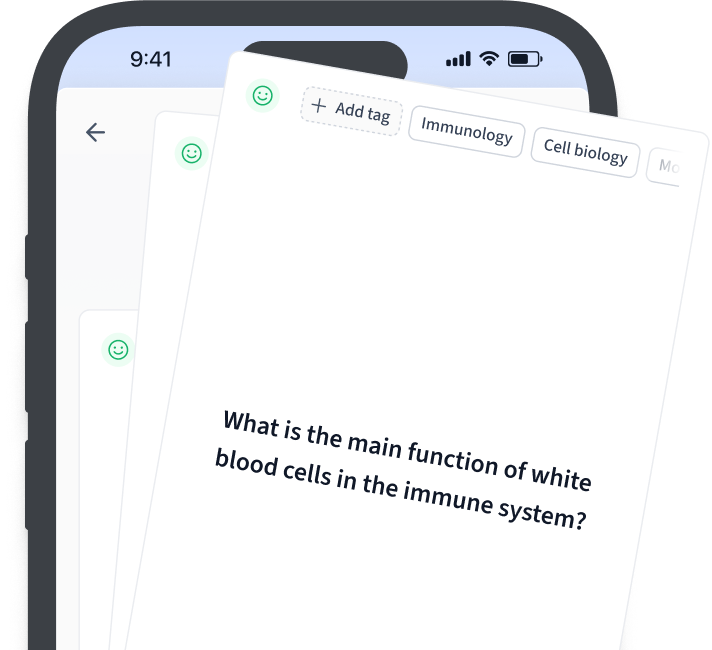
Frequently Asked Questions about Turbo Machinery
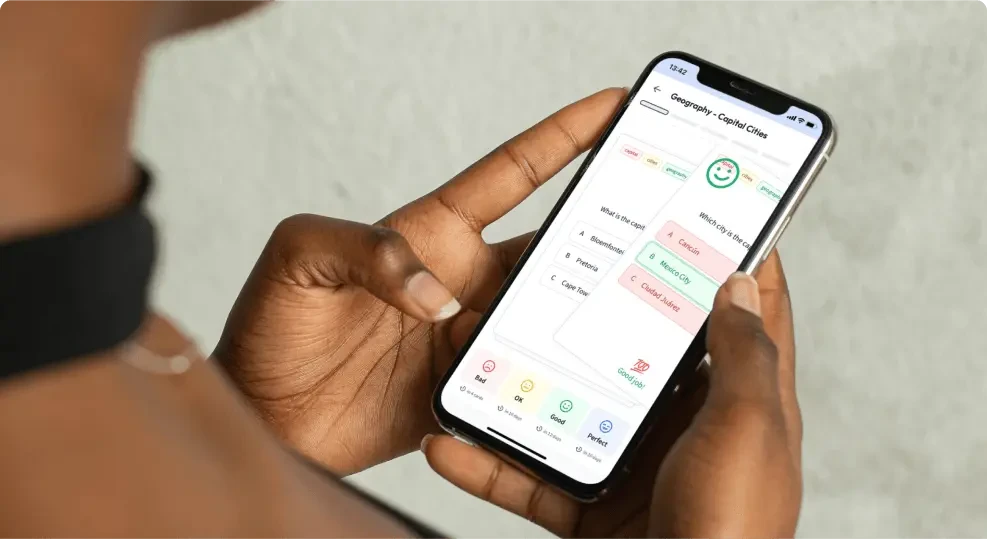
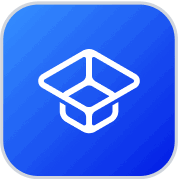
About StudySmarter
StudySmarter is a globally recognized educational technology company, offering a holistic learning platform designed for students of all ages and educational levels. Our platform provides learning support for a wide range of subjects, including STEM, Social Sciences, and Languages and also helps students to successfully master various tests and exams worldwide, such as GCSE, A Level, SAT, ACT, Abitur, and more. We offer an extensive library of learning materials, including interactive flashcards, comprehensive textbook solutions, and detailed explanations. The cutting-edge technology and tools we provide help students create their own learning materials. StudySmarter’s content is not only expert-verified but also regularly updated to ensure accuracy and relevance.
Learn more