Jump to a key chapter
Lateralization Definition in Engineering
In the field of engineering, lateralization refers to the process of assigning specific functions or responsibilities to different parts or components within a system. Understanding this concept is crucial for the effective design and implementation of complex systems, where it is important to optimize functionality and efficiency.
Lateralization Meaning in Engineering
Lateralization in engineering involves the distribution of tasks or functionalities across various sections or components within a system. It is particularly relevant in systems engineering, robotics, and software design. Essentially, lateralization helps in enhancing the performance and reliability of an engineering system by ensuring that each component or section of the system is specialized to perform a specific role. One common example in engineering is the implementation of distributed control systems (DCS) in industrial settings. Here, control functions are spread across multiple controllers rather than relying on a single centralized system. This approach increases system resilience and redundancy, reducing the impact of a failure in any single component.Another area where lateralization is crucial is in the development of multi-core processors. Each core in a multi-core processor can handle different tasks simultaneously, optimizing the overall processing speed and efficiency. This is particularly beneficial in high-performance computing where parallel processing is paramount.Lateralization can also be observed in the world of robotics. Robots with multiple limbs often distribute tasks among different limbs for better balance, speed, and efficiency. This distribution is an engineering application of lateralization where each limb is optimized for specific movements and tasks.Some of the benefits of lateralization in engineering include:
- Increased efficiency as tasks are divided according to the strengths of each part.
- Improved redundancy, offering better fault tolerance.
- Enhanced scalability, as systems can be expanded by adding more specialized components.
Lateralization Techniques in Engineering
Lateralization techniques are pivotal in engineering, enabling the division of labor among different components of a system to enhance performance. These techniques vary widely between traditional methods and more innovative approaches being developed.
Common Techniques
Common lateralization techniques in engineering often revolve around the distribution of control and processing tasks within a system. Some established techniques include:
- Distributed Control Systems (DCS): In DCS, control functions are spread across multiple controllers, aiding in task distribution and increasing system reliability. This method is frequently used in industrial automation.
- Use of Multi-Core Processors: Multi-core processors allocate different tasks to separate cores, allowing parallel processing and improving computational speed.
- Modular Design: Designing systems in modules, where each module handles specific tasks, allows for easier maintenance and increases flexibility.
Consider a solar energy system that uses lateralization. The system is divided into:
- Solar panels for energy collection.
- Inverters for converting DC to AC.
- Batteries for storing energy.
Remember, lateralization not only improves efficiency but also aids in achieving redundancy within systems, enhancing overall reliability.
Mathematical Representation of Lateralization: In the context of processing systems, consider the formulas like \[ T_{\text{total}} = \frac{N}{p} + T_{\text{com}} \]Where:
- N = Total number of tasks
- p = Number of processors
- T_{\text{com}} = Communication overhead
Innovative Approaches
New and innovative lateralization approaches leverage advanced technologies to further enhance system efficiency. Emerging techniques include:
- Machine Learning Models for dynamic task allocation, allowing systems to adapt and optimize resource utilization in real-time.
- Edge Computing: This approach involves performing data processing at the edge of the network closer to the source of data generations, reducing latency and the need for centralized data centers.
- Cyber-Physical Systems (CPS): CPS integrate computing and physical processes. Such systems optimize lateralization by providing real-time data and adapting automatically according to changing conditions.
In the context of Edge Computing, lateralization refers to strategically distributing data processing to multiple decentralized locations rather than a single centralized point. This reduces latency and improves processing efficiency.
Lateralization Applications in Engineering
Lateralization has a wide array of applications in engineering, significantly enhancing the functionality and efficiency of various systems by distributing responsibilities across different components.
Real-World Examples
In practical applications, lateralization shines across numerous engineering disciplines. These examples highlight its impact:Automobile Engineering: Modern cars utilize lateralization through distributed electronic control units (ECUs). Each ECU is responsible for specific vehicle functions, such as engine management, braking, or in-car entertainment. This division facilitates enhanced performance and easier troubleshooting.Telecommunications: Lateralization is evident in cellular networks, where tasks are divided among numerous base stations. Each station handles coverage for a specific area, ensuring seamless communication and service availability. Software Development: In software engineering, lateralization occurs via microservices architecture. Instead of a monolithic system, applications are broken into smaller, independent services, each handling specific tasks and easily scalable. These examples prove lateralization's invaluable role in refining efficiency and adaptation across diverse engineering fields.
In the realm of space exploration, lateralization is utilized extensively within the architecture of space probes. The systems of a space probe are distributed among different modules, each designed to perform distinct tasks. For example, the communication module maintains contact with Earth, while the scientific instruments module collects data. This division not only optimizes probe performance but also ensures that if one module fails, others can continue operating. Moreover, the International Space Station (ISS) provides a tangible example of lateralization in action. The various nations contributing to the ISS each assume responsibility for different modules and systems, enabling specialization and efficient management. This global collaboration exemplifies the principles of lateralization on an international scale.
Emerging Fields
Emergent fields are embracing lateralization to push the boundaries of innovation. Consider these areas:Artificial Intelligence (AI) Integration:- AI systems are becoming increasingly distributed across networks, enabling rapid data processing and decision-making near the source of data generation. For instance, edge AI leverages local devices' processing power to reduce latency and increase real-time analysis capabilities.Internet of Things (IoT):- In IoT systems, every device performs specific tasks, contributing to the overall network's functionality. This lateralization in role distribution enhances system efficiency and promotes seamless integration of heterogeneous devices.Blockchain Technology:- In blockchain, lateralization appears through decentralized nodes that validate transactions and maintain the integrity of the distributed ledger, ensuring security and operational efficiency.In these cutting-edge domains, lateralization is redefining possibilities and leading to groundbreaking developments.
An excellent example of lateralization in emerging fields is the development of smart grids. Smart grids use lateralized components to manage and distribute electricity more efficiently. Sensors, smart meters, and control devices each perform specific roles in monitoring and adjusting energy distribution in real-time. This results in reduced energy waste and increased grid reliability.
Lateralization Impact in Engineering
The impact of lateralization in engineering is profound, significantly influencing innovation and shaping future advancements. Understanding its effects allows engineers to leverage its benefits across various applications.
Influence on Innovation
Lateralization plays a crucial role in driving innovation within the engineering sector. By facilitating the distribution of tasks and responsibilities, it leads to the development of more efficient and cutting-edge technologies.One area where lateralization fosters innovation is in the realm of smart manufacturing. In this context, it allows for the implementation of decentralized manufacturing processes, where different parts of a system can independently handle specific production tasks.Let's consider a robotic assembly line with multiple robots, each designed to perform a specific operation such as welding, painting, or assembling. By distributing these tasks, lateralization reduces the operational complexity of each robot and enhances the overall efficiency of the production process. Each robot operates as a specialized unit, contributing to a smoother and more efficient assembly line.
- Lateralization encourages specialized development, allowing engineers to focus on modular innovations.
- It expands collaborative opportunities by enabling different teams to work on distinct components of a larger solution.
- Fosters quick adaptation to new technologies, as lateralized systems can be modified swiftly.
In the automotive industry, lateralization's influence on innovation is evident in the emergence of autonomous vehicles. These vehicles rely on distributed systems for functions such as navigation, collision detection, and communication with traffic systems. By compartmentalizing these tasks, manufacturers can innovate in each area independently, leading to rapid advancements and improvements in autonomous technology.
Innovations stemming from lateralization often result in improved scalability of engineering systems, allowing them to adapt to increasing demands seamlessly.
Mathematical Aspect of Lateralization:In engineering system design, lateralization can be quantitatively analyzed using equations such as:\[ E = \frac{\text{W}}{\text{N}} + C \ \ \]Where:
- E: Efficiency of the system
- W: Total work to be done
- N: Number of units or components
- C: Communication overhead among components
Challenges and Future Directions
While lateralization brings numerous benefits, it also introduces specific challenges that engineering fields must address. Understanding these challenges helps in paving effective future directions.One major challenge is coordination complexity. As systems become more distributed, ensuring seamless coordination among different components can be difficult. This complexity may lead to issues such as latency or inefficient communication, which can impact system performance.
- The challenge of ensuring security in lateralized systems is paramount, as decentralization often increases vulnerability to cyber threats.
- Interoperability poses another significant challenge, where integrating new technologies with existing distributed components must be handled carefully.
An example of innovative directions in addressing lateralization challenges can be seen in the field of networked control systems. Here, new algorithms are being designed to ensure robust synchronization among distributed controllers, ensuring reliable system performance even under varying operating conditions.
Addressing the challenges of lateralization often requires interdisciplinary approaches, combining knowledge from computer science, electrical engineering, and cybersecurity.
lateralization - Key takeaways
- Lateralization Definition in Engineering: Refers to assigning specific functions or responsibilities to different components within a system for optimized design and implementation.
- Lateralization Techniques in Engineering: Include distributed control systems, multi-core processors, and modular design to enhance system performance by division of labor.
- Lateralization Applications in Engineering: Can be seen in automotive engineering, telecommunications, and software via microservices architecture, promoting specialization and scalability.
- Lateralization Impact in Engineering: Drives innovation by facilitating task distribution, leading to more efficient technologies like smart manufacturing and autonomous vehicles.
- Innovative Lateralization Approaches: Utilize machine learning, edge computing, and cyber-physical systems for dynamic task allocation and enhanced system efficiency.
- Challenges of Lateralization: Include coordination complexity and security concerns, addressed through advanced synchronization techniques and blockchain technology.
Learn faster with the 11 flashcards about lateralization
Sign up for free to gain access to all our flashcards.
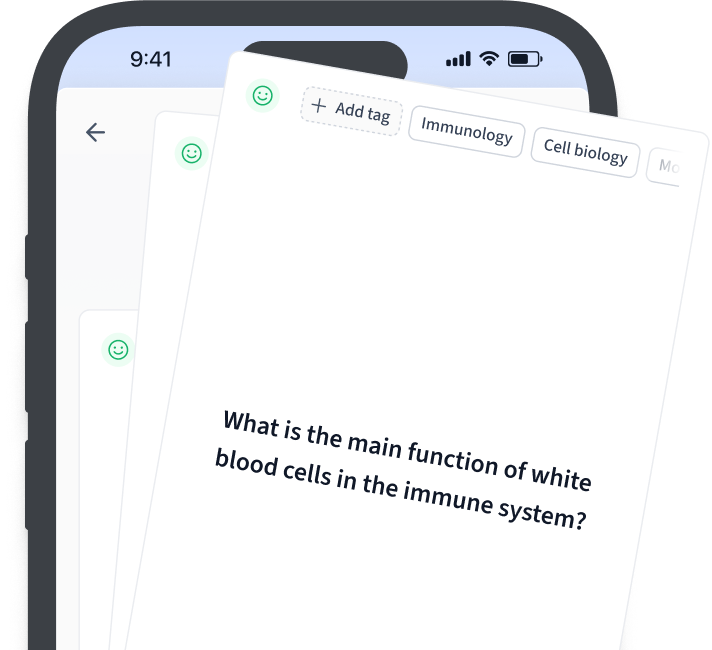
Frequently Asked Questions about lateralization
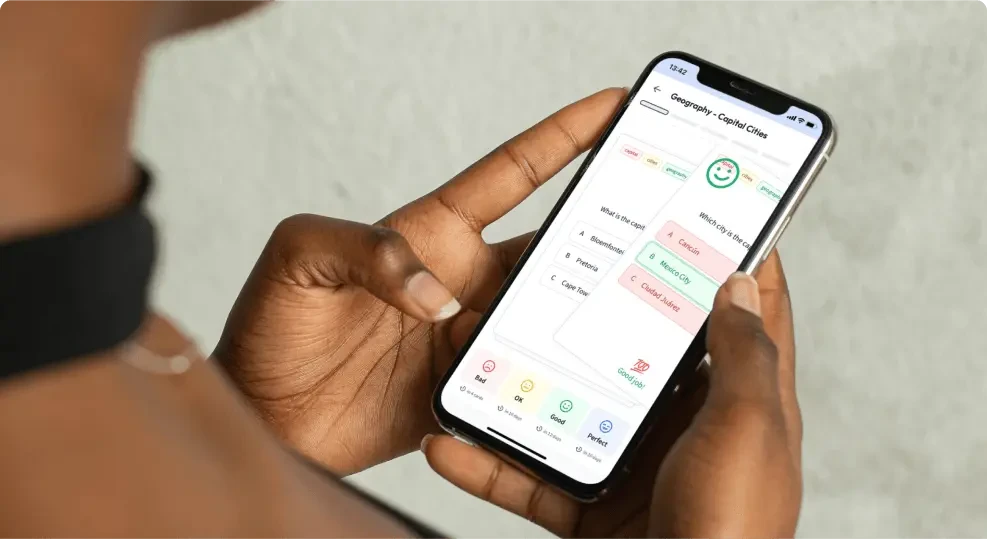
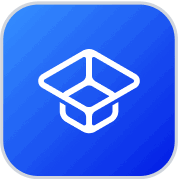
About StudySmarter
StudySmarter is a globally recognized educational technology company, offering a holistic learning platform designed for students of all ages and educational levels. Our platform provides learning support for a wide range of subjects, including STEM, Social Sciences, and Languages and also helps students to successfully master various tests and exams worldwide, such as GCSE, A Level, SAT, ACT, Abitur, and more. We offer an extensive library of learning materials, including interactive flashcards, comprehensive textbook solutions, and detailed explanations. The cutting-edge technology and tools we provide help students create their own learning materials. StudySmarter’s content is not only expert-verified but also regularly updated to ensure accuracy and relevance.
Learn more