Jump to a key chapter
Induction Motors Overview
Delve into the fascinating world of induction motors, a vital component in numerous applications due to their efficiency and reliability. In this overview, you'll explore key concepts and theories that form the foundation of these electric machines.
Induction Electric Motor Basics
Induction motors, also known as asynchronous motors, are incredibly prominent in both industrial and household applications. These motors operate on the principle of electromagnetic induction, where the electric current is induced in the rotor due to the magnetic field produced by the stator. They are known for their simplicity, durability, and low cost.
An induction motor is an electric motor in which the electric current in the rotor needed to produce torque is obtained via electromagnetic induction from the magnetic field of the stator winding.
The stator is the stationary part of the motor's electromagnetic circuit, while the rotor is the rotating part. The electricity supplied to the stator creates a magnetic field, which induces a current in the rotor. This phenomenon generates the force that rotates the rotor, thus performing work.
For example, in a three-phase induction motor, the magnetic field rotates around the stator at a speed known as the synchronous speed which can be calculated using the formula: \[ N_s = \frac{120f}{P} \]where,
- N_s is the synchronous speed in revolutions per minute (RPM).
- f is the frequency of the electric supply in hertz (Hz).
- P is the number of poles in the stator.
The torque production process in an induction motor can be better understood by studying the slip. Slip is defined as the difference between the synchronous speed, \( N_s \), and the actual rotor speed, \( N_r \). It can be expressed with the formula: \[ s = \frac{N_s - N_r}{N_s} \] where,
- s is the slip,
- N_r is the rotor speed in RPM.
Induction Motor Theory Explained
The theory behind induction motors involves several facets, starting with the basic principles of electromagnetism which govern their operation. At its core, an induction motor converts electrical energy into mechanical energy through the process of electromagnetic induction.
The interaction between the magnetic field generated by the stator and the induced currents in the rotor is what results in the motor's rotation. This interaction can be analyzed using the concept of the rotating magnetic field, which provides a comprehensive understanding of the motor's behavior under different load conditions.
The rotating magnetic field is a magnetic field whose magnitude is constant, but its direction continuously rotates around a fixed axis.
The operation of the induction motor can be characterized by its different types, including squirrel-cage and slip-ring motors. Both types work on the same principle but differ in construction and applications.
Consider a squirrel-cage motor, which is the most common type of induction motor. Its rotor resembles a hamster wheel or squirrel cage, and it is known for its ruggedness and reliability. These motors are ubiquitous in industrial settings due to their low cost and maintenance demands, making them ideal for heavy-duty applications.
In an induction motor, the rotor's construction is always a factor in determining its performance characteristics such as starting torque, efficiency, and speed regulation.
Induction Motor Working Principle
Discover the fundamentals of how induction motors operate, focusing on key elements like electromagnetic induction and the interplay between the rotor and stator. These principles are foundational to understanding the mechanics and applications of induction motors.
Electromagnetic Induction
At the heart of an induction motor is the principle of electromagnetic induction. This phenomenon was first discovered by Michael Faraday, and it forms the basis for many electrical machines and devices. When a conductor, such as a coil of wire, is placed in a changing magnetic field, an electromotive force (EMF) is induced in the conductor. This is mathematically represented by Faraday’s law of induction: \[ \text{EMF} = - N \frac{d\text{B}}{dt} \] where,
- EMF is the electromotive force in volts,
- N is the number of turns in the coil,
- B is the magnetic flux.
Imagine an induction motor used in a ceiling fan. The alternating current (AC) in the stator creates a rotating magnetic field. This rotating field induces a current in the rotor, efficiently causing it to spin and drive the fan blades.
Beyond Faraday’s law, electromagnetic induction in an induction motor also involves Lenz's Law. Lenz’s Law states that the direction of induced current in a conductor will be such that it opposes the change in magnetic field that produced it. This opposing force contributes to what is known as back EMF in the motor. Understanding this offers deeper insights into both the energy conversion efficiency and dynamic response of the motor under variable loads.
Role of Rotor and Stator
The stator and rotor are crucial components of an induction motor, each playing significant roles in its operation. The stator, comprising of iron cores and conductive windings, is fixed and acts as the source of the motor's magnetic field. It is connected to the AC supply and induces a magnetic field that radiates towards the rotor.
The stator is the stationary part of an induction motor, containing windings in which the magnetic field is generated by the supplied AC electrical energy.
The rotor, on the other hand, is the rotating part of the machine. It is placed inside or next to the stator field and through electromagnetic induction, carries the induced current necessary for generating motive force. The most common type of rotor used is the squirrel cage rotor.
Consider a three-phase induction motor. The stator creates a rotating magnetic field that moves at synchronized velocity with the supplied AC frequency. This behavior can be calculated by the equation for synchronous speed: \[ N_s = \frac{120f}{P} \] where,
- N_s is the synchronous speed in RPM,
- f is the frequency of the AC supply in Hz,
- P is the number of poles.
The efficiency of an induction motor can often be improved by minimizing energy losses in the stator and rotor windings, emphasizing the importance of quality materials and design.
Induction Motor Torque Equation
Understanding the torque equation of an induction motor is crucial for grasping its performance characteristics. It ties electrical parameters with mechanical output, providing insights into how variations in supply and motor design impact functionality.
Derivation of Torque Equation
The torque produced by an induction motor can be derived from electromagnetic principles and relates to the power interaction between the stator and rotor. Torque, denoted as \( T \), can be mathematically formulated using the following expression: \[ T = \frac{P_{air}}{\omega_s} \] where,
- \( T \) is the torque in Newton-meters (Nm),
- \( P_{air} \) is the air-gap power in watts,
- \( \omega_s \) is the synchronous speed in radians per second (rad/s).
- \( V_{ph} \) is the phase voltage,
- \( I_{rph} \) is the rotor current per phase,
- \( \phi \) is the phase angle.
The torque equation is a mathematical representation of the torque generated by an induction motor, linking electrical input to mechanical output.
Consider a situation where a three-phase induction motor operates at a slip \( s \) of 0.04 with a synchronous speed \( N_s \) of 1500 RPM. The rotor current results in an air-gap power of 5 kW. The torque can be computed using the derived formula as:\[ \omega_s = \frac{2\pi N_s}{60} \]\[ T = \frac{5000}{\omega_s} \] Substitute the value of \( \omega_s \) to find \( T \).
The slip is intricately involved in the generation of torque and can deeply affect motor performance. The slip, \( s \), can be expressed as:\[ s = \frac{N_s - N_r}{N_s} \]where
- \( N_s \) is the synchronous speed,
- \( N_r \) is the actual rotor speed.
Factors Affecting Torque
Several factors influence the torque production in induction motors, determining their practical application and efficiency. These include motor design parameters and operational conditions.
Key factors affecting torque include:
- Supply Voltage: Higher voltage can increase torque up to a saturation point.
- Frequency: Changing the frequency alters the synchronous speed and thus affects torque.
- Rotor Resistance: Increased rotor resistance at start boosts starting torque but may diminish efficiency.
- Load Conditions: External load changes affect the motor’s slip and resultant torque.
A higher slip can be beneficial for applications requiring high starting torque, such as elevators and hoists. However, it comes at the cost of efficiency during normal operation.
Induction Motor Applications
Explore the diverse applications of induction motors in various fields. Known for their robustness and efficiency, these motors are integral to numerous devices and machinery, whether in household settings or industrial complexes.
Common Uses
Induction motors are prevalent in everyday applications due to their simplicity and reliability. They are widely used in:
- Household Appliances: Induction motors power common appliances like ceiling fans, washing machines, and refrigerators. Their ability to run quietly and with minimal maintenance makes them ideal for domestic settings.
- HVAC Systems: They play a crucial role in heating, ventilation, and air conditioning systems, providing the necessary mechanical work to circulate air effectively.
- Mini Electric Vehicles: Small-scale electric vehicles, such as scooters and bikes, often utilize induction motors for their efficiency and instant torque output.
Imagine a washing machine that uses an induction motor to rotate its drum. The motor's reliable and efficient performance ensures the drum spins at the desired speed, using the formula \[ N_r = N_s (1-s) \] where
- \( N_r \) is the rotor speed,
- \( N_s \) is the synchronous speed,
- \( s \) is the slip.
The efficiency of an induction motor in household appliances could be optimized further by adjusting slip and rotor resistance.
Industrial Applications
Induction motors serve as the backbone in various industrial environments due to their capacity to deliver high power output and their durability. Key industrial applications include:
- Pumping Systems: Induction motors are used extensively in water and oil pumps, demanding reliability and continual operation.
- Machine Tools: In manufacturing, tools like lathes and drills rely on induction motors for precise and powerful rotations.
- Conveyors: They are ideal for driving conveyor systems, especially in automated production lines, where consistent speed and torque are required.
A variable frequency drive (VFD) is a type of motor controller that drives an electric motor by varying the frequency and voltage supplied to the motor.
Of note in industrial use is the impact of the motor's power factor, which is a measure of how effectively the electrical power is being converted into useful work while minimizing losses. Power factor can be improved by tweaking design parameters like optimizing stator and rotor windings, which not only enhances efficiency but also saves on energy costs in large scale operations. Additionally, induction motors benefit industrial applications by providing reduction in wear and tear compared to other types due to the absence of brushes, thus lowering maintenance demands in tough working conditions.
Induction Motor Performance
The performance of induction motors is crucial, as it determines their suitability for various tasks and impacts operational costs. This section addresses important factors related to the efficiency and optimization of induction motor performance.
Efficiency Considerations
The efficiency of induction motors is a key performance metric, reflecting how well they convert electrical energy into mechanical energy. Higher efficiency results in reduced energy consumption and operational costs.Highlighting several elements that impact efficiency:
- Load Conditions: Efficiency varies with the load level. Motors typically operate at peak efficiency when running near their full-load capacity.
- Motor Design: The design of the motor, including aspects such as the quality of the stator and rotor materials, influences efficiency.
- Supply Voltage: Deviations in supply voltage can lead to inefficiencies, often causing an increase in losses.
- \( P_{\text{out}} \) is the output mechanical power.
- \( P_{\text{in}} \) is the input electrical power.
Keep motors well-maintained to avoid efficiency losses due to component wear or dirt accumulation which can impede performance.
A critical element impacting efficiency is the power factor. It is defined as the cosine of the angle \( \phi \) between the input voltage and current, thus:\[ \text{Power Factor} = \cos(\phi) \]Improving the power factor with capacitive elements or other techniques can enhance overall motor efficiency and reduce energy costs. Efficient motors not only save on electrical expenses but also mitigate heat buildup, prolonging motor life. Additionally, choosing high-efficiency motors during design stages, such as those meeting NEMA Premium energy efficiency standards, can provide long-term savings.
Performance Optimization Methods
To ensure optimal performance of induction motors, various strategies can be employed. These methods focus on enhancing output while minimizing energy waste and operational costs.Some key methods include:
- Proper Sizing: Selecting the motor size appropriate for the load helps maintain efficiency by avoiding under or oversizing.
- Variable Frequency Drives (VFDs): Implementing VFDs allows control over the motor speed, adjusting to match the operational demand.
- Regular Maintenance: Periodic inspections help identify inefficiencies such as misalignment or bearing issues which could degrade performance over time.
A Variable Frequency Drive (VFD) is a device that controls the speed of an electric motor by varying the frequency of the electrical power supplied.
Consider an industrial conveyor system that employs an induction motor. By incorporating a VFD, the motor speed can be aligned with the conveyor's load requirements, ensuring efficiency improvements. For instance, using the formula \[ N = \frac{120f}{P} \]where:
- \( N \) is the rotor speed in RPM,
- \( f \) is the frequency of the AC supply,
- \( P \) is the number of poles,
induction motors - Key takeaways
- Induction Motors: Electric machines relying on electromagnetic induction to generate torque, widely used for their simplicity, durability, and low cost.
- Induction Motor Working Principle: Operates on the principle of electromagnetic induction where the stator's magnetic field induces a current in the rotor, producing mechanical rotation.
- Induction Motor Torque Equation: Torque is calculated using the formula: \[ T = \frac{P_{air}}{\omega_s} \] where torque ties electrical inputs to mechanical outputs.
- Induction Motor Applications: Induction motors are used in household appliances like fans and washing machines, HVAC systems, and industrial applications such as pumps and conveyors.
- Induction Motor Theory: Involves electromagnetism principles and the interaction between stator's magnetic field and rotor's induced current for energy conversion.
- Induction Motor Performance: Performance is determined by motor efficiency, load conditions, and optimization methods like proper sizing and use of Variable Frequency Drives (VFDs).
Learn faster with the 20 flashcards about induction motors
Sign up for free to gain access to all our flashcards.
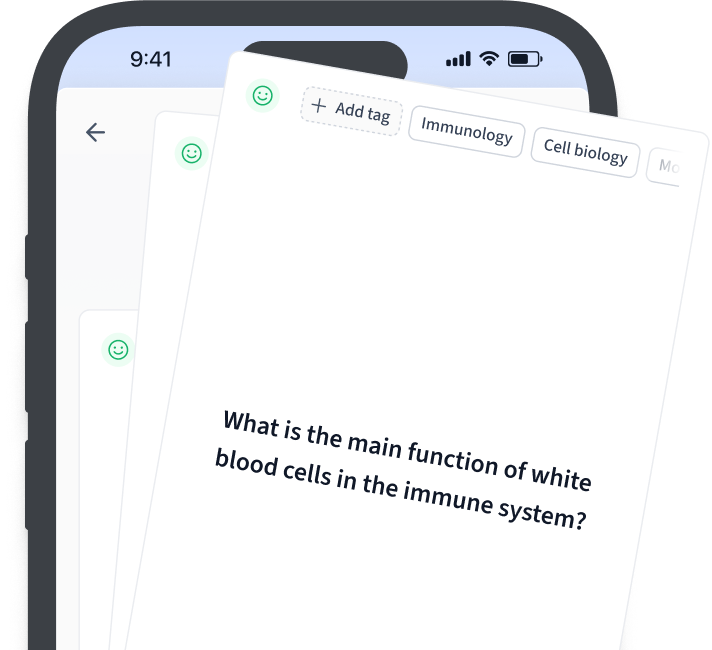
Frequently Asked Questions about induction motors
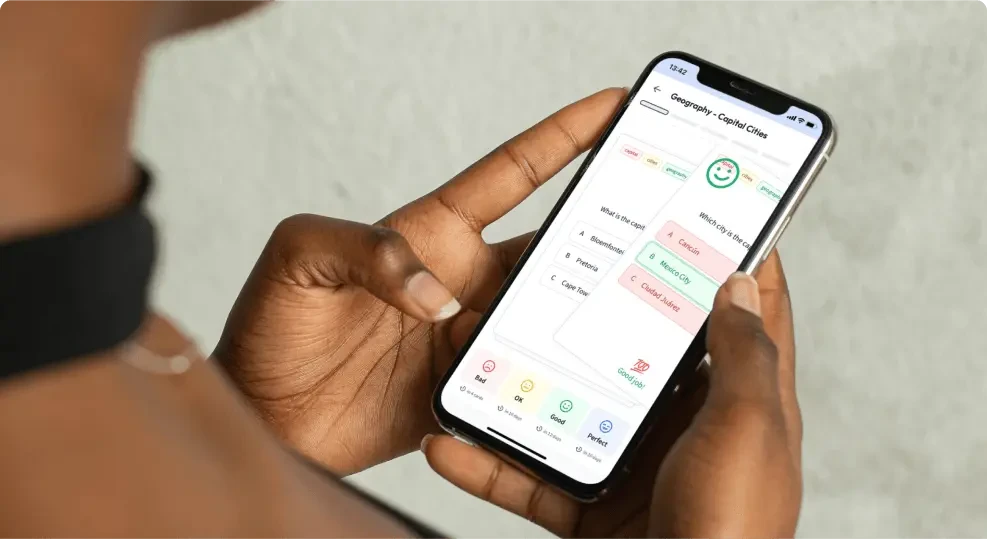
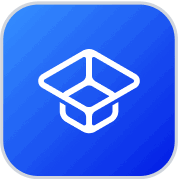
About StudySmarter
StudySmarter is a globally recognized educational technology company, offering a holistic learning platform designed for students of all ages and educational levels. Our platform provides learning support for a wide range of subjects, including STEM, Social Sciences, and Languages and also helps students to successfully master various tests and exams worldwide, such as GCSE, A Level, SAT, ACT, Abitur, and more. We offer an extensive library of learning materials, including interactive flashcards, comprehensive textbook solutions, and detailed explanations. The cutting-edge technology and tools we provide help students create their own learning materials. StudySmarter’s content is not only expert-verified but also regularly updated to ensure accuracy and relevance.
Learn more