Jump to a key chapter
Understanding Vibration Control in Engineering
Vibration control is a critical aspect of engineering that deals with the minimisation or management of vibrations to enhance the performance and lifespan of engineering structures and systems. This field is particularly relevant in situations where vibrations can cause damage, reduce efficiency, or generate undesirable noise levels. As such, effective vibration control is vital for ensuring the safe, efficient, and durable operation of various engineering applications.
The Basics of Vibration Control
At its core, vibration control involves the application of specific techniques and materials to reduce or eliminate vibrations emanating from machines, structures, and other systems. The basic principle is to first identify the sources of vibration, understand how these vibrations are transmitted, and then apply measures to diminish their impact. This process typically involves a combination of passive and active vibration control techniques.
Passive Vibration Control: | Relies on physical mechanisms to absorb or dampen vibrations without the need for external power or control systems. |
Active Vibration Control: | Uses sensors and actuators, powered by an energy source, to counteract vibrations in real time. |
Materials used in passive vibration control often include rubber, springs, and viscoelastic materials, all of which can absorb energy efficiently.
Importance of Vibration Control Techniques in Aerospace Engineering
In the field of aerospace engineering, vibration control is paramount for both the safety and performance of aircraft and spacecraft. Vibrations in aerospace systems can arise from various sources, including engine operation, turbulent airflow, and mechanical feedback. If not adequately controlled, these vibrations can lead to structural failure, impair equipment function, and even endanger lives.
Effective vibration control techniques in aerospace engineering help to:
- Enhance the structural integrity and longevity of aircraft and spacecraft.
- Improve passenger comfort by reducing noise and vibration levels.
- Prevent critical system failures that can arise from prolonged exposure to high-vibration environments.
A notable example of vibration control in aerospace engineering is the use of tuned mass dampers in spacecraft. These dampers are designed to absorb and counteract specific vibration frequencies, thus protecting sensitive components from damage during launch and operation.
How Vibration Control Systems Work
Vibration control systems function by detecting vibrations and then applying counter-vibrations or damping methods to reduce or neutralise the effect of these vibrations. The effectiveness of a vibration control system depends on accurately identifying the frequency, amplitude, and direction of the unwanted vibrations and applying the appropriate control measures at the right time.
An essential component of these systems is the use of sensors to monitor vibrations and actuators to apply the countermeasures. Advanced systems may also include controllers that process the sensor data and dictate the response, ensuring that vibrations are managed efficiently and effectively.
To understand how advanced vibration control systems are designed, one can explore the field of adaptive structures a discipline that integrates materials science, computer algorithms, and mechanical engineering. Such systems can dynamically adjust their properties or behaviour in response to changes in their environment or operation, allowing for real-time control over vibration levels. This approach is particularly effective in scenarios where vibration sources frequently change or in managing complex vibration patterns.
Techniques for Vibration Control
Understanding the diverse techniques for vibration control is essential in engineering to enhance the functionality and longevity of mechanical systems and structures. These techniques are crucial for mitigating the effects of vibrations, which can lead to noise issues, mechanical failures, and decreased system performance.
Introduction to Vibration Control Techniques
Vibration control encompasses a range of methods designed to reduce or eliminate the vibration transmitted from external sources to a mechanical system or to control the vibrations produced within the system itself. It involves an understanding of both the sources of vibration and the transmission pathways, as well as the application of techniques to manage or mitigate these vibrations.
Two main approaches to vibration control are vibration isolation and vibration damping. Isolation seeks to prevent the transmission of vibration from a source to a system, whereas damping aims to dissipate the energy associated with vibration within the system itself.
Vibration Isolation Methods Explained
Vibration isolation refers to the practice of preventing vibrations generated by a source from reaching a particular receiver or system. This technique is typically applied by introducing a barrier or isolator between the source of the vibration and the system to be protected.
Key components in vibration isolation include:
- Isolators: Devices that physically separate the source of vibrations from the surrounding environment or sensitive systems. Examples include rubber mounts, spring systems, and pneumatic isolators.
- Damping materials: Used in conjunction with isolators, these materials absorb and dissipate vibrational energy, further reducing the transmission of vibrations. Common materials include viscoelastic compounds, foam, and rubber.
The effectiveness of vibration isolation depends on selecting the right isolator and material for the specific frequency and amplitude of vibrations to be managed.
Vibration Isolation: A technique used in vibration control to prevent the transmission of vibration from a source to a system or structure by introducing a physical barrier or system between them.
An example of vibration isolation is the use of rubber pads beneath a washing machine. These pads serve as isolators that minimise the transfer of vibrations from the washing machine to the floor, thus reducing noise and preventing structural damage to the building.
Advanced Vibration Damping in Structures
Advanced vibration damping techniques involve the use of sophisticated materials and designs to absorb and dissipate vibrational energy within structures. Unlike basic damping methods that may only target specific frequencies, advanced techniques offer broader solutions that can adapt to varying vibrational characteristics.
Examples of advanced vibration damping include:
- Tuned Mass Dampers (TMDs): These are used in buildings and bridges to reduce oscillations caused by wind or seismic activity. A TMD consists of a mass attached to a building structure via springs or pendulums and moves out of phase with the building's oscillation, thus damping the motion.
- Active Vibration Control: This method involves the use of sensors and actuators to detect and counteract vibrations in real-time, providing an adaptable solution to dynamically changing vibrational forces.
Advanced damping methods are crucial in critical applications such as aerospace, automotive, and structural engineering, where controlling vibration can mean the difference between operational success and failure.
Tuned Mass Dampers not only serve a functional purpose in vibration control but have also become iconic features in some of the world's most renowned structures. A famous example is Taipei 101 in Taiwan, one of the tallest skyscrapers globally, which houses a massive tuned mass damper visible to the public. This TMD is among the largest in the world, weighing 660 metric tonnes, and is a critical component for stabilising the tower against typhoons and earthquakes. Such examples illustrate the intricate balance between engineering solutions and architectural aesthetics, demonstrating how vibration control technologies can become integral elements of design and function.
Components of Vibration Control Systems
When delving into the intricacies of vibration control systems, you'll encounter a variety of components each playing a critical role in minimising unwanted vibrations. These systems are central to ensuring machinery, buildings, and vehicles operate more smoothly, quietly, and reliably. By breaking down the key elements, you can gain a deeper understanding of how these systems work to protect and enhance the performance of various engineering projects.
Key Elements in Vibration Control Systems Explained
Effective vibration control systems incorporate a series of sophisticated elements designed to detect, mitigate, or eliminate undesired vibrations. Central to these systems are dampers, sensors, and actuators, each serving a unique function within the wider context of vibration control.
Together, these components form an integrated framework capable of addressing the challenges presented by vibrational energy in structures and machinery:
- Dampers work to absorb and dissipate the energy generated by vibrations, effectively reducing their amplitude.
- Sensors are used to detect vibrations, measuring their magnitude and frequency to inform the necessary response.
- Actuators apply force in a controlled manner to counteract detected vibrations, based on the feedback from sensors.
Vibration Control System: An assembly of components including dampers, sensors, and actuators designed to detect, mitigate, or eliminate undesirable vibrations in structures and mechanical systems.
The Role of Dampers in Vibration Control
Dampers play a pivotal role in vibration control systems by absorbing and dissipating vibrational energy. These devices can be simple mechanical springs and shock absorbers or more complex viscoelastic, fluid-filled, or friction-based mechanisms. Their effectiveness hinges on their ability to convert kinetic energy from the vibration into another form of energy, typically heat, which is then expelled from the system.
Dampers are particularly effective in applications where passive vibration control is required, serving as the first line of defence against the detrimental effects of vibration.
A common application of dampers is in automotive suspension systems. Here, hydraulic shock absorbers reduce and smooth out the oscillations of the vehicle's springs, improving comfort and handling by controlling the rate at which the springs compress and rebound.
Sensors and Actuators in Vibration Control Systems
In the realm of vibration control, sensors and actuators work together to form a feedback loop that continuously monitors and responds to vibrations.
Sensors measure the frequency, amplitude, and direction of vibrations, providing real-time data that is essential for understanding the dynamics of a vibratory system. This data is then processed by a controller, which determines the appropriate response.
Actuators, on the other hand, take the controller's commands and apply forces to the system to counteract the vibrations. Depending on the system, actuators can apply mechanical, hydraulic, pneumatic, or electrical forces to achieve this goal.
The precision and effectiveness of sensors and actuators are critical for the successful implementation of active vibration control strategies, highlighting the importance of advanced materials and technology in these systems.
One innovative approach in vibration control involves the use of smart materials, such as piezoelectric materials, in sensors and actuators. These materials have the unique ability to convert mechanical strain into electrical charge and vice versa, allowing for the creation of highly responsive vibration control systems. Such systems can adapt to changing vibration patterns in real time, offering superior performance compared to traditional passive systems. This adaptability is particularly beneficial in sectors like aerospace and high-precision manufacturing, where even minimal vibrations can have significant consequences.
Real-World Applications of Vibration Control
Vibration control is a pivotal technology in the engineering field, significantly impacting the design, construction, and maintenance of a wide array of structures and machinery. Its applications span numerous sectors, from aerospace and automotive to construction and manufacturing, each benefiting from the ability to mitigate unwanted vibrations.
Vibration Control in Aerospace Engineering Structures
In aerospace engineering, vibration control is integral to both the safety and performance of aircraft and spacecraft. The high-vibration environment of aerospace applications demands robust solutions to prevent structural damage, ensure operational reliability, and safeguard sensitive components. Techniques such as passive damping, active vibration control, and the use of specialised materials are employed to address these challenges.
For instance, vibration control systems in aerospace applications manage the intense vibrations produced during launch and operation, ensuring the structural integrity of spacecraft and the safety of satellite deployment mechanisms.
Aerospace Engineering: A field of engineering focused on the design, development, testing, and production of aircraft, spacecraft, and related systems and equipment.
A classic example of vibration control in aerospace engineering is the design of engine mounts for jet aircraft. These mounts not only support the weight of the engine but also dampen the vibrations it produces, protecting the airframe and enhancing passenger comfort.
Benefits of Using Vibration Control in Engineering Projects
The integration of vibration control systems in engineering projects offers numerous benefits, significantly enhancing the durability, reliability, and performance of structures and machinery. Key advantages include:
- Increased lifespan of components and structures by reducing wear and tear associated with vibrations.
- Improved operational efficiency and safety by minimising noise and the risk of vibrational damage.
- Enhanced comfort for occupants in buildings and vehicles through reduced vibration transmission.
- Compliance with regulations and standards regarding noise and vibration levels in various industries.
Investing in vibration control technology can lead to substantial cost savings over time, due to reduced maintenance requirements and the extended service life of equipment and infrastructure.
Future Trends in Vibration Control Techniques
The future of vibration control in engineering sees the advent of innovative techniques and materials poised to further enhance the effectiveness of vibration mitigation strategies. Advancements include:
- Development of smart materials and structures that can adaptively respond to changing vibration patterns and intensities.
- Increased use of active vibration control systems powered by artificial intelligence to provide real-time adaptive responses.
- Integration of nanotechnology in the construction of vibration dampers for superior performance in microscale applications.
One particularly promising area is the use of metamaterials, structured materials with engineered properties that can bend, block, or redirect energy waves, including vibrations. These materials offer the potential for revolutionary vibration control solutions that outperform conventional methods, especially in challenging environments such as extreme temperatures or in the vacuum of space. Such innovations are expected to significantly expand the capabilities and applications of vibration control systems in the future.
Vibration Control - Key takeaways
- Vibration control in engineering refers to the minimisation and management of vibrations for improved performance and lifespan of structures and systems.
- There are two primary vibration control techniques: passive vibration control (relying on physical mechanisms without external power) and active vibration control (using sensors and actuators to counteract vibrations).
- Vibration isolation methods prevent vibrations from reaching a system by using isolators and damping materials, while advanced vibration damping absorbs energy within structures through devices like Tuned Mass Dampers (TMDs).
- Components of vibration control systems often include dampers (to absorb energy), sensors (to detect vibrations), and actuators (to counteract vibrations), working together to maintain system stability.
- In aerospace engineering, vibration control is crucial for safety and performance, involving techniques to manage intense vibrations during launch and operation of aircraft and spacecraft.
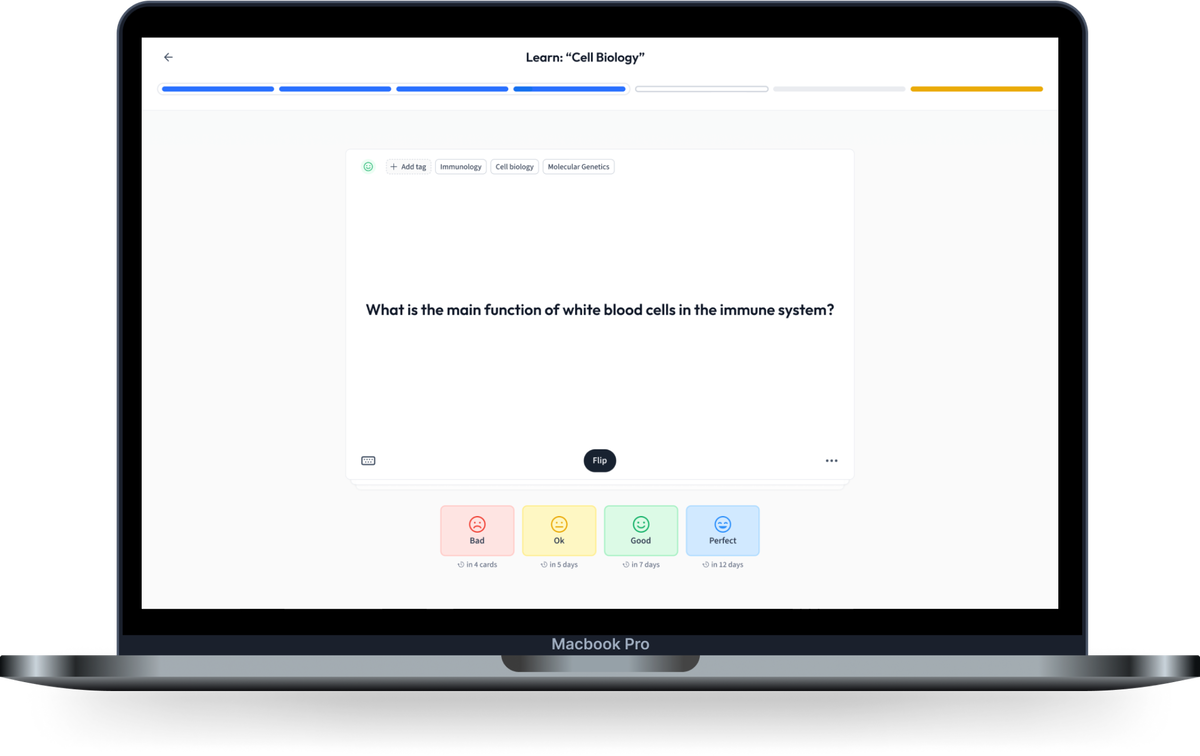
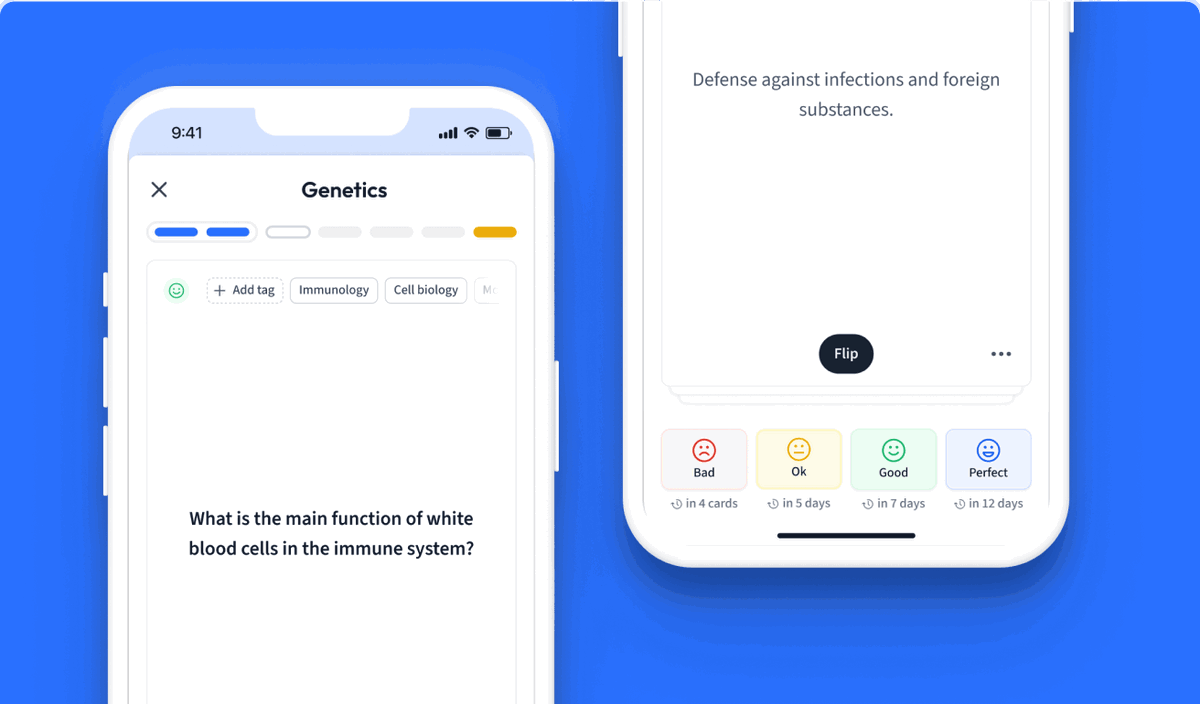
Learn with 12 Vibration Control flashcards in the free StudySmarter app
Already have an account? Log in
Frequently Asked Questions about Vibration Control
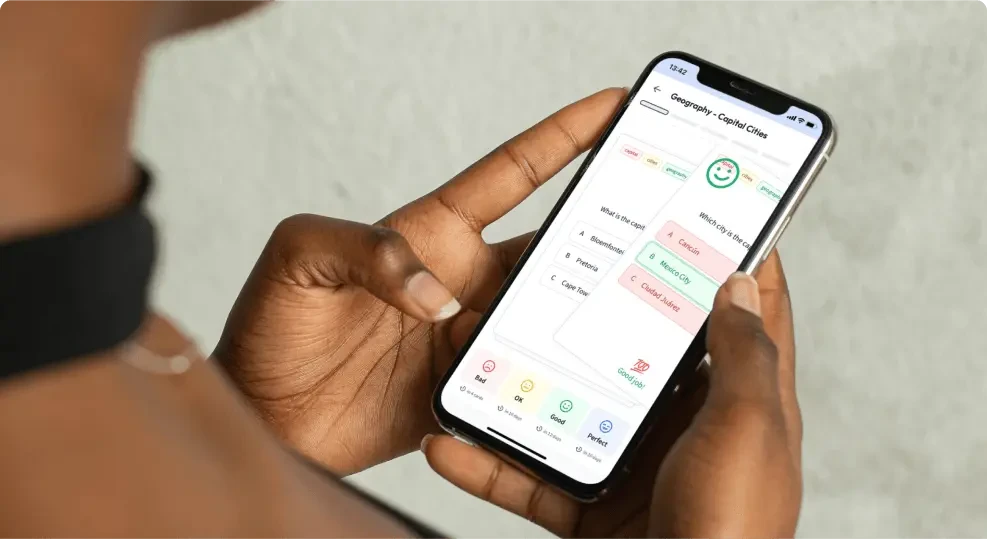
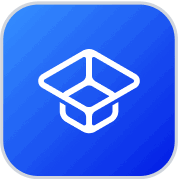
About StudySmarter
StudySmarter is a globally recognized educational technology company, offering a holistic learning platform designed for students of all ages and educational levels. Our platform provides learning support for a wide range of subjects, including STEM, Social Sciences, and Languages and also helps students to successfully master various tests and exams worldwide, such as GCSE, A Level, SAT, ACT, Abitur, and more. We offer an extensive library of learning materials, including interactive flashcards, comprehensive textbook solutions, and detailed explanations. The cutting-edge technology and tools we provide help students create their own learning materials. StudySmarter’s content is not only expert-verified but also regularly updated to ensure accuracy and relevance.
Learn more