Jump to a key chapter
Kinetic Energy Recovery Definition
Kinetic energy recovery is a process that involves capturing and storing energy generated during the motion of a vehicle, which would otherwise be wasted. This energy can later be reused to power the vehicle, improving efficiency and reducing energy consumption. Understanding how kinetic energy recovery systems (KERS) work is crucial to grasp the innovations in modern vehicle technology.
Kinetic Energy Recovery System (KERS): A system that recovers energy normally lost during braking and stores it for later use. It converts kinetic energy from the motion of the vehicle into electric or mechanical energy, which can be utilized to enhance vehicle performance or improve fuel efficiency.
Types of Kinetic Energy Recovery Systems
There are several types of Kinetic Energy Recovery Systems, each with unique mechanisms and applications. Here, we explore some of the common systems used in vehicles today:
- Flywheel Systems: Use a flywheel to store kinetic energy as rotational energy, which can be released to assist acceleration.
- Battery Systems: Convert kinetic energy into electrical energy stored in batteries, often employed in hybrid and electric vehicles.
- Hydraulic Systems: Use hydraulic fluid under pressure to capture and store energy, later used to power the vehicle hydraulically.
Consider a hybrid vehicle equipped with a battery-based KERS. When the vehicle brakes, kinetic energy is transformed into electrical energy, charging the vehicle's battery. Later, this energy assists in propulsion, reducing the workload on the internal combustion engine. Mathematically, if a vehicle of mass \(m\) travels at velocity \(v\), the kinetic energy \(E_k\) is reduced as:\[E_k = \frac{1}{2} m v^2\]This energy is what the KERS aims to recover and store.
Let's delve deeper into flywheel energy storage systems. Flywheels work on the principle of rotational inertia, where energy is stored in a rapidly spinning mass. The speed at which a flywheel spins is crucial as its energy storage capacity is proportional to the square of the rotation speed. The equation governing this relationship is:\[E = \frac{1}{2} I \omega^2\]where \(E\) is the energy stored, \(I\) is the moment of inertia, and \(\omega\) is the angular velocity. The effectiveness of flywheels comes from their ability to transfer energy rapidly, making them ideal for situations requiring quick power bursts. However, they require careful engineering to manage the high rotational speeds safely and efficiently.
KERS technology not only improves fuel efficiency but also reduces emissions, making it a key component in sustainable vehicle design.
Kinetic Energy Recovery System Overview
Kinetic energy recovery involves harnessing energy generated during motion that would typically be lost as heat. This energy is stored for later use, enhancing vehicle efficiency and performance. Systems implementing this technology are known as kinetic energy recovery systems (KERS), key to modern automotive innovation.
Types of Kinetic Energy Recovery Systems
Kinetic Energy Recovery Systems come in various forms, benefiting different types of vehicles. Let's explore a few common types:
- Flywheel Systems: These systems utilize a flywheel to store energy as rotational energy, releasing it later during acceleration.
- Battery Systems: Convert kinetic energy to electrical energy stored in batteries, mainly found in hybrid and electric vehicles.
- Hydraulic Systems: Employ hydraulic fluid to store energy under pressure for later vehicle propulsion.
Kinetic Energy Recovery System (KERS): Devices designed to recover energy typically lost during braking, storing it for future utilization. This energy conversion enhances the performance efficiency of vehicles.
Assume a hybrid car with a battery-based KERS. During braking, kinetic energy is transformed into electrical energy, charging the battery. Later, this stored energy assists in vehicle propulsion, reducing engine load. Mathematically, the kinetic energy \(E_k\) of a moving vehicle with mass \(m\) and velocity \(v\) is calculated as:\[E_k = \frac{1}{2} m v^2\]This represents the energy component that KERS aims to recapture and reuse.
Exploring flywheel systems deeper, these mechanisms store energy in a rapidly spinning mass based on rotational inertia. The flywheel's energy storage capacity is related to its rotation speed. The governing equation is:\[E = \frac{1}{2} I \omega^2\]where \(E\) represents stored energy, \(I\) is the moment of inertia, and \(\omega\) is angular velocity. Flywheels excel at quick energy transfers, beneficial for immediate power demands. However, managing high-speed rotations necessitates precision engineering for safety and efficiency.
The use of KERS technology reduces vehicle emissions and enhances fuel economy, making it a vital component in advancing sustainable vehicle innovations.
Kinetic Energy Recovery Techniques Explained
Understanding kinetic energy recovery is essential for grasping modern innovations in vehicle technology. It involves capturing and storing kinetic energy that's generated during motion, preventing its loss as heat. By reusing this energy, vehicles enhance efficiency and performance. Here, we explore various techniques used to achieve kinetic energy recovery.
Mechanical Recovery Techniques
Mechanical recovery techniques involve capturing kinetic energy through mechanical means. This is achieved using components such as flywheels and springs, which store energy as rotational or potential energy. These techniques are effective for providing immediate bursts of power.One common approach is the use of flywheel systems where energy is stored as rotational energy. The flywheel spins rapidly and can release stored energy quickly, aiding vehicle acceleration.
Imagine a vehicle using a flywheel system. When the vehicle decelerates, some of the kinetic energy is converted to rotational energy in the flywheel. The equation describing the energy stored in a flywheel is:\[E = \frac{1}{2} I \omega^2\]where \(E\) is the energy stored, \(I\) is the moment of inertia, and \(\omega\) is the angular velocity. This formula helps determine the energy content based on the flywheel's physical characteristics.
Another fascinating mechanical recovery technique uses springs to harness kinetic energy. Found mainly in compact applications, these systems compress a strong spring during braking, storing potential energy. Upon release, this energy assists in propelling the vehicle. Though simple, engineering durable, long-lasting springs capable of holding significant energy is challenging. Springs are less common in large, high-speed vehicles due to their limited energy storage capacity.
Electrical Recovery Techniques
Electrical recovery techniques primarily involve converting kinetic energy into electrical energy, stored in batteries or capacitors. These systems are inherent to hybrid and electric vehicles, enabling energy storage during braking for later use in acceleration or auxiliary power demands.
Battery-based KERS: A system converting kinetic energy into electrical energy, stored in batteries. It is integral to the functionality of hybrid and electric vehicles by improving energy efficiency and extending range.
Consider a battery-based electrical recovery system on an electric vehicle. When the car brakes, kinetic energy converted to electricity is stored in the battery. Later, this energy aids the vehicle's propulsion. For a detailed understanding, the stored energy \(E\) in a capacitor, used similarly to batteries, can be described by:\[E = \frac{1}{2} C V^2\]where \(E\) is the energy stored, \(C\) is the capacitance, and \(V\) is the voltage. Here, the capacitor provides short bursts of energy efficiently.
Electrical recovery systems not only improve vehicle energy efficiency but also contribute to lower emissions and extend driving ranges.
Kinetic Energy Recovery Mechanism Basics
Understanding kinetic energy recovery is crucial for grasping advancements in modern vehicle technology. It involves capturing energy generated during motion and storing it for future use, which enhances vehicle efficiency.
Mechanical vs. Electrical Recovery Techniques
Two primary methods for kinetic energy recovery exist: mechanical and electrical techniques.
- Mechanical Systems: Use devices like flywheels and springs to store and release energy as mechanical motion.
- Electrical Systems: Convert kinetic energy into electrical energy, storing it in batteries or capacitors for later use.
Kinetic Energy Recovery System (KERS): A device or set of devices that convert lost kinetic energy into a storable form, which is then used to enhance vehicle efficiency or performance.
Consider a hybrid vehicle utilizing a battery-based KERS during deceleration. The kinetic energy, converted to electricity, charges the battery. This system benefits fuel efficiency by using stored energy to aid propulsion. Mathematically, kinetic energy \(E_k\) is given by:\[E_k = \frac{1}{2} m v^2\]where \(m\) is the vehicle's mass and \(v\) its velocity. This represents the energy potential recaptured by KERS.
Exploring flywheel mechanisms further, these systems rely on the flywheel's rotational inertia. As the vehicle decelerates, kinetic energy spins the flywheel, storing energy efficiently.The effectiveness of flywheels comes from their quick energy transfer capability, governed by:\[E = \frac{1}{2} I \omega^2\]where \(I\) is the moment of inertia and \(\omega\) the angular velocity. Flywheels are optimal for scenarios needing rapid energy discharge but require high-precision engineering to manage high rotational speeds safely.
Flywheel systems excel in applications requiring quick bursts of power, such as in motorsport and performance cars.
Advantages of Kinetic Energy Recovery
Kinetic energy recovery systems (KERS) provide significant advantages in modern vehicles by optimizing the use of energy generated during motion. These systems not only enhance a vehicle's efficiency and performance but also contribute positively to environmental conservation.
Efficiency and Performance Enhancement
One of the core benefits of utilizing KERS in vehicles is the substantial boost in efficiency and performance. By recuperating energy that would otherwise be lost during braking, vehicles can achieve improved fuel economy and power output.For instance:
- Improved Fuel Efficiency: By using stored energy, less fuel is required, leading to longer distances traveled per liter of fuel.
- Enhanced Performance: The extra power from stored energy can assist in acceleration, offering better performance, especially in sports and high-end vehicles.
Efficiency Enhancement Formula: The efficiency gained from KERS can be mathematically represented by the formula:\[ \text{Efficiency} = \frac{\text{Energy Recovered}}{\text{Total Energy Output}} \times 100\]
Imagine a hybrid car with a KERS that recovers 30% of the energy usually wasted in braking. If the total energy output of the vehicle is 1000 MJ, the energy recovered would be calculated as:\[ \text{Energy Recovered} = 0.3 \times 1000 = 300 \text{ MJ} \]Thus, the efficiency enhancement would be:\[ \text{Efficiency} = \frac{300}{1000} \times 100 = 30\text{%}\]
A deeper dive into KERS reveals the complexities of integrating these systems into existing vehicle architectures. The challenge lies in balancing weight, cost, and effectiveness.Weight considerations are critical; heavier systems may negate potential performance benefits. Technological advancements, such as lightweight materials and compact designs, are making KERS more viable even for consumer vehicles.Additionally, electronic control systems are pivotal in managing energy flows efficiently to maximize recovery and assist functions. Engineers often use a combination of simulations and prototype testing to optimize KERS configurations. These simulations utilize equations accounting for loss factors, regenerative efficiency, and state-of-charge limits to refine system design.
Environmental Benefits
KERS are a vital component in reducing the environmental impact of vehicles. By promoting energy efficiency, these systems decrease emissions and reliance on fossil fuels, making vehicles more eco-friendly.The key environmental benefits include:
- Reduced Emissions: With better fuel efficiency, vehicles emit less CO2, helping to lower their carbon footprint.
- Decreased Energy Use: Vehicles consume less fuel, directly reducing oil dependency and conserving natural resources.
By improving vehicle efficiency and lowering emissions, KERS not only aid in combating climate change but also reduce operational costs in the long term.
Applications of Kinetic Energy Recovery in Automotive Engineering
Kinetic energy recovery systems (KERS) are increasingly being integrated into modern automotive engineering to enhance vehicle efficiency and performance. These systems capture energy that would otherwise be lost during vehicle operation, making it available for reuse and contributing to more sustainable automotive solutions.
Integration in Modern Vehicles
The integration of kinetic energy recovery systems in modern vehicles is a dynamic field with several applications, each contributing to efficiency and performance.
- Hybrid Vehicles: Use KERS to assist combustion engines, improving fuel efficiency and reducing emissions.
- Electric Vehicles (EVs): Employ KERS to extend battery life and range by recuperating energy during deceleration.
- Formula 1 Cars: Implement advanced KERS to provide a competitive edge through strategic energy boosts.
- Public Transport: More buses and trains are adopting these systems to cut down on operational costs and environmental impact.
In a hybrid vehicle equipped with KERS, when the driver brakes, kinetic energy is converted into electrical energy and stored in batteries. This stored energy is later used to power the vehicle, reducing the need for fuel consumption.Mathematically, the energy stored \(E\) in a battery can be represented as:\[E = \frac{1}{2} C V^2\]where \(C\) is the capacitance of the battery and \(V\) is the voltage. This formula helps optimize the battery's size and capacity based on the vehicle's energy needs.
By integrating KERS into public transport systems like buses and trains, cities can significantly cut down on fuel usage and emissions. These systems provide an efficient solution for urban areas by recovering large amounts of energy during frequent stops, typical in city driving.To make the integration cost-effective, transport agencies often collaborate with engineers to retrofit existing vehicles with modern KERS, using data analytics to fine-tune these systems for maximum efficiency. The operational data helps in adjusting parameters like braking patterns and energy discharge rates. Ultimately, KERS can lead to reduced operational costs and enhanced energy management in public transport.
Future of Kinetic Energy Recovery in Automobiles
The future of kinetic energy recovery in automobiles is poised for remarkable growth, driven by ongoing innovations and the global push towards sustainable technology. Vehicle manufacturers are continuously exploring ways to advance and expand the applications of KERS.Upcoming trends include:
- Increased Adoption in Consumer Cars: With cost reductions and technological advancements, KERS will become standard in more everyday vehicles.
- Integration with Advanced Electric Grids: Vehicles equipped with KERS can potentially feed recovered energy back into the grid, helping manage supply and demand.
- Smarter Control Systems: Enhancements in digital control systems will optimize energy recovery and utilization with precision.
KERS could soon integrate with smart city infrastructures, leveraging IoT technologies to lead a new era of intelligent transportation networks.
kinetic energy recovery - Key takeaways
- Kinetic Energy Recovery Definition: The process of capturing and storing energy generated during motion, typically lost during actions like braking, to enhance vehicle efficiency.
- Kinetic Energy Recovery System (KERS): Devices or mechanisms that convert kinetic energy into other forms, such as electrical or mechanical, for efficient use in vehicles.
- Kinetic Energy Recovery Techniques: Includes mechanical methods like flywheel systems and springs, as well as electrical systems using batteries or capacitors, to store energy.
- Kinetic Energy Recovery Mechanism: The working principle behind KERS, involving the conversion of kinetic energy into storable forms for improved vehicle efficiency.
- Advantages of Kinetic Energy Recovery: Improved fuel efficiency, reduced emissions, and enhanced vehicle performance by capturing and reusing energy.
- Applications of Kinetic Energy Recovery: Widely used in hybrid and electric vehicles, Formula 1 cars, and increasingly in public transport to reduce energy consumption and emissions.
Learn faster with the 24 flashcards about kinetic energy recovery
Sign up for free to gain access to all our flashcards.
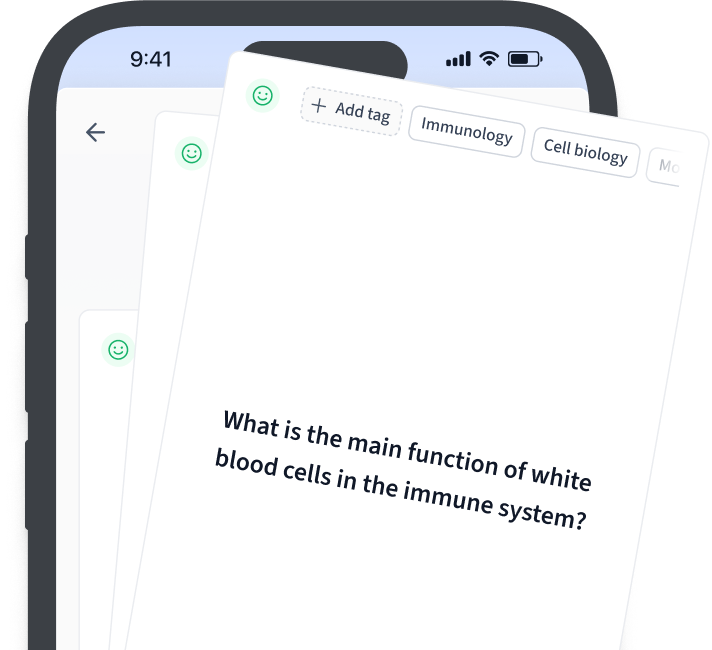
Frequently Asked Questions about kinetic energy recovery
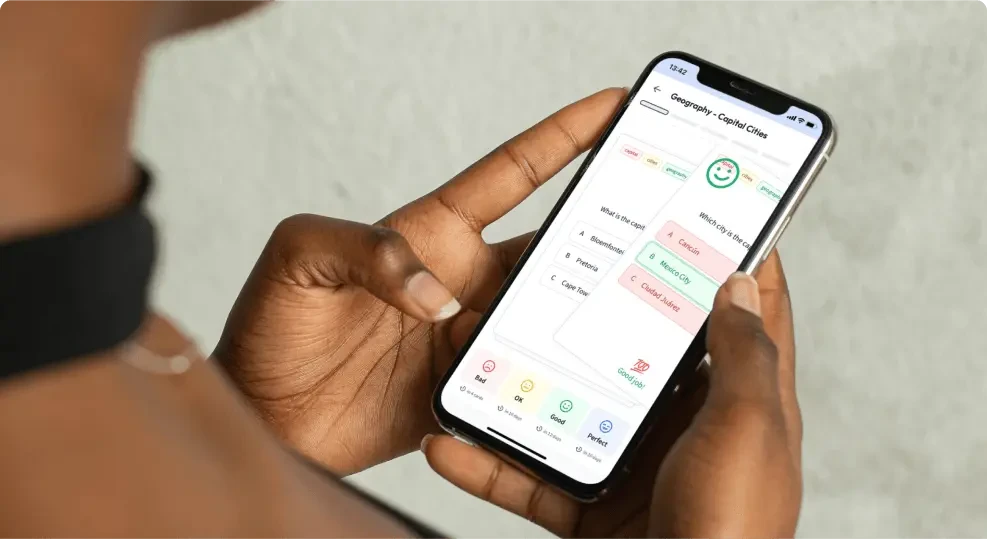
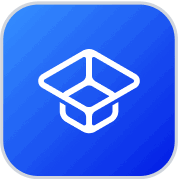
About StudySmarter
StudySmarter is a globally recognized educational technology company, offering a holistic learning platform designed for students of all ages and educational levels. Our platform provides learning support for a wide range of subjects, including STEM, Social Sciences, and Languages and also helps students to successfully master various tests and exams worldwide, such as GCSE, A Level, SAT, ACT, Abitur, and more. We offer an extensive library of learning materials, including interactive flashcards, comprehensive textbook solutions, and detailed explanations. The cutting-edge technology and tools we provide help students create their own learning materials. StudySmarter’s content is not only expert-verified but also regularly updated to ensure accuracy and relevance.
Learn more