Jump to a key chapter
Interconnectivity Definition and Meaning
In engineering, understanding the concept of interconnectivity is essential as it plays a crucial role in the design and functionality of complex systems. Let’s delve into its definition and explore how this concept is implemented.
What is Interconnectivity?
Interconnectivity refers to the state or quality of being connected together. It involves the linking of various distinct systems, networks, or components to work in a unified manner to achieve common goals.
Interconnectivity is a key aspect of modern engineering and technology. It enables multiple systems to communicate and function seamlessly. Consider the example of a computer network where different devices such as printers, computers, and routers are interconnected to share resources and information efficiently. Each component within the network plays a role, and their integration eliminates isolated functionality.
Imagine a smart home system, where interconnectivity allows communication between the thermostat, lights, and security system. When the security system detects you leaving the property, it can automatically reduce heating and turn off lights, optimizing energy usage through interconnected actions.
Interconnectivity is vital in the Internet of Things (IoT), where devices are interconnected to enhance functionality.
Understanding Interconnectivity Meaning
Understanding the meaning of interconnectivity goes beyond just linking devices. It encompasses the interaction and coordination between the connected elements. This coordination allows for more complex functionalities and data exchanges. For instance:
- Resilience: Systems that are highly interconnected can often compensate when one component fails, improving overall system resilience.
- Scalability: Interconnected systems can adapt when new components are added, ensuring scalability.
A deep understanding of interconnectivity involves exploring models and theories that support how connected systems operate. Two commonly referred models include the Open Systems Interconnection (OSI) model and the Internet protocol suite. These models provide frameworks for understanding the interactions within interconnected networks. Engineers often rely on these to design, develop, and manage systems efficiently.
Interconnectivity vs Interconnection
It is vital to distinguish between interconnectivity and interconnection. Although these concepts are related, they have distinct meanings. Interconnection often refers to the actual links established between systems or components to allow communication or data exchange. In contrast, interconnectivity refers to the quality or extent of connections and the achieved resultant synergy of a system working as a collective whole.
Think of interconnection as the physical wires and protocols connecting devices, while interconnectivity is the overall network functionality they enable.
In a data center, cables, and switches provide interconnections between servers. Interconnectivity is illustrated in the seamless processing and efficient data handling capabilities across the whole center, ensuring optimal performance.
Interconnectivity Examples in Audio Engineering
In the realm of audio engineering, interconnectivity is paramount. It involves linking diverse audio components to create cohesive sound systems that deliver optimal performance.
Real-world Interconnectivity Examples
Real-world examples of interconnectivity in audio engineering highlight how various components work in harmony. Consider professional recording studios:
- Microphones: These capture sound and send signals to mixing consoles.
- Mixing Consoles: They manage input from multiple sources, allowing sound adjustments before output.
- Speakers: They receive optimized audio from consoles, providing sound output.
In a live concert setting, a mixing engineer manages audio systems to ensure seamless sound delivery. The interconnection between microphones, mixing boards, and amplifiers facilitates real-time audio quality adjustments for performers.
A deeper exploration into audio interconnectivity uncovers protocols like AES/EBU and S/PDIF, which define standard digital audio connections. These formats ensure consistent and high-fidelity sound transmission across interconnected systems.
Audio Systems and Interconnectivity
In audio systems, interconnectivity ensures precise and effective sound management. Connectivity is crucial for components such as:
- Amplifiers: Provide power to drive speakers, interconnected via audio cables.
- Signal Processors: Modify audio signals to refine sound quality before routing to output devices.
- Monitors: Used by sound engineers for critical listening and mixing, interconnected within the entire audio chain.
Balanced cables help minimize noise in interconnected audio systems, using opposing signal phases to cancel out interference.
Peripheral Component Interconnect in Audio
Peripheral Component Interconnect (PCI) plays a role in audio by facilitating expansion in computer-based audio systems.
PCI is a hardware bus used to connect peripheral devices to a computer's motherboard, enhancing interconnectivity for audio applications.
In audio engineering, PCI sound cards allow for improved audio processing and connectivity beyond the motherboard’s basic capabilities. Features include:
- Multiple I/O ports: More input and output options increase connectivity flexibility.
- High-quality audio conversion: Sound cards offer better digital-to-analog and analog-to-digital conversion for superior audio quality.
An example of PCI implementation is the addition of a dedicated sound card in a desktop setup used for amateur music production. The card allows the user to connect keyboards, guitars, and microphones directly to the computer, establishing advanced interconnectivity within the audio workstation.
Open Systems Interconnection Model
The Open Systems Interconnection (OSI) Model is an essential concept in the world of digital communications. It provides a framework to understand how different networking protocols interact and function together within interconnected systems.
Basics of Open Systems Interconnection Model
The Open Systems Interconnection Model is a conceptual framework used to describe the functions of a networking system. It divides networking functions into seven distinct layers, each with specific responsibilities.
Understanding the OSI Model is foundational for studying network systems as it organizes communication functions into seven layers. Each layer serves a distinct role:
- Physical Layer: Concerned with the transmission of raw bit streams over a physical medium.
- Data Link Layer: Responsible for node-to-node data transfer and error detection/correction.
- Network Layer: Manages data addressing and routing across networks.
- Transport Layer: Ensures complete data transfer and reliability.
- Session Layer: Manages sessions and controls dialogues between computers.
- Presentation Layer: Translates data between the application and network formats.
- Application Layer: Provides network services to end-user applications, ensuring interoperability.
When you send an email, the Application Layer ensures it is in the correct format, while the Network Layer directs it to the right recipient. The Transport Layer confirms it reaches the destination reliably.
Think of the OSI Model as a blueprint that guides the design of network communication across different technologies.
Delving deeper into the OSI Model, it's essential to understand how the layers interact. Each receives data from the layer above, processes it, and transmits it to the layer below. This modular design allows individual layers to evolve without necessitating changes in others. The independence of layers facilitates robust innovation and the incorporation of emerging tech advancements. It also ensures devices and programs can communicate effectively, irrespective of their internal architectures.
Open Systems Interconnection Model and Audio Engineering
In audio engineering, the OSI Model plays a significant role in ensuring smooth and efficient data transmission over networks. By utilizing the OSI framework, audio engineers can effectively manage digital audio data as it travels across interconnected systems.
The OSI Model aids audio engineers by:
- Standardizing Protocols: Facilitates compatibility across different devices and software.
- Error Detection and Correction: Ensures the integrity of audio files transmitted over networks.
- Managing Audio Formats: Translates audio into suitable formats for transmission and playback.
During a live broadcast, the OSI Model ensures that audio captured at the venue is transmitted accurately and clearly to remote listeners, enabling both real-time and on-demand access to quality sound.
Interconnectivity in Modern Audio Systems
Interconnectivity is a foundational element in modern audio systems, ensuring that a variety of audio components work together seamlessly. From home audio setups to professional sound systems, understanding how interconnectivity operates can enhance sound quality and system efficiency.
Importance of Interconnectivity in Audio Technology
Audio technology relies on interconnectivity to create an integrated experience where different components function as a cohesive unit. This integration is particularly important for:
- Signal Transmission: Ensures effective communication between devices such as amplifiers, speakers, and mixers.
- Synchronization: Crucial for aligning audio data streams, whether in live performance or recording.
- Flexibility: Allows for scalable systems, easily adding or removing components as needed.
An intricate aspect of interconnectivity in audio technology involves understanding impedance matching. This principle is crucial for maximizing power transfer and minimizing reflections in audio signals. When the impedance of the source equals the load impedance, the transfer is most efficient. This is represented mathematically as: \( Z_{source} = Z_{load} \). Proper impedance matching improves audio fidelity and reduces noise interference in systems.
Consider a home theater system where the interconnectivity allows a single remote to control the audio receiver, speakers, and DVD player. This seamless operation is possible through interconnected controls and data protocols.
Optical cables offer high-fidelity audio transmission, providing an excellent choice for minimizing data loss in interconnected audio systems.
Future Trends in Audio Engineering and Interconnectivity
The future of audio engineering is closely tied to advancements in interconnectivity. As wireless technology evolves, it has begun to redefine how audio systems are interconnected.
Wireless Interconnectivity is the integration of components without physical cables, relying on technologies like Bluetooth and Wi-Fi to transmit audio signals.
Emerging trends in this field include:
- Smart Devices: Integration of voice-activated assistants with audio systems for hands-free operation.
- Multi-room Audio: Control of audio playback across several rooms using a centralized device.
- High-Resolution Streaming: Streaming lossless audio quality wirelessly using advanced codecs.
A promising dimension in the future of audio interconnectivity is the development of 3D spatial audio technologies. This involves creating an immersive audio environment where sound appears to emanate from any point in space. Achieving this requires precise synchronization across multiple interconnected speakers or headphones. The formula for calculating the necessary delay to achieve accurate sound localization using interaural time difference (ITD) can be expressed as: \( \text{ITD} = \frac{d}{c} \), where \( d \) is the distance between the ears, and \( c \) is the speed of sound. This enhancement will greatly enrich user experiences in virtual reality and augmented reality applications.
Keep an eye on advancements in low-latency Bluetooth technology, which promises to reduce delay issues in wireless audio transmission.
interconnectivity - Key takeaways
- Interconnectivity Definition: The state or quality of being connected together, linking various systems, networks, or components to work as a unified entity.
- Interconnectivity Examples: Smart home systems, audio engineering setups like recording studios, and computer networks where devices share resources and information efficiently.
- Open Systems Interconnection (OSI) Model: A conceptual framework with seven layers that describe the functions of a networking system, essential for understanding interactions within interconnected systems.
- Interconnectivity vs. Interconnection: While interconnection refers to physical links allowing communication, interconnectivity encompasses the quality and synergy achieved by a system working collectively.
- Peripheral Component Interconnect (PCI): A hardware bus that connects peripheral devices to a computer's motherboard, enhancing audio systems by allowing advanced connectivity and processing.
- Interconnectivity Meaning: Beyond device linking, it involves the coordination and interaction of connected elements, enabling scalability, resilience, and complex functionalities.
Learn faster with the 12 flashcards about interconnectivity
Sign up for free to gain access to all our flashcards.
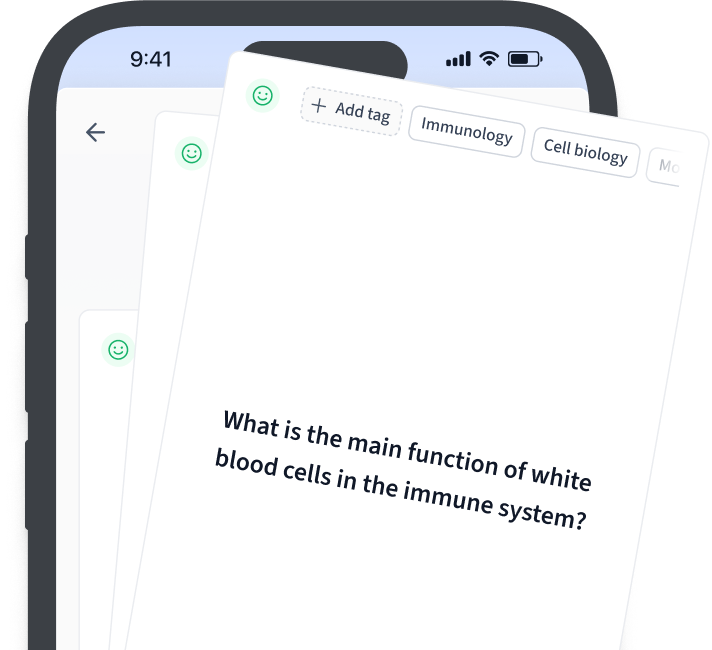
Frequently Asked Questions about interconnectivity
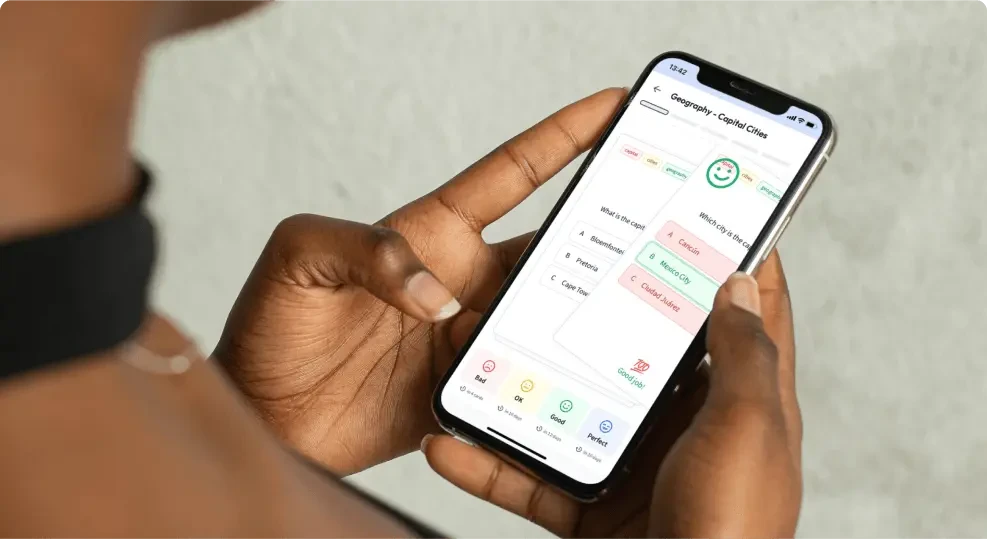
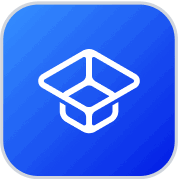
About StudySmarter
StudySmarter is a globally recognized educational technology company, offering a holistic learning platform designed for students of all ages and educational levels. Our platform provides learning support for a wide range of subjects, including STEM, Social Sciences, and Languages and also helps students to successfully master various tests and exams worldwide, such as GCSE, A Level, SAT, ACT, Abitur, and more. We offer an extensive library of learning materials, including interactive flashcards, comprehensive textbook solutions, and detailed explanations. The cutting-edge technology and tools we provide help students create their own learning materials. StudySmarter’s content is not only expert-verified but also regularly updated to ensure accuracy and relevance.
Learn more