Jump to a key chapter
Crankshaft Dynamics Explained
Understanding crankshaft dynamics is crucial for those interested in mechanical engineering and automotive design. The motion and behavior of a crankshaft significantly influence engine performance and efficiency. This section will explore fundamental concepts of crankshaft dynamics, providing key insights into the subject.
Basic Concepts of Crankshaft Dynamics
A crankshaft is a mechanical component that converts the linear motion of pistons into rotational motion. This is achieved through a complex system of cranks and bearings that synchronize the motion. The dynamics involved in this process are intricate, affected by numerous factors including engine speed, load, and vibration.
Crankshaft dynamics refer to the study and analysis of the forces and motions acting upon the crankshaft in an engine system. The main factors influencing these dynamics include torque, balance, and harmonic vibrations.
Each of these factors plays a significant role in how efficient and durable an engine can be:
- Torque: The amount of rotational force applied to the crankshaft.
- Balance: Ensures smooth operation by maintaining uniform motion.
- Harmonic Vibrations: Oscillations that can lead to mechanical failures if not controlled.
Torque and Crankshaft Dynamics
Torque is critical in crankshaft dynamics as it determines how effectively the engine's power is transmitted. The torque on a crankshaft can be calculated using the formula: \[ T = F \times r \] where T is the torque, F is the force applied, and r is the radius at which the force acts. Increased torque leads to higher engine power but also influences the stress on the crankshaft.
Consider an engine where the force applied by the pistons is 500 Newtons, and the radius of the crankshaft is 0.15 meters. The torque would be:\[ T = 500 \times 0.15 = 75 \text{ Nm} \]
Remember that torque impacts the mechanical advantage, which is crucial in engines for improving power output.
In more advanced studies, crankshaft torque serves as a critical parameter in evaluating an engine's performance under different conditions. Engineers often involve detailed simulations to predict torque characteristics across a range of operating speeds. Such simulations require considering factors such as frictional losses and transient loads. Additionally, the role of material properties in torque transfer is another interesting aspect. Advanced materials can provide better torque resilience, reducing wear and tear over time.
Balancing the Crankshaft
Crankshaft Torsional Dynamics
In the realm of mechanical engineering, crankshaft torsional dynamics is a pivotal study area. These dynamics involve understanding how torsional vibrations affect the crankshaft's operation and longevity. By analyzing torsional forces, engineers can design more efficient and reliable engines.
Understanding Torsional Vibrations
Torsional vibrations are oscillations that occur due to twists along the crankshaft's axis. These vibrations arise from fluctuations in torque caused by the uneven firing of engine cylinders. It is crucial to gauge these vibrations accurately as they can lead to mechanical wear and potential failure.
Torsional vibrations are the oscillatory movements in an engine's crankshaft, often characterized by angular displacements due to alternating forces during operation.
Regular monitoring and addressing of torsional vibrations are critical because:
- They can cause fatigue failure over time.
- They impact the smooth functioning of the engine.
- Mitigating them extends engine lifespan.
For instance, suppose an engine's crankshaft experiences a torsional vibration with an amplitude of 0.05 radians at a frequency of 100 Hz. The resulting angular velocity change can be computed using:\[ \theta(t) = A \times \text{sin}(2\text{π}ft) \]where A is the amplitude and f is the frequency.
Understanding vibration frequencies can lead to better design and balancing of crankshafts.
In-depth studies on torsional vibrations often involve utilizing damping mechanisms or dynamic dampers within the engine systems. These designs incorporate mechanisms that absorb or counteract the oscillatory motion, thereby reducing the amplitude of vibrations. Moreover, finite element analysis (FEA) is a powerful mathematical tool used to simulate and predict torsional behaviors in engines, offering insights into potential failures before they occur.
Causes of Torsional Vibrations
Several factors lead to torsional vibrations in a crankshaft. The most prevalent causes include:
- Engine Imbalances: Uneven weight distribution can initiate vibrations.
- Ignition Timing Issues: Incorrect spark timings disrupt the rotational balance.
- Material Defects: Imperfections in the crankshaft material may exacerbate oscillations.
- Loading Conditions: Variable loads during operation can generate undesirable vibrations.
Consider an engine experiencing issues due to loading conditions. If load varies between 300 Nm and 500 Nm, the fluctuation in torque is:\[ \tau = \tau_{max} - \tau_{min} = 500 - 300 = 200 \text{ Nm} \]Such variations can contribute to increased vibrational stresses.
Material choice and precision in manufacturing are essential for minimizing defects and imbalances.
Crankshaft Dynamic Analysis
The study of crankshaft dynamics provides crucial insights into engine design and performance. By examining how crankshafts respond to various forces and motions, engineers can enhance the reliability and efficiency of engines. This section explores the methods and tools used for effective crankshaft dynamic analysis.
Methods of Analysis
Several approaches exist for analyzing the dynamics of crankshafts, each offering unique perspectives and insights. These methods are essential for understanding and mitigating issues such as vibrations and torque anomalies.Common methods include:
- Finite Element Analysis (FEA): A numerical method that predicts how the crankshaft will react to forces, vibrations, heat, and other physical effects. It divides the crankshaft into discrete elements for detailed evaluation.
- Modal Analysis: Used to determine the natural frequencies and mode shapes of the crankshaft. Understanding these frequencies helps in addressing resonance issues.
- Vibration Analysis: Focuses on measuring and analyzing the oscillations within the crankshaft to identify sources of excessive vibrations.
Finite Element Analysis (FEA) is a computational technique used to simulate how physical systems react to external forces. It helps in identifying potential points of failure and areas requiring reinforcement.
Consider a crankshaft subject to a constant load of 1000 N and evaluated using FEA. The displacement due to the force can be computed using the formula for deflection:\[ \delta = \frac{F \times L^3}{3EI} \]where F is the force, L is the length, E is the modulus of elasticity, and I is the moment of inertia.
Modal analysis is crucial in avoiding resonance, which occurs when the engine operates at a natural frequency of the crankshaft.
In-depth modal analysis not only discovers the natural frequencies but also provides insight into the various mode shapes of crankshaft motion, such as bending, torsional, and axial modes. Precise mode shapes help in redesigning components for improved damping and reduced stress concentration. Advanced modal analysis often employs multi-degree-of-freedom (MDOF) models to simulate the complex interactions in engine assemblies, providing an accurate depiction of dynamic behavior. These insights enable engineers to develop crankshafts that offer optimal performance across a broad range of operational scenarios.
Tools Used in Crankshaft Analysis
The dynamic analysis of crankshafts employs a variety of tools, each selected for its ability to simulate and examine different aspects of crankshaft behavior. These tools allow for modeling, testing, and validation of designs before physical prototypes are created.Popular tools include:
- ANSYS: Widely used for FEA, allowing for detailed analysis of structural, thermal, and dynamic properties.
- MATLAB: Used for mathematical modeling, simulation and data analysis. It helps in solving complex differential equations associated with dynamic systems.
- ADAMS: Known for multibody dynamics, offering extensive capabilities to simulate the motion of linked bodies.
When dealing with a crankshaft subjected to torsional loads, one might use MATLAB to solve the governing differential equation:\[ J \frac{d^2 \theta}{dt^2} + C \frac{d\theta}{dt} + K\theta = 0 \]where J is the polar moment of inertia, C is the damping coefficient, and K is the torsional stiffness. MATLAB can provide numerical solutions for \(\theta(t)\).
ADAMS can simulate the impact of dynamic forces, helping to refine the balance of components such as counterweights and dampers.
Crankshaft Vibration Analysis
Conducting a thorough crankshaft vibration analysis is imperative in engine and mechanical systems. Vibrations arise from rotational dynamics and can significantly affect the performance and longevity of engines. By analyzing these vibrations, you can enhance the reliability and efficiency of the engine system.
Importance of Vibration Analysis
Vibration analysis in crankshafts is essential for several reasons. It helps identify potential issues before they escalate into major problems. This process can:
- Predict potential mechanical failures by identifying excessive vibration levels.
- Reduce maintenance costs by targeting specific areas for inspection and repair.
- Improve engine efficiency by optimizing component alignment and balance.
Vibration analysis involves the measurement and interpretation of oscillatory motion caused by internal and external forces impacting the crankshaft.
During a routine inspection, a vibration analysis identified a frequency vibration at 120 Hz with an amplitude of 0.02 mm in the crankshaft of an engine. The analysis suggested realigning the crankshaft to mitigate this resonance and prevent damage.
Vibration analysis often employs advanced techniques such as Fast Fourier Transform (FFT) to decompose vibration signals into sine waves of various frequencies. This detailed breakdown allows for pinpointing the root causes of abnormalities. Moreover, coupling these insights with machine learning algorithms could provide predictive maintenance solutions, automatically identifying potential failures before they occur, based on historical vibration data patterns and current measurements.
A well-executed vibration analysis can significantly prolong the lifespan of the engine and enhance its operational efficiency.
Techniques for Reducing Vibrations
Reducing crankshaft vibrations requires targeted interventions. Effective techniques include:
- Dynamic Balancing: Ensuring the mass distribution around the crankshaft axis is uniform minimizes vibrations.
- Dampers: Installation of dampers absorbs and dissipates vibrational energy.
- Tuning: Adjusting engine operational parameters to avoid natural frequencies can reduce resonance.
In a case where crankshaft vibrations were causing bearing failures, implementing dynamic balancing by adding counterweights dramatically reduced vibration levels, thereby preventing further damage.
Dynamic balancing not only involves physical modifications but also requires computational simulations to model mass distribution. Using simulation tools, engineers can predict the impact of changes before physically altering the components. Innovations in material technology have also introduced composite dampers, which provide effective vibration reduction while being lightweight.
Regular assessment and fine-tuning can keep vibrations in check, leading to optimal engine performance.
Dynamic Load and Stress Analysis of a Crankshaft
In engineering, understanding the dynamic load and stress on a crankshaft is crucial for optimizing its design and performance. This involves analyzing how different forces affect the mechanical integrity of the crankshaft over time. Such analysis helps in predicting failures and designing for longevity.
Evaluating Loads and Stresses
The process of evaluating loads and stresses on a crankshaft requires a detailed understanding of the forces acting upon it. These forces are often dynamic, changing with engine speed and operating conditions. Main factors considered in the evaluation include:
- Axial Loads: Forces along the crankshaft's axis.
- Radial Loads: Forces perpendicular to the crankshaft axis.
- Torsional Stress: Twisting forces due to rotational motion.
Dynamic Load refers to the varying forces exerted on a mechanical component due to motion, which can cause fluctuating stresses in structures like crankshafts.
For example, if a crankshaft experiences an axial force of 5000 N and the cross-sectional area is 0.02 m², the axial stress can be calculated as:\[ \sigma = \frac{5000}{0.02} = 250,000 \text{N/m}^2 \]This calculation helps in determining whether the material can withstand the generated stress.
Comprehensively understanding load paths can significantly aid in efficient crankshaft design.
Advanced studies in stress analysis often incorporate finite element methods to simulate how crankshafts respond under various loading conditions. These simulations can provide detailed insights into stress distribution and the identification of potential stress concentrators, which are regions where stress is significantly higher than average. By applying fatigue analysis, engineers can predict the lifespan of materials under cyclic loading, which is paramount in preventing unexpected failures. Furthermore, ensuring that materials used for crankshafts have a high fatigue limit can help in enhancing durability. Innovations such as using composite materials, which offer superior fatigue resistance, have been increasingly integrated into modern designs.
Impact of Load Variations
Load variations can significantly affect the dynamic behavior of crankshafts. These variations often occur due to changes in engine load, speed, or operating environments. The consequences of such variations include increased wear and risk of failure.Key factors influencing load variations include:
- Engine RPMs: Fluctuating revolutions per minute alter dynamic forces.
- Temperature Changes: Variations in temperature affect material properties and stress.
- Material Inhomogeneity: Inconsistencies in materials can lead to uneven distribution of stresses.
Consider an engine experiencing load variations due to altitude changes, leading to reduced oxygen and varying combustion effectiveness. Such environmental factors alter the stress experienced by the crankshaft. Engineers must incorporate these loads in design simulations to ensure structural integrity is maintained under varying atmospheric pressures.
Considering environmental and operational factors during the design phase can dramatically reduce risk and enhance durability.
Further exploration into the impact of load variations reveals that factors such as environmental conditions, irregular combustion cycles, and mechanical faults can exacerbate wear patterns. Crankshafts subjected to these conditions require robust design optimizations, including precise alignment, rotational balancing, and the integration of advanced materials that can withstand fluctuating thermal and mechanical stresses. Employing load monitoring systems in engines can provide real-time data, alerting engineers to unexpected variations before they can cause irreversible damage. This proactive approach emphasizes the importance of continuous monitoring and adaptive systems in modern mechanical design.
Dynamic Crankshaft Balancing
In the field of automotive engineering, dynamic crankshaft balancing is fundamental for enhancing the efficiency and performance of engines. Proper balancing can significantly reduce vibrations, improve power output, and extend engine life. This section delves into the intricate process of achieving ideal crankshaft balance and its subsequent effects on engine performance.
The Process of Balancing
Dynamic balancing involves equalizing the mass distribution around the axis of rotation of a crankshaft. This procedure minimizes vibrational disturbances that can lead to excessive wear and mechanical failures. The primary steps in the process include:
- Identifying Imbalances: Initial step involves the detection of mass distribution irregularities using diagnostic tools.
- Correction: The application of precise counterweights or material removal to balance the crankshaft assembly.
- Verification: Testing the crankshaft after adjustments to ensure stability under operational conditions.
Dynamic balancing is the process of arranging the mass distribution of a rotating body so that it moves smoothly, without wobble or vibration.
Consider a crankshaft where a 10g imbalance is detected at a radius of 0.1m from the rotational axis. To achieve balance, a counterweight creating an equal and opposite motion is used. The correction can be calculated using:\[ F = m \cdot r \cdot \omega^2 \]where F is the force, m is the mass needed, r is the radius, and \omega is the angular velocity.
Balancing is critical not only for performance but also for preventing long-term structural damage to the engine components.
Dynamic crankshaft balancing also involves the use of high-precision instruments and software to simulate and predict vibrations across various operational conditions. Engineers use these simulations to validate the balancing procedure and ensure no new imbalances arise in practical operations.Successful balancing relies on:
- Accurate detection and measurement of imbalances
- Proper selection of corrective methods such as adding/removing materials
- Consistent verification through testing and analysis
In-depth studies on dynamic balancing techniques focus on the rotational dynamics and gyroscopic effects that are not immediately apparent. Engineers use complex algorithms to model these conditions, optimizing the balance under varying loads and speeds. High-speed balancing machines are calibrated to detect even minute imbalances by simulating actual operational speeds, ensuring the crankshaft performs optimally across all possible conditions. Innovations in balancing also include the use of advanced materials for counterweights, such as high-density alloys, which provide efficient mass distribution without significantly increasing the crankshaft's overall weight.
Effects on Engine Performance
Dynamic crankshaft balancing plays a crucial role in enhancing engine performance. Balanced engines exhibit:
- Reduced Vibrations: Less mechanical stress leads to smoother operation and reduced noise levels.
- Increased Efficiency: Energy losses due to vibrations are minimized, leading to better fuel efficiency and power output.
- Extended Lifespan: Lower mechanical stress translates into decreased wear and tear, extending the durability of engine components.
For instance, an engine previously experiencing high levels of vibration post-balancing demonstrated a 5% improvement in fuel consumption and a 10% reduction in maintenance frequency over a year. This improvement underscores the tangible performance benefits achieved through meticulous crankshaft balancing.
Regular monitoring and balancing check-ups are integral to maintaining engine efficiency and preventing unexpected failures.
The effect of balancing reaches beyond immediate performance metrics. In engines with dynamic stability, there is a notable reduction in sound emissions and a smoother ride quality, which are essential considerations for automotive manufacturers targeting high-end consumer satisfaction. By minimizing vibrations, balancing amplifies the engine's ability to maintain peak performance across extended periods, reducing pollutants and emissions and aligning with regulatory standards for greener technologies. This holistic improvement adds a competitive edge to automotive products in the marketplace, reflecting the essential nature of dynamic crankshaft balancing in modern engineering.
crankshaft dynamics - Key takeaways
- Crankshaft Dynamics: Study of forces and motions acting on the crankshaft in an engine system, crucial for engine performance and efficiency.
- Crankshaft Torsional Dynamics: Analysis of torsional vibrations affecting crankshaft operation and longevity.
- Crankshaft Dynamic Analysis: Techniques used to evaluate how crankshafts respond to various forces, including FEA and vibration analysis.
- Dynamic Load and Stress Analysis of a Crankshaft: Examination of how different forces affect crankshaft mechanical integrity, predicting failures and optimizing design.
- Dynamic Crankshaft Balancing: Process of adjusting mass distribution to reduce vibrations, improve efficiency, and extend engine life.
- Crankshaft Vibration Analysis: Measurement and interpretation of oscillatory motion in crankshafts to enhance reliability and efficiency.
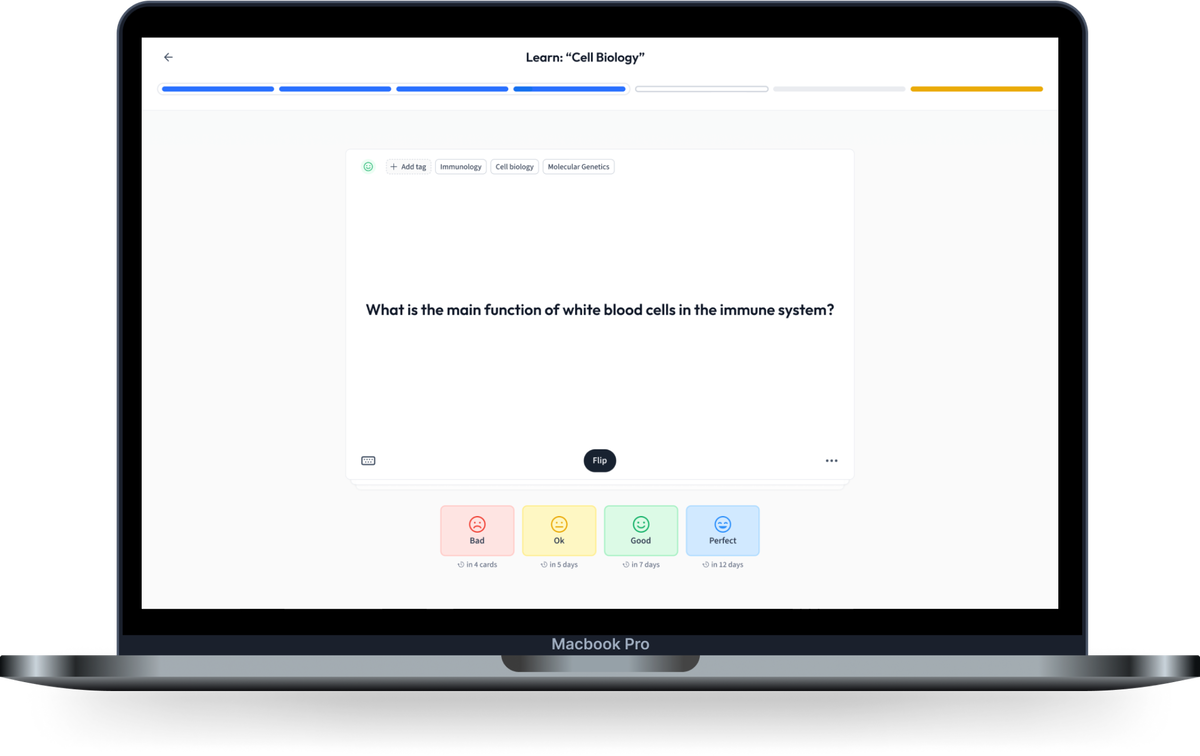
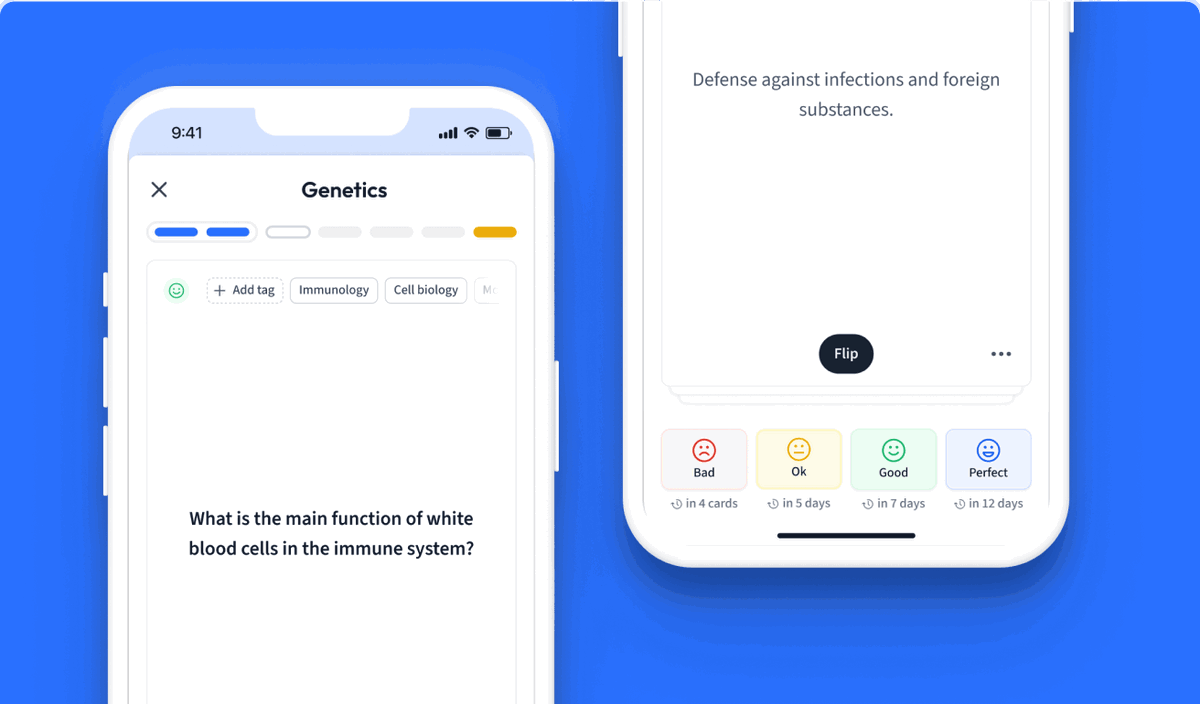
Learn with 24 crankshaft dynamics flashcards in the free StudySmarter app
Already have an account? Log in
Frequently Asked Questions about crankshaft dynamics
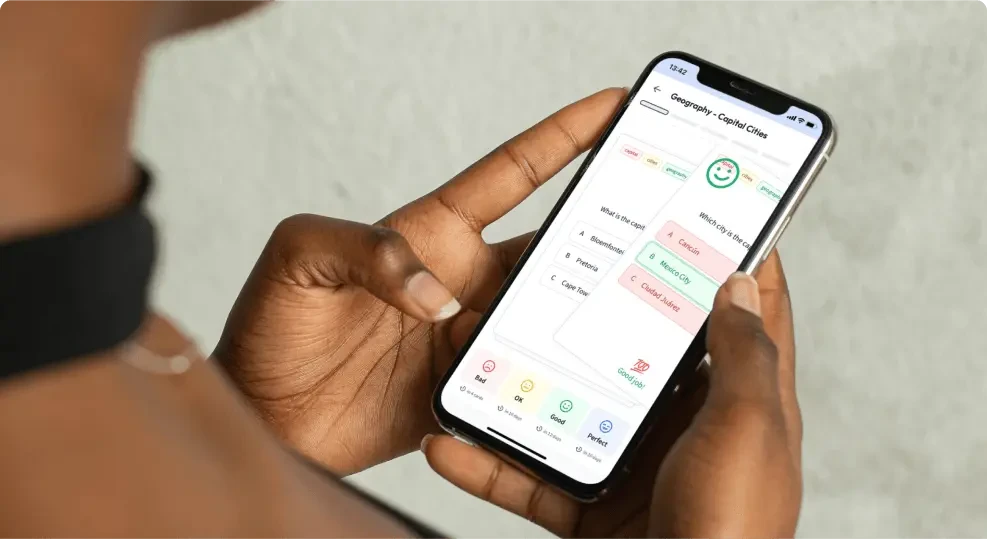
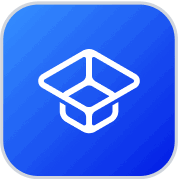
About StudySmarter
StudySmarter is a globally recognized educational technology company, offering a holistic learning platform designed for students of all ages and educational levels. Our platform provides learning support for a wide range of subjects, including STEM, Social Sciences, and Languages and also helps students to successfully master various tests and exams worldwide, such as GCSE, A Level, SAT, ACT, Abitur, and more. We offer an extensive library of learning materials, including interactive flashcards, comprehensive textbook solutions, and detailed explanations. The cutting-edge technology and tools we provide help students create their own learning materials. StudySmarter’s content is not only expert-verified but also regularly updated to ensure accuracy and relevance.
Learn more